8.4 Glycolysis
Glycolysis is a metabolic pathway that uses carbohydrate in the form of glucose or glycogen as fuel to generate ATP anaerobically. Glycolysis begins with the six-carbon, ring-shaped structure of a single glucose molecule and ends with two molecules of a three-carbon sugar called pyruvate. As you can see in Figure 8.9, glycolysis consists of two distinct phases. In the first part of the glycolysis pathway, two ATP are required so that the six-carbon sugar molecule can be split evenly into two three-carbon pyruvate molecules. In the second part of glycolysis, four ATP and two NADH’s are produced. After subtracting the two ATP required upfront, the net yield of glycolysis when starting with glucose is 2 ATP, 2 NADH, and 2 pyruvate. Muscle glycogen enters glycolysis part way through the first phase of glycolysis and only one ATP is required upfront. This means that when starting with muscle glycogen, the net yield of glycolysis is 3 ATP, 2 NADH, and 2 pyruvate. This is why exercising muscles preferentially use muscle glycogen instead of blood glucose when glycogen is available.
Figure 8.9 Glycolysis Energy System
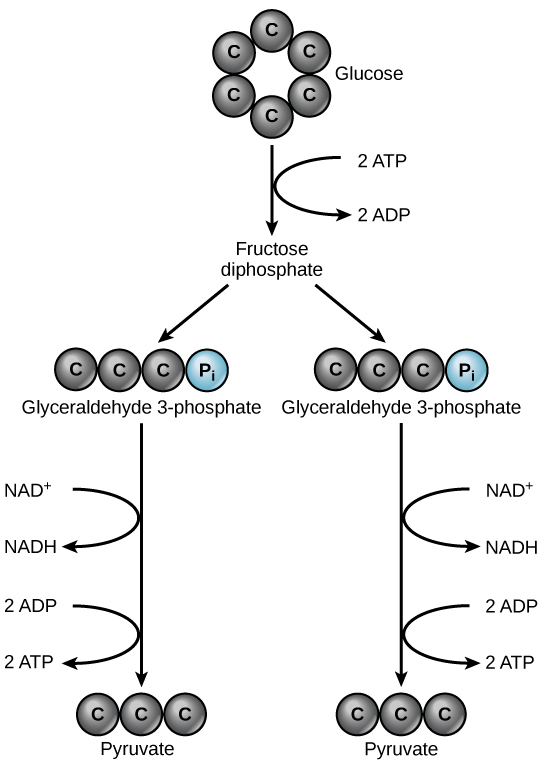
Because it is more complex than the ATP–CP system, glycolysis is a slightly slower system. It is still, however, a relatively fast-acting energy system and is the predominating energy system for moderate-to-high intensity, speed types of activities. During high intensity exercise, oxygen availability is limited in the exercising muscles; therefore, ATP must be produced anaerobically. At the beginning of high intensity exercise, the ATP-CP system predominates for the first 5-10 seconds and by the time CP stores become depleted, glycolysis is functioning at full capacity and will become the predominant energy system for 1-2 minutes. We can say that glycolysis is the predominant energy system for high intensity speed activities lasting up to 2 minutes. Some examples of speed activities include:
- Slightly longer sprints (200m, 400m, or 800m sprints in track)
- Most swimming races (50-200m)
- Repeated intervals (during the work portion)
- Sprinting down the field or court in soccer or basketball
- Weight training (sets of 10-15 repetitions)
One of the end products of glycolysis is pyruvate. This pyruvate can be metabolized under both aerobic and anaerobic conditions. During moderate-high to high intensity exercise when oxygen availability is limited, most of the pyruvate is converted into lactate anaerobically. During more aerobic conditions (low-to-moderate–intensity exercise), pyruvate is oxidized to acetyl CoA which then enters the aerobic system. This conversion of pyruvate to acetyl CoA is the first point of carbohydrate metabolism that requires oxygen. Lactate is an important chemical intermediary during high-intensity exercise. If you refer back to Figure 8.9, you see that during the second half of glycolysis, the coenzyme NAD+ is required for one of the parallel chemical reactions in the second phase of glycolysis (NAD+ is the coenzyme form of the B vitamin, niacin). During glycolysis, two molecules of NAD+ are reduced to NADH. However, the amounts of NAD+ in the cell are limited, and therefore NADH must be oxidized back to NAD+. Why must NADH be oxidized back to NAD+? Without NAD+, glycolysis could not continue and ATP production would grind to a halt. When adequate amounts of oxygen are present in the cell, NADH continues on to aerobic metabolism where it is converted back into NAD+ in the electron transport chain. During high intensity exercise when adequate oxygen is not available, lactate plays an important role in the oxidation (or regeneration) of NADH to NAD+ which allows glycolysis to continue to proceed at high intensities for a longer period of time.
Pyruvate + NADH + H+ → Lactate + NAD+
The lactate then diffuses out of the cell and the NAD+ is used to continue glycolysis. It is in this manner that the cell continues to produce energy under anaerobic conditions.
The conversion of pyruvate to lactate is a two-way reaction; pyruvate can be converted to lactate but when lactate concentration is high, it can be converted back into pyruvate. As stated earlier, pyruvate can be converted to acetyl CoA and oxidized under more aerobic conditions. It is by this mechanism that lactate is metabolized during exercise. When lactate accumulates in cells during exercise, the increase in concentration causes it to diffuse out of the cell and move into the bloodstream or into neighboring muscle cells. Once in the blood, the lactate can be taken up by more highly aerobic tissues (heart, liver, and kidney), converted back into pyruvate, and used to produce ATP in the aerobic system. When lactate diffuses into neighboring aerobic (slow twitch) muscle cells it can also be converted back into pyruvate and used for ATP production in aerobic metabolism. The third fate of lactate is known as the Cori Cycle. The Cori Cycle, as seen in Figure 8.10, is a metabolic pathway in which lactate from the blood is taken up by the liver and converted back into glucose. This glucose is then released back into the blood and can be used to help maintain blood glucose levels. The Cori Cycle is an example of gluconeogenesis and does require oxygen. While this may seem like a good solution to regenerate glucose, the Cori Cycle requires 6 ATP to convert lactate into glucose. Because glycolysis only produces 2 ATP, the Cori Cycle will lead to an energy deficit over time and cannot be sustained indefinitely. Regardless of its fate, lactate, which was once considered “metabolic waste,” is a critical part of energy production during intense exercise.
Figure 8.10 Cori Cycle
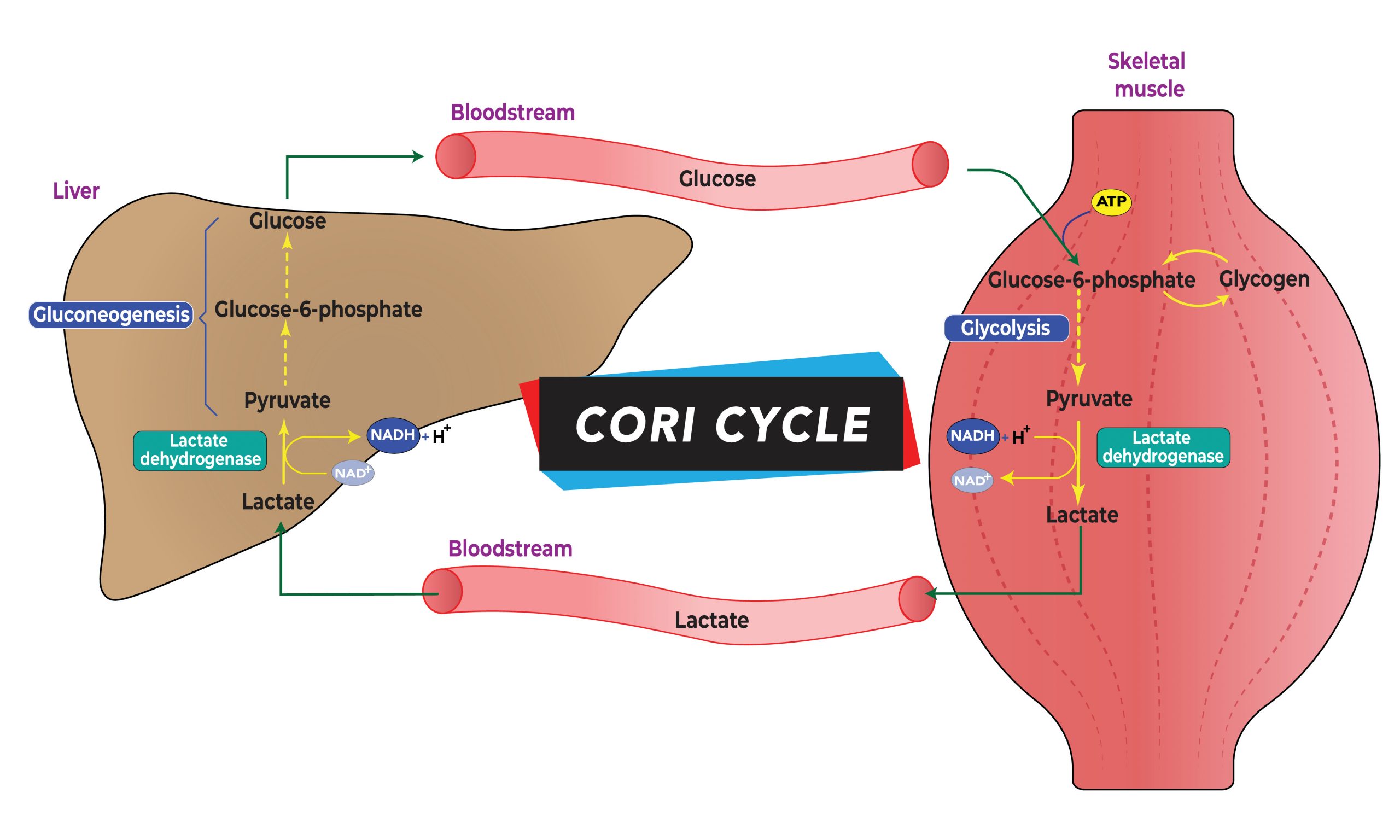
Media Attributions
- Glycolysis © OpenStax is licensed under a CC BY (Attribution) license
- Cori Cycle © Adobe Stock is licensed under a CC BY (Attribution) license