Mesenteric Vasculature
Nicole M. Hindman
Christoph U. Herborn
R. Brooke Jeffrey
Introduction: Spectrum and Prevalence of Disease
Mesenteric Arteries
A broad spectrum of disorders may affect the mesenteric arteries, including such diverse conditions as collagen vascular disease, vasculitis, fibromuscular dysplasia, trauma, and neoplastic encasement. However, by far, the most common pathologic process is occlusive disease caused by atherosclerosis. The vast majority of patients with atherosclerotic disease of the mesenteric arteries are elderly and asymptomatic (1,2). The celiac axis (CA), superior mesenteric artery (SMA), and inferior mesenteric artery (IMA) comprise the three major branch vessels arising off the aorta that supply the abdominal gastrointestinal (GI) viscera.
Atherosclerotic occlusive disease of the mesenteric arteries carries the potential risk for life-threatening bowel ischemia and infarction. Yet, despite the widespread prevalence of mesenteric atherosclerosis in the adult population,
the actual incidence of clinically significant intestinal ischemia is remarkably low in clinical practice. Surgery for acute mesenteric ischemia (AMI) represents <1% of all laparotomies (3).
the actual incidence of clinically significant intestinal ischemia is remarkably low in clinical practice. Surgery for acute mesenteric ischemia (AMI) represents <1% of all laparotomies (3).
The low incidence of clinically significant intestinal ischemia is undoubtedly due to the ability of the mesenteric arterial circulation to form extensive collateral pathways, given the slow progressive nature of the disease. The one exception to this is acute occlusion of the SMA due to embolic disease, usually from a cardiac source. This is an important etiology of acute intestinal ischemia, representing 50% of all cases (4).
The development of chronic mesenteric ischemia typically requires disease progression in two of the three major branch vessels. Chronic mesenteric ischemia (CMI) is an unusual but important cause of abdominal pain. Although this condition accounts for only 5% of all intestinal ischemic events, it can have significant morbidity (5).
All other disease processes involving the mesenteric arteries—including arteritis, collagen vascular disease, fibromuscular dysplasia, and intestinal dissection—are clinically exceedingly rare.
Portal Veins
The hemodynamic effect of cirrhosis and portal hypertension is far and away the most common disease process to affect the portal venous system. Anatomic changes accompany the prolonged raised pressure within the intra- and extrahepatic portal veins, including dilation of the main portal vein and the development of numerous venous collaterals, most notably esophageal varices. In the United States for the year 2003, cirrhosis was the twelfth leading cause of death, representing 1.1% of all fatalities (6,7). Although alcoholism still represents an important cause of cirrhosis worldwide, its incidence in the United States is currently declining (8). The prevalence of portal hypertension now most closely parallels the development of cirrhosis due to the worldwide epidemic of viral hepatitis, particularly in Asia. It is estimated that chronic hepatitis B infection occurs in 0.5% of the population of the United States and Europe but infects approximately 10% of the population of Asia and nearly 350 million people worldwide (9). Hepatitis C is estimated to infect 3% of the global population, or approximately 170 million individuals (9,10). The incidence of hepatitis C in the United States is approximately 3.8 million (10). Even if a very small percentage of individuals go on to develop clinically significant cirrhosis from hepatitis B and C, the dimension of the problem is enormous, given the worldwide prevalence of infection.
The most clinically significant effects of cirrhosis and portal hypertension are the development of esophageal varices and the risk of acute life-threatening variceal hemorrhage. Approximately half of all patients with cirrhosis will develop esophageal varices, and one third of these patients will have a clinically significant GI hemorrhage from these varices within 2 years of the diagnosis (11). Compared with other causes of upper GI bleeding, hemorrhage from esophageal varices carries an unusually high mortality rate of 30% to 60%, thus underscoring the clinical severity of this entity (12,13,14).
The second most clinically important disease process to involve the portal veins is thrombosis. Thrombus formation within the portal or superior mesenteric veins may be classified as acute or chronic and either partially or totally occlusive. Patients may be entirely asymptomatic if the thrombus is nonobstructive or may have symptoms of intestinal ischemia if there is acute total occlusion of the superior mesenteric vein (SMV). Venous thrombosis accounts for 15% to 20% of all cases of bowel infarction (15). Primary or idiopathic thrombosis of the portal venous system is actually quite rare, and the vast majority of cases are secondary to identifiable risk factors such as hypercoagulable states, pancreatitis, abdominal surgery, trauma, portal hypertension, and bowel obstruction from volvulus or intussusception (15).
Global View of Unique Aspects and Considerations of Imaging of This Organ System
The early diagnosis of acute occlusive mesenteric ischemia is essential to minimize the associated high mortality rates. A liberal use of conventional, catheter-based angiography is thus frequently advocated. Depending on resource availability, some patients presenting acutely can be well served with multidetector computed tomography (MDCT) or computed tomographic angiography (CTA).
CMI is a condition that can be clinically elusive and greatly benefits from the physiologic assessments available with duplex sonography and phase contrast (PC) MRA, comparing velocity and flow values prior to and after a prescribed caloric intake (16,17,18,19).
For cases that require a rapid evaluation of the vasculature and parenchyma, such as trauma, computed tomography (CT)/CTA is the preferred technique (20,21,22), whereas in an elective assessment, such as for malignancy or portal hypertension, both CT/CTA and magnetic resonance (MR)/magnetic resonance angiography (MRA) are most effective (20).
Venous imaging is the clear domain of CTA/CTV (CT venography) and MRA/MRV (MR venography), as delineation of the relevant vasculature is most reliable with these techniques (15).
What Do Computed Tomography and Magnetic Resonance Bring over Other Imaging and Nonimaging Diagnostic Testing?
Digital Subtraction Angiography
When evaluating patients suspected of having a mesenteric vascular disease, it is the clinical presentation of the patient that guides the choice of a specific diagnostic modality. For patients presenting with AMI, digital subtraction angiography (DSA) is typically the diagnostic modality of choice offering the opportunity for diagnosis and treatment of many conditions. Depending on the particular diagnosis, vasodilators (23), thrombolytics (24), and angioplasty (+/- stent) (25) can be applied to achieve revascularization and restoration of blood flow at the time of diagnosis.
DSA is an invasive and expensive technique that is capable of providing high-quality images of the mesenteric vasculature but is associated with a small but finite morbidity, including arterial dissection and thrombosis (26,27). In addition to abdominal aortography, selective injections into the celiac and SMA are routinely required for comprehensive evaluation. In selected patients, this results in a considerable radiation dose and contrast burden. Arteriography was used in the past to diagnosis both embolic disease to the SMA (cutoff sign) and arterial stenoses in CMI (Fig. 19-1). With DSA, information about blood flow can only be inferred. In addition, global assessment of intra-abdominal processes is relatively poor with angiography, with only limited information about the bowel wall, mesentery, peritoneal cavity, and solid viscera.
Duplex Sonography
Duplex sonography is a noninvasive means to provide quantitative data about the flow velocity in the larger mesenteric vessels of interest. This has been of value in CMI, but its success depends on operator expertise. A combination of increased peak systolic velocity (greater than 275 cm/second in the SMA) and increased end diastolic velocity correlates with a greater than 70% stenosis with sensitivities in the 80% to 90% range (28,29,30). This may be useful information to screen for CMI, but it does not provide sufficient anatomic information to direct therapy (either stent placement or surgical reconstruction). A combination of color flow imaging with duplex sonography and ultrasound (US) contrast agents may be a rapid and inexpensive screening technique for patients with CMI (31,32).
Pre- and postprandial studies can provide physiologic information regarding the ability to mount a normal postprandial hyperemia (33). Direct imaging of the major branch vessels can be limited by body habitus and bowel gas and may not benefit greatly from the use of US contrast agents (34).
Color Doppler sonography, however, may be highly effective to screen patients for suspected portal vein thrombosis (31,32). Yet, sonography often fails to visualize the entire length of the SMV, and, therefore, in patients with possible SMV thrombosis, CT and MR are the imaging techniques of choice.
Computed Tomographic Angiography
Both contrast-enhanced (CE) CT and magnetic resonance imaging (MRI) may provide exquisite anatomic displays of the mesenteric arteries and portal venous system. The acuity of patient symptoms, therefore, often dictates the choice of imaging modality. In patients with sudden onset of severe abdominal pain, AMI is one of many important diagnostic considerations, including such diverse entities as perforated duodenal ulcer, pancreatitis, bowel obstruction, peritonitis, and cholecystitis. In general, CT is the preferred diagnostic modality in acutely ill patients. CT can visualize not only the mesenteric arterial circulation and portal venous system but can also detect important secondary findings involving the liver, bowel wall, and mesentery—such as pneumotosis, portal venous gas, bowel wall thickening, and other secondary findings of intestinal ischemia—thus providing comprehensive information for diagnosis and treatment planning.
The net iodinated contrast media load administered to the patient and its attendant risks must be considered in the context of possible follow-up with catheter-based interventional techniques.
Magnetic Resonance Angiography
Although not frequently used in the emergent setting (due to instability of patients, remote location of MRI scanners, and relatively long imaging times compared with CT), MRA provides excellent contrast resolution of the mesenteric vasculature, bowel, and mesentery. Acquisition of images utilizing three-dimensional (3D) volumetric sequences allows for reconstruction in multiple planes, facilitating the visualization of subtle vascular abnormalities. Despite advances in limiting motion of the bowel, the resolution of the distal vasculature is still limited, but application of advanced techniques combined with pharmacologic preparation (e.g., glucagon, hyoscyamine sulfate) offers exciting future possibilities in this arena.
In patients with suspected abdominal angina and CMI, CT and MRI often provide complementary diagnostic information. CE MDCT and gadolinium-enhanced MRI in combination with image displays such as volume
rendering (VR), maximum-intensity projection (MIP), and curved planar reformations (CPRs) provide excellent anatomic displays of the mesenteric arterial and portal venous systems and resultant collaterals forming in response to chronic occlusion (2,35,36,37,38,39,40,41,42,43,44).
rendering (VR), maximum-intensity projection (MIP), and curved planar reformations (CPRs) provide excellent anatomic displays of the mesenteric arterial and portal venous systems and resultant collaterals forming in response to chronic occlusion (2,35,36,37,38,39,40,41,42,43,44).
In addition to this high-resolution anatomic information, MRI has the additional advantage of providing quantitative functional information about mesenteric arterial and venous blood flow. Using PC angiography, quantitative flow information can be provided that may be useful in documenting CMI (39). In addition, novel MRI techniques have been developed to measure postprandial oxygen extraction in the SMV that may provide physiologic confirmation in conjunction with anatomically demonstrated mesenteric arterial stenoses (36,37,38,41,43,44).
Imaging Strategies
Computed Tomography
In order to evaluate both the mesenteric arteries and the portal venous system with CE MDCT, a biphasic scan is required. An initial high-resolution arterial study is performed during peak arterial enhancement following a rapid intravenous (IV) injection (4 to 5 mL/second) from an IV
power injection. This is followed by a delayed scan (60 to 70 seconds from the start of the injection) to acquire images during the portal venous phase to assess the portal venous system and solid abdominal viscera (42).
power injection. This is followed by a delayed scan (60 to 70 seconds from the start of the injection) to acquire images during the portal venous phase to assess the portal venous system and solid abdominal viscera (42).
The use of negative oral contrast agent represents a practical means of marking the bowel, facilitating the visualization of bowel wall enhancement, and the presentation of the vascular structures (45,46) (Fig. 19-2).
The initial patient preparation should involve only water (500 to 700 mL) as a negative oral contrast agent prior to scanning. Positive oral contrast agents will substantially interfere with reconstructions such as MIPs to delineate the mesenteric arteries and should always be avoided. In addition, water distention of the bowel lumen facilitates evaluation of focal or diffuse mural abnormalities and perfusion of the small bowel, stomach, and duodenum. As with all CTA techniques, newer multidetector scanners (either 16- or 64-detector row) offer the distinct advantage of thinner collimation and shorter scan times, and therefore shorter breath-holding requirements for patients. However, adequate imaging can be provided with a 4-detector row CT if the patient is able to hold their breath for at least 25 seconds. Because of the scan acquisition in the craniocaudal direction, shallow breathing can be performed during the latter part of the scan, when images are obtained through the lower abdomen, without significant motion artifact.
Careful attention should be given to the contrast bolus administration and timing of image acquisition for optimal arterial opacification. Although in thinner patients with low body mass index, as little as 80 mL of contrast may provide adequate enhancement, in general, approximately 100 to 120 mL of warm iodinated contrast is administered via a 20- or 18-gauge IV cannula placed in an antecubital vein. This is to accommodate a power injection of 4 to 5 mL/second, which is essential for adequate arterial opacification. A saline flush of 40 to 50 mL is quite valuable to ensure complete delivery of all the iodinated contrast. Bolus tracking to determine peak arterial enhancement is the most efficient method of determining the appropriateness of scan delay. This is generally performed by obtaining a series of low-dose scans at the level of the upper abdominal aorta and placing a region-of-interest cursor within the aorta. Once a triggering threshold reaches 150 Hounsfield units (HU) above the baseline noncontrast scans, the patient is instructed to perform a breath hold. Image acquisition is then obtained for delineation of the mesenteric arteries. The scanning region of interest for the arterial acquisition is from just above the CA to the symphysis pubis. Following a 60- to 70-second delay from the initial injection, another breath-hold acquisition is obtained of the entire upper abdomen and pelvis to delineate the portal veins and solid abdominal viscera. Scanning parameters for both a 4- and 16-detector row MDCT scanner will differ slightly. Typically, collimation is 1 to 1.25 mm for the 4-detector row scanner and 0.75 mm for the 16-detector row scanner. Venous phase scans should be obtained at 2.5- to 5.0-mm collimation.
To obtain the highest quality volumetric imaging with voxels, there should be at least a 30% to 50% overlap in reconstruction interval. This substantially improves z-axis resolution and the quality of multiplanar and 3D reformations. A variety of postprocessing techniques should be performed routinely for optimal mesenteric arterial imaging. This includes obtaining MIP and VR images, as well as CPRs of the celiac, and SMA (Fig. 19-3).
CPRs are particularly useful to delineate individual vessels such as the SMA and celiac and may be invaluable in the presence of metallic stents to determine precise positioning of the stent and intraluminal thrombus formation (Fig. 19-4). In patients with extensive calcification within the abdominal aorta and splanchnic vessels, CPRs may
more accurately delineate the assessment of arterial stenoses. The patient with extensive calcification may provide unique challenges for MIP or VR; therefore, CPR should be done routinely to precisely assess the degree of luminal narrowing. Similarly, in patients with suspected tumor encasement of the mesenteric vessels, CPRs are quite useful to assess perivascular tumor extension (33,34,35,47,48,49).
more accurately delineate the assessment of arterial stenoses. The patient with extensive calcification may provide unique challenges for MIP or VR; therefore, CPR should be done routinely to precisely assess the degree of luminal narrowing. Similarly, in patients with suspected tumor encasement of the mesenteric vessels, CPRs are quite useful to assess perivascular tumor extension (33,34,35,47,48,49).
Magnetic Resonance
MRI has been successfully established as an outstanding diagnostic tool for the morphological and functional evaluation of the mesenteric and portal venous system since the early 1990s. Besides offering excellent analysis of the parenchymal and hollow organs in the abdomen with unsurpassed soft tissue contrast, 3D CE MRA also permits accurate assessment of the abdominal aorta and particularly the splanchnic arteries, that is, the celiac trunk and its branches as well as the SMA and the IMA (40,50,51,52,53,54,55).
Recent improvements in gradient capabilities and new parallel imaging techniques allow for rapid image acquisition with submillimeter spatial resolution acquired in a single short breath hold (56). Therefore, it is anticipated that there will be a shift toward utilizing MRA examinations for evaluation of the visceral arteries and portal vessels for many clinical indications, including vascular diseases, functional disorders, and neoplasms (57).
The gains in gradient power capabilities have laid foundation for a variety of new fast MRI techniques for the abdominal vasculature. In patients suspected of having CMI, patient preparation is not as important for routine MRA as it is for catheter-based arteriography, CT, or US. Patients do not need to fast prior to the MR examination nor does intraluminal gas in the small bowel impair image quality. Application of glucagon (0.5 to 1 mg IV or intramuscularly [IM]) or scopolamine (40 mg IV) prior to the MR examination has been proposed as a means for reducing bowel motion and augmenting splanchnic flow. However, with a fast scanner capable of high-resolution imaging during a mere breath hold, arresting bowel motion is not deemed mandatory. Alternatively, a high-caloric meal prior to the examination can transiently increase splanchnic flow, thereby enhancing visibility of the smaller branch vessels (58). Patients with chronic abdominal symptoms, suspected of AMI, should be considered surgical emergencies. MRA should only be performed in patients presenting with an acute abdomen under unusual circumstances and only if adequate monitoring is available. An abdominal surgeon should be nearby in case the patient decompensates and requires immediate surgical exploration.
Patients suspected of CMI are often very thin and thus well suited to MRI with surface array coils, such as a body array or torso phased array coils. Large patients may be easier to image by using the body coil. The patient is placed in the supine position. The arms may be in a comfortable position by the patient’s side during the initial localizer sequence and throughout the 3D CE MRA acquisition in the coronal plane. However, if the patient is able to elevate the arms
over the head throughout the examination, this might help to minimize aliasing.
over the head throughout the examination, this might help to minimize aliasing.
Time-of-flight Magnetic Resonance Angiography
Time-of-flight (TOF) MRA enables high-contrast views of the abdominal arteries with the use of presaturation bands (53,59). However, being one of the oldest techniques used, TOF MRA has not been established in current clinical practice for the evaluation of the mesenteric arteries due to several limitations. Images have to be acquired orthogonal to flow, thereby complicating the depiction of the ostia of both the celiac trunk and the SMA because the flow within the aorta is at right angles to that in the proximal part of both vessels. This often results in artifacts at the vessel origins where most of the pathology in patients with CMI is located. Reflecting the anatomic predisposition of the celiac artery and the SMA, it is impossible to optimize a single TOF-sequence for the depiction of the two arteries. In addition, misregistration artifacts may arise from the need to acquire images during suspended respiration in patients with limited breath-holding capabilities.
Phase Contrast Magnetic Resonance Angiography
PC MRA techniques require relatively long imaging times, as at least two data sets are acquired by using flow-encoding gradients of opposite polarity, and at least three measurements in the orthogonal planes are needed for detection of flow. However, PC MRA can be used to assess the mesenteric vasculature by using either 3D (60) or two-dimensional (2D) approaches (16,36,61,62); the aforementioned technique allows for image acquisition in any desired plane (60).
Wasser and associates (60) reported their experience with a systolically gated PC MRA technique in patients with suspected mesenteric ischemia. Only 66% of stenoses seen on catheter-based arteriography could be visualized by MRA, and false-positive results were encountered. The limitations include problems with phase ghosting, motion artifacts, and uncertainty as to choice of appropriate velocity-encoding (VENC) gradient value.
The long scan times of PC MRA predisposed the study to respiratory motion artifacts. In addition, ghost artifacts caused by diminished and reversed flow during systole can severely hamper image quality. Cardiac triggering can counter the latter limitation, albeit with a further increase in scan time. Thus, respiratory motion artifacts remain a major problem with PC MRA. Combinations of cardiac triggering and respiratory compensation strategies can be quite successful.
Cardiac-gated 2D PC MRA techniques have been implemented for the mesenteric vasculature to provide functional information. This approach segments the phase data acquired at different time points throughout the cardiac cycle and thereby permits quantitative flow measurements, including velocities and volumes. However, accurate contrast is poorly achieved due to flow and saturation effects within visceral vessels that course in different directions, bowel peristalsis, and motion artifacts owing to long acquisition times.
Contrast-enhanced Magnetic Resonance Angiography
3D CE MRA does not depend on flow effects of unsaturated spins and flow phenomena and thus overcomes the saturation problems encountered with the above-mentioned techniques. CE MRA relies on heavily T1-weighted gradient echo sequences with very short repetition times and echo times, which produce high contrast between vessels and the surrounding tissue. Short repetition times result in fast acquisition times and, therefore, fewer motion artifacts and greater coverage of the area of interest. Inherent complexity of CE MRA leads to a trade-off between spatial resolution, volume coverage, and acquisition time for each respective examination. Subtraction of pre- and postcontrast examinations, as is commonly used in MRA imaging of the vasculature of the extremities, does not always improve image quality owing to bowel motion between the data acquisitions. However, the introduction of CE MRA enables the generation of high-quality images of the mesenteric vasculature (54,63,64).
3D imaging in the coronal acquisition plane permits evaluation of the aorta, splanchnic arteries, and portal vein in a single examination. A slice partition thickness between 3 and 5 mm is acceptable if zero padding of the k-space is available for interpolation. In the absence of an interpolation algorithm, the slice thickness should be kept to less than 3 mm in order to depict smaller branches of the mesenteric arteries. To determine stenotic disease of the proximal portions of the SMA and IMA, imaging in the sagittal plane leads to improved image quality. Aliasing is less of a concern when scanning in the sagittal plane, and it is possible to prescribe an aggressive rectangular field-of-view volume with a high-resolution acquisition matrix (Fig. 19-5).
When using magnets with slower gradients, it might be advantageous to acquire data in the sagittal plane with fewer sections required to cover the aorta, celiac SMA, and IMA. A relatively short breath-hold scan performed in the sagittal plane is preferable to a comparably longer coronal acquisition that may be hampered by respiratory artifacts. Imaging parameters should be individually adjusted to
allow for data acquisition within reasonable breath holds for the patient being studied. Axial imaging may be useful if the primary goal is evaluation of the hepatic arteries, hepatic parenchyma, and portal venous system. One difficulty with the axial orientation is aliasing in the slice direction, which tends to be severe with the extremely short radio frequency (RF) pulses typically used in 3D CE MRA. To minimize aliasing, coils with S-I dimensions only slightly larger than the S-I dimension of the imaging volume tend to provide better image quality. Fat saturation or chemically selective fat inversion pulses might also be applicable. This will help to minimize unwanted signal from pericardial and abdominal fat wrapping onto the bottom and top of the image volume. A 3D CE MRA image data set should be collected before, during, and following completion of the IV contrast administration. Precontrast images should be checked to be sure that the imaging volume is positioned correctly.
allow for data acquisition within reasonable breath holds for the patient being studied. Axial imaging may be useful if the primary goal is evaluation of the hepatic arteries, hepatic parenchyma, and portal venous system. One difficulty with the axial orientation is aliasing in the slice direction, which tends to be severe with the extremely short radio frequency (RF) pulses typically used in 3D CE MRA. To minimize aliasing, coils with S-I dimensions only slightly larger than the S-I dimension of the imaging volume tend to provide better image quality. Fat saturation or chemically selective fat inversion pulses might also be applicable. This will help to minimize unwanted signal from pericardial and abdominal fat wrapping onto the bottom and top of the image volume. A 3D CE MRA image data set should be collected before, during, and following completion of the IV contrast administration. Precontrast images should be checked to be sure that the imaging volume is positioned correctly.
The use of 3D sequences structured to achieve excellent delineation of both the vasculature and soft tissues, such as volumetric interpolated breath-hold examination (VIBE; Siemens Medical Systems, Erlangen, Germany) and liver acquisition with volume acceleration (LAVA; General Electric, Giles, United Kingdom), is recommended (65).
Manual versus automated contrast material injection has been as much debated as the amount of gadolinium, the rate of injection, and the optimal synchronization of contrast material injection and data acquisition. A single dose of contrast material (i.e., 0.1 mmol/kg) is feasible for high-quality contrast enhancement of the mesenteric arteries, but many choose to use double-dose (0.2 mmol/kg) administrations. Above all, proper gadolinium bolus timing is essential for arterial phase acquisitions. This can easily be accomplished with automatic triggering (SmartPrep by General Electric, Waukesea, WI, or CARE Bolus by Siemens Medical Systems, Erlangen, Germany) or fluoroscopic triggering (Bolus Track by Philips Medical Systems, Best, Netherlands) or with a test bolus to the mid abdominal aorta (66,67,68) (see Chapter 2). After arterial phase imaging, a delayed image data set is useful for showing the portal venous and hepatic venous anatomy.
Arterial phase 3D CE MRA is best analyzed by first performing multiple overlapping thin-slice MIPs in the
coronal plane. Subsequently, reformations and subvolume MIPs can be reconstructed in perpendicular planes through each major abdominal aortic branch vessel, including the celiac trunk, SMA, and IMA. It might also be useful to assess the iliac arteries, especially internal iliac arteries, as they may represent an important collateral pathway in patients with CMI.
coronal plane. Subsequently, reformations and subvolume MIPs can be reconstructed in perpendicular planes through each major abdominal aortic branch vessel, including the celiac trunk, SMA, and IMA. It might also be useful to assess the iliac arteries, especially internal iliac arteries, as they may represent an important collateral pathway in patients with CMI.
By means of CE 3D MRA, an accurate morphologic assessment of the proximal mesenteric vessels can be accomplished. Meaney et al. (63) examined 65 patients with suspected mesenteric ischemia by using a 3D CE MRA technique. In all 14 patients with catheter-based angiographic correlation, all significant stenoses of the celiac artery and the SMA were identified. Twenty-eight of 30 arteries with correlation were correctly graded; therefore, despite disagreement between MRA and catheter-based arteriography in two patients, MRA had both sensitivity and specificity of 100% for diagnosis of mesenteric ischemia compared with catheter-based arteriography or surgery, and the specificity was 95%. The authors concluded that CE 3D MRA is accurate for the evaluation of the origins of the mesenteric and celiac arteries, although the image resolution is too low for a reliable assessment of the IMA. In addition, the delineation of the small mesenteric branch vessels is still hampered by the limited spatial resolution of MRA (52). Furthermore, the development of a strong collateral circulation can prevent major abdominal symptoms in patients with severe alterations of the mesenteric arteries (38,69,70).
Complementary Sequences
If the patient is capable of lying still in the magnet for a short while, additional T1- and T2-weighted turbo spin echo images covering the liver and upper abdomen may be acquired before and after contrast material injection to search for other relevant pathology that might account for the patient’s abdominal symptoms.
3D CE MRA provides a morphological analysis of the mesenteric arteries. The high incidence of visceral artery stenosis in the asymptomatic population makes it difficult to determine the clinical significance of a morphologic finding. For patients with mesenteric artery stenosis or an equivocal history of mesenteric ischemia, it may be difficult to predict whether correcting the mesenteric artery stenosis will alleviate symptoms. Functional MRI may complement 3D CE MRA in this regard. A cine PC sequence can assess blood flow in the SMV following caloric stimulation. Postprandial blood flow is increased within the SMV out of proportion to SMA blood flow with mesenteric ischemia (16,61). This effect is due to recruitment of collateral flow. By exploiting the known paramagnetic effect of deoxyhemoglobin, correlation can be made between flow-independent T2 measurement of blood in the SMV and oxygen saturation in an in vivo animal model (71,72). Identifying low oxygen saturation in the SMV compared with that in the inferior vena cava (IVC) suggests ischemia (71,72).
When the celiac and the SMA are patent, the possibility of branch vessel stenosis and regional ischemia can be assessed by looking at bowel wall enhancement before and after IV application of contrast material. Bowel mucosa normally enhances avidly immediately after gadolinium injection. Regional areas of diminished or delayed bowel enhancement are suggestive of regional ischemia. The combination of morphologic 3D contrast MRA of the splanchnic vasculature with functional assessment of mesenteric flow holds considerable promise as the emerging modality of choice for evaluation of patients suspected of mesenteric ischemia.
Portal Venous Magnetic Resonance Angiography
Both 2D and 3D TOF and PC techniques provide accurate evaluation of the portal venous system. However, they have been widely replaced by fast CE MRA due to their inherent limitations (motion artifacts, acquisition time). Nevertheless, PC MRA can still be used to determine the direction of portal venous blood flow by acquisition of an axial or an oblique plane, perpendicular to the portal vein with a VENC between 30 and 50 cm/second. Alternatively, a simple and fast assessment of flow direction can be achieved by using TOF with a saturation band to suppress a given direction of flow (Fig. 19-6).
The imaging plane for CE MRA of the portal venous system can be set to either coronal or axial. The axial plane permits a more thorough evaluation of the hepatic parenchyma and displays the main portal vein and its branches in-plane, which generates higher resolution as compared with reformats. However, the coronal plane has the advantage of including the mesenteric veins as well as the retroperitoneal collaterals in case of portal vein obstruction. As CE MRA of the portal venous system often is accomplished at the end of an MRA examination, the acquisition time should be kept as short as possible (i.e., below 30 seconds) in order for patients to perform satisfactory breath holds. Slice thickness should not be more than 5 mm. This approach provides sufficient coverage of the portal venous anatomy and the liver in the majority of patients.
In patients with suspected concomitant parenchymal pathology of the liver, additional T1- and T2-weighted MRI is beneficial in order to identify and characterize hepatic lesions. In case of biliary obstruction, pancreatitis, or ampullary carcinoma, a turbo spin echo magnetic resonance cholangiopancreatography (MRCP) sequence acquired in oblique coronal planes is useful.
Normal Anatomy and Congenital Anatomic Variants
Mesenteric Arteries
The blood flow to the viscera and the intestinal tract is supplied through the three major anterior branches of the abdominal aorta: the CA, the SMA, and the IMA (Fig. 19-7). A multitude of variants exists with regard to the origin of these vessels from the aorta and their branches. With CE 3D MRA and CTA, the detailed assessment of the normal and abnormal vascular anatomy in the majority of cases can readily be displayed and analyzed.
Celiac Axis
The CA arises from the ventral surface of the aorta at the thoracolumbar level. It supplies the upper abdominal viscera. In as many as two thirds of patients, the CA has a classic branching pattern into three major vessels: the splenic artery, common hepatic artery (CHA), and left gastric artery (LGA) (Fig. 19-8). The branching pattern of the CA in the remaining third of patients is highly variable. In the most common variants, the splenic, the CHA, or the LGA may arise directly from the aorta or from the SMA. The proper hepatic artery is the distal continuation of the CHA, arising after the origin of the gastroduodenal artery (GDA).
The CHA normally divides into the left and right hepatic artery, a branching pattern that is present in approximately 55% of all individuals; the other half have replaced or accessory hepatic arterial branches. The GDA arises from the CHA in roughly 75% of patients and usually presents two main branches: the superior pancreaticoduodenal artery and the right gastroepiploic artery. The superior pancreaticoduodenal artery forms an anastomotic arcade with the inferior pancreaticoduodenal artery that arises from the SMA. The GDA-pancreaticodoudenal arcade serves as an important collateral pathway in the setting of visceral artery occlusion.
The CHA normally divides into the left and right hepatic artery, a branching pattern that is present in approximately 55% of all individuals; the other half have replaced or accessory hepatic arterial branches. The GDA arises from the CHA in roughly 75% of patients and usually presents two main branches: the superior pancreaticoduodenal artery and the right gastroepiploic artery. The superior pancreaticoduodenal artery forms an anastomotic arcade with the inferior pancreaticoduodenal artery that arises from the SMA. The GDA-pancreaticodoudenal arcade serves as an important collateral pathway in the setting of visceral artery occlusion.
In approximately 3% of individuals, there is a large anastomotic connection between the proximal celiac, common hepatic, or splenic artery and the proximal SMA, called the arc of Buhler (73,74). The arc of Buhler represents a persistent embryologic channel and serves as a collateral from the SMA to the proximal celiac (Fig. 19-9). Note is made that the arc of Buhler is frequently confused with the dorsal pancreatic arteries, and differentiation is sometimes difficult (73).
Splenic Artery
The splenic artery usually stems from the CA, but it may occasionally arise from the aorta or SMA. The course of this vessel varies because of its high degree of tortuousity. According to a classification by Kupic et al. (75), the splenic artery can be divided into four main segments including: (a) the suprapancreatic part, which is the first 1 to 3 cm directly behind the origin; (b) the most tortuous pancreatic part, which lies on the dorsal surface of the pancreas; (c) the prepancreatic section, which runs on the anterior surface of the pancreas; and finally, (d) the prehilar part, which courses between the pancreas and the spleen. Between 2 to 10 short gastric branches arise from the splenic artery and supply the cardia and the fundus of the stomach. The most frequent origin of these vessels is the splenic terminals.
The splenic artery usually gives off the dorsal pancreatic artery (superior pancreatic branch of Testut), which sustains the dorsal and ventral surfaces of the pancreas in the region of the neck. Two right branches arise from the dorsal pancreatic artery, one anastomosing with the superior pancreaticoduodenal artery, the other directly supplying the uncinate process of the pancreas.
Additional pancreatic branches off the splenic artery include the irregularly observed transverse pancreatic artery as well as small splenic branches to the body and the tail of the pancreas. These small branches run in an almost vertical direction and can be the only vessels demonstrated to the left part of the body and the tail of the pancreas. The largest artery of the latter is named the pancreatic magna artery. This artery frequently courses obliquely to the left after entering the pancreas. Then, it subdivides into right branches, which anastamose with terminal branches of the transverse pancreatic artery. Another more or less constant branch is the caudal pancreatic artery, which originates either from the distal splenic artery in the hilum of the spleen or from the left gastroepiploic artery. However, these rather small vessels can hardly ever be appreciated with cross-sectional imaging techniques.
The left epiploic branch arises from the distal part of the splenic artery and descends along the left side of the greater curvature of the stomach in an anterior layer of the omentum and anastomoses with the right gastroepiploic arch to form the arcus arteriosus ventriculi inferior of Hyrtl. This vessel may supply a rather large amount of blood to the spleen through inferior polar branches. The left epiploic branch is an important branch of the left gastroepiploic artery, which descends in the posterior layer of the greater omentum inferior to the transverse colon and constitutes the left limb of the arcus epiploicus magnus of Barkow. The right limb is formed by the right epiploic branch arising from the right gastroepiploic branch or the transverse pancreatic artery. The arch of Barkow supplies the transverse colon with multiple ascending branches.
Accessory superior or inferior polar splenic arteries might be encountered arising as a branch from various parts of the splenic artery. Rarely, polar arteries originate from the CA. Polar arteries have a tortuous course and give off multiple small branches to adjacent viscera.
Gastroduodenal Artery
The GDA arises from the CHA in most cases; however, it may also originate from an aberrant right or left hepatic artery. The GDA has a relatively variable course in a caudal direction, largely depending on the degree of filling of the stomach and on the size and position of the liver and the gallbladder (Fig. 19-10).
Pancreaticoduodenal Arcades
The superior and inferior pancreaticoduodenal arteries represent the pancreaticoduodenal arcades, which show wide interconnections through anterior and posterior branches forming single or double arcades on the corresponding sites of the pancreas. While the superior arcades often arise directly from the GDA, the inferior arteries arise as a common branch directly from the posterior aspect of the SMA or, more frequently, from the first branch of the jejunal artery coursing behind the SMA.
Table 19-1 Hepatic Arterial Variants According to Michels (N = 200) | ||||||||||||||||||||||||||||||||||||
---|---|---|---|---|---|---|---|---|---|---|---|---|---|---|---|---|---|---|---|---|---|---|---|---|---|---|---|---|---|---|---|---|---|---|---|---|
|
Common Hepatic Artery
The CHA also shows a highly variable course along the cranial border of the body and the head of the pancreas. The course is variable in direction, but tortuous courses are usually not observed. According to Michels (76,77,78,79), anatomic variations most often affect the origin of the hepatic artery. Table 19-1 provides a list of the variations, as noted on the autopsy series by Michels (80).
Most commonly, the CHA arises from the celiac trunk, gives off the GDA and the right gastric and supraduodenal artery, and then becomes the proper hepatic artery, which courses obliquely within the anterior hepatoduodenal ligament. The proper hepatic artery then gives off the cystic artery and bifurcates into the right and left hepatic arteries, with a middle hepatic artery that may arise from either the right or left hepatic arterial branch (79). As described in Table 19-1, this conventional arterial pattern occurs only 55% of the time.
The replaced right hepatic artery is a common variant that traverses the pancreatic head as it passes from the SMA to the liver, providing branches to the pancreas (Fig. 19-11). Another common variant is the replaced left hepatic artery, which arises from the LGA, usually associated with the right and middle hepatic artery arising from the proper hepatic artery (Fig. 19-12). Less frequent variations include an accessory left hepatic artery from the LGA, an accessory right hepatic artery from the SMA, and the entire CHA arising from the SMA without any hepatic artery arising from the CA. Many of the major variations described by Michels in his dissection of the arterial trees of cadavers have also been detected in vivo with CTA and MRA techniques, and some additional variations have been demonstrated as well (81) (Figs. 19-13, 19-14).
Superior Mesenteric Artery
The SMA arises from the ventral aspect of the aorta approximately 1 to 20 mm below the origin of the celiac trunk at the level of the twelfth thoracic to the second lumbar
vertebral bodies. It courses ventrally and caudally over the uncinate process of the pancreas and follows the small bowel mesentery into the right lower quadrant. A single celiomesenteric trunk rarely arises directly from the aorta and divides into the respective vessels. The first part of the SMA is immediately behind the body of the pancreas and can also be completely surrounded by this organ when the uncinate process of the pancreas extends medially. The relationship of the SMA to the surrounding tissue can nicely be studied with CE CT and MR. US permits detection of this vessel in as much as 91% of cases (82). The width of the main stem of the SMA varies from 6 to 13 mm in diameter (83). The course of the very proximal SMA varies from a 45- to 90-degree angle to the aorta, depending on the amount of adipose tissue in the abdomen.
vertebral bodies. It courses ventrally and caudally over the uncinate process of the pancreas and follows the small bowel mesentery into the right lower quadrant. A single celiomesenteric trunk rarely arises directly from the aorta and divides into the respective vessels. The first part of the SMA is immediately behind the body of the pancreas and can also be completely surrounded by this organ when the uncinate process of the pancreas extends medially. The relationship of the SMA to the surrounding tissue can nicely be studied with CE CT and MR. US permits detection of this vessel in as much as 91% of cases (82). The width of the main stem of the SMA varies from 6 to 13 mm in diameter (83). The course of the very proximal SMA varies from a 45- to 90-degree angle to the aorta, depending on the amount of adipose tissue in the abdomen.
The first branch of the SMA normally is the inferior pancreaticoduodenal artery, which passes from the right side directly to the duodenum and the pancreas. This vessel normally arises from the main stem of the SMA or from the first jejunal artery. Less frequently, it may arise as a single artery or as one posterior and one anterior branch, each with separate origins. An intercommunicating arterial arcade between the inferior pancreaticoduodenal artery and the first jejunal artery can be found in roughly 60% of individuals (76). This arcade supplies the fourth part of the duodenum and simultaneously represents an important anastomotic collateral in the case of arterial occlusive disease (77).
The jejunal and ileal branches vary in size and number. These arteries originate from the left side of the SMA. As no strict anatomic border between the jejunum and ileum exists, there is no way to discriminate where jejunal arteries end and ileal arteries begin. Therefore, the simplest rule is to regard those arteries arising proximal to the ileocolic artery as jejunal branches and those distal to this landmark as ileal arteries. There are between 2 and 7 jejunal arteries but up to 17 ileal arteries (excluding those supplying the terminal ileum).
The size and the width of the ileal arteries decrease distally, and the smallest vessels are observed in the terminal ileum. The latter is supplied by three to fifteen separate branches arising from the ileal branch of the ileocolic artery. This ileal arch anastomoses directly with the most distal part of the SMA to form a distal arcade.
The size and the width of the ileal arteries decrease distally, and the smallest vessels are observed in the terminal ileum. The latter is supplied by three to fifteen separate branches arising from the ileal branch of the ileocolic artery. This ileal arch anastomoses directly with the most distal part of the SMA to form a distal arcade.
Such intra-arterial arcades are a characteristic feature of the mesenteric circulation. However, the number and extent of such arcades differs between distinct parts of the bowel: While the midportion of the small bowel shows the largest number of arcades, they are rare in the terminal ileum. Those arcades close to the mesenteric border let off the long vasa recta, which penetrate the bowel wall and enter the submucosa layer. The short vasa recta arise from the last arcade or directly from the long vasa recta and enter the submucosa of the intestinal wall from the mesenteric border. A rather tight interarterial anastomotic network among the vasa recta is present in the small bowel wall, while the number of such connections in the large bowel is comparatively low (Fig. 19-15).
The only constant artery arising from the right side of the SMA is the ileocolic artery, which supplies the terminal ileum, the appendix, the cecum, and the proximal part of the ascending colon. Extension of supply to more distal bowel regions may occur when the right colonic artery is absent, occluded, or directly originates from the ileocolic artery. The ileocolic artery gives off an ileal and a colic artery, which usually communicate through an ileocolic arcade. The ileal artery communicates directly with the SMA. The
right colic artery has a highly variable origin from the SMA, the middle colic, or ileocolic artery. It supplies the ascending colon and the hepatic colonic flexure. The middle colic artery usually arises from the first part of the SMA at the level of the first jejeunal artery and supplies the transverse colon. Rarely, an accessory middle colonic artery is present and is then named the middle mesenteric artery. The left branch of the middle colic artery is in direct communication with the left colic artery, which originates from the IMA and herewith forms the most important collateral pathway for the mesenteric circulation (38,69,70) (Fig. 19-16). The next most functionally important collateral is the arc of Riolan, also called the central anastomotic artery, or the meandering mesenteric artery, a short, direct, and retroperitoneal connection from the root of the SMA (or one of its primary branches) to the IMA (or one of its branches). The marginal artery of Drummond is an additional collateral pathway that supplies the arterial arcades along the mesenteric border of the colon.
right colic artery has a highly variable origin from the SMA, the middle colic, or ileocolic artery. It supplies the ascending colon and the hepatic colonic flexure. The middle colic artery usually arises from the first part of the SMA at the level of the first jejeunal artery and supplies the transverse colon. Rarely, an accessory middle colonic artery is present and is then named the middle mesenteric artery. The left branch of the middle colic artery is in direct communication with the left colic artery, which originates from the IMA and herewith forms the most important collateral pathway for the mesenteric circulation (38,69,70) (Fig. 19-16). The next most functionally important collateral is the arc of Riolan, also called the central anastomotic artery, or the meandering mesenteric artery, a short, direct, and retroperitoneal connection from the root of the SMA (or one of its primary branches) to the IMA (or one of its branches). The marginal artery of Drummond is an additional collateral pathway that supplies the arterial arcades along the mesenteric border of the colon.
Pancreatic and pancreaticoduodenal arteries may also serve as collateral pathways in cases of stenosis or occlusion of the main stem of the SMA (Figs. 19-17, 19-18). Further collaterals for bowel supply in case of vascular occlusion include the epiploic arteries, retroperitoneal parietal arteries, renal capsular arteries, inferior phrenic arteries, and arteries for the adipose tissue of the mesentery. Dilatation of such collateral vessels may be extreme and lead to aneurysms as indirect signs of occlusive disease. In addition to these three more common collaterals, more than 50 collateral pathways in the small and
large bowels have been listed by Michels (77), and a detailed description of these anastomoses can be found in his work.
large bowels have been listed by Michels (77), and a detailed description of these anastomoses can be found in his work.
Superior Mesenteric Vein
The SMV follows the course of the corresponding artery. Anatomy and flow are displayed to best extent on CT, MRI, and MRA or duplex and color Doppler US. The SMV usually can be observed on cross-sectional imaging being to the right and anterior to the SMA.
Inferior Mesenteric Artery
The IMA arises from the left anterior wall of the abdominal aorta approximately at the level of the third lumbar vertebra. After descending parallel to the aorta for 2 to 4 cm, it gives off the left colic artery, which has an ascending course. The left colic artery then gives off sigmoid arterial branches. The remaining IMA gives off additional sigmoid branches not originating from the left colic artery. Below the iliac arteries, the IMA becomes the superior hemorrhoidal artery. Branches of the left colic artery and the sigmoid arteries form the large arterial channel of the mesocolon, the marginal artery of Drummond. This artery represents a complex arcade fed by branches from the ileocolic, right colic, middle colic, left colic, and upper sigmoid arteries and thereby represents an important collateral in case of occlusion in the aforementioned vascular territories. There is great variability in the size of the IMA in adults (1 to 6 mm in diameter). Reflecting the small size of the IMA and its subtle branches, these vessels are difficult to consistently image with MRA (84).
Clinical Relevance of Splanchnic Arterial Anatomic Variations
Variations in the splanchnic arterial anatomy occur in more than 40% of patients. For this reason, pretherapeutic planning for hepatic resections, liver transplantations, resection of retroperitoneal lymph nodes, chemoinfusion pump placement, surgical shunting, or other abdominal operations may require mapping of the visceral arterial anatomy. In the past, this was done by conventional angiography for the fine detail necessary to identify variations involving tiny arteries. However, for evaluation of the splanchnic artery origins and major branches, 3D contrast MRA in its latest implementation is sufficient. In those patients being considered for a surgical renal revascularization, it is important to know the status of the splanchnic arterial anatomy as the source of inflow to a splenorenal or hepatorenal bypass graft.
Portal Venous System
The liver has a double blood supply: the proper hepatic artery divides into the right and left hepatic artery and carries oxygenated blood to the liver, and the portal vein carries venous blood from the GI tract to the liver (Fig. 19-19). The venous blood from the GI tract drains into the superior and inferior mesenteric veins; these two vessels are then joined by the splenic vein (whose tributaries include the short gastric
veins, the gastroepiploic vein and pancreatic veins) just posterior to the neck of the pancreas to form the portal vein, which runs cephalad and obliquely toward the right to the hilum of the liver, where it is the most posterior structure in the hepatoduodenal ligament. The main portal vein receives the coronary vein and then splits to form the right and left branches, each supplying about half of the liver.
veins, the gastroepiploic vein and pancreatic veins) just posterior to the neck of the pancreas to form the portal vein, which runs cephalad and obliquely toward the right to the hilum of the liver, where it is the most posterior structure in the hepatoduodenal ligament. The main portal vein receives the coronary vein and then splits to form the right and left branches, each supplying about half of the liver.
In adults, the length of the main portal vein is typically 8 cm, and the diameter of the portal vein after its confluence ranges from 9 to 11 mm in diameter. Variations in the branching pattern of the main portal vein are less common than variations in the hepatic arteries, veins, and bile ducts, with a reported incidence of 0.09% to 24% (85,86,87). For the majority of cases, the main portal vein divides into a short, oblique right portal vein and a transversely oriented left portal vein (88). The right portal vein quickly branches within the right lobe of the liver to supply the anterior segment (V and VIII, and, variably, IV), and the posterior segment (VI and VII) of the right lobe (Fig. 19-20). The cystic vein drains into the right portal vein before the right portal vein enters into the right lobe of the liver. As a single trunk, the left branch of the portal vein turns transversely to the left between the quadrate and caudate lobe. Portal branches to the caudate lobe arise from the transverse portion of the left portal vein, from the first part of the right portal vein, and/or directly from the portal trunk. The left portal vein has a long transverse course to the level of the umbilical fissure, where it gives off medial branches to segment IV and lateral branches to segments II and III.
Hepatic Veins
On entering the liver, the blood drains into the hepatic sinusoids. Three major hepatic veins originating from the right and left lobe and from the middle portion of the liver join the IVC just inferior to the diaphragm (76,77,78,79).
The right, middle, and left hepatic veins enter the retrohepatic IVC just below the diaphragm, usually 2 cm inferior to the right atrium (89). The right hepatic vein drains separately into the IVC, but the middle hepatic vein and the left hepatic vein may share a common trunk (65% to 85%) (90). In addition to the three main hepatic veins, small accessory (short) hepatic veins from the pericaval liver segments drain directly into the IVC inferior to the drainage of the three major veins (91) (Fig. 19-21). These short hepatic veins usually drain the right lobe or caudate lobe and need to be accounted for when a living donor hepatic transplant candidate is being evaluated (92,93,94). The major short hepatic veins are the inferior or middle right hepatic veins. All of the venous drainage, major hepatic venous and accessory venous drainage, exits the liver in its bare area.
Portal Vein Variants
Portal venous variants are primarily important for interventionalists performing transjugular intrahepatic portosystemic shunt (TIPS) procedures and for transplant surgeons evaluating candidacy of patients. Venous anomalies include early branching of the portal vein, a trifurcation of the portal vein (late origin of the left portal vein at the bifurcation of the right portal vein into its anterior and posterior branches), an anomalous origin of the left portal vein from the right anterior portal vein branch, and early origin of the right posterior portal vein. These venous anomalies are important preoperative findings. The more branches, the more anastomoses the surgeon must make, increasing the potential complications (96,97).
The right anterior portal vein (which supplies segments V and VIII, and variably IV), may arise from the left portal vein distal to the bifurcation. This poses a problem for living donor donation of either the right or the left lobe of the liver. If this variant is not appreciated preoperatively, resection of the left portal vein will devascularize segments V, VIII, and, variably, IV. In these patients, resection of the left portal vein must follow the takeoff of the aberrant right anterior portal vein.
The left portal vein may have a very short extraparenchymal segment. When explanted, the small extrahepatic segment will be insufficient in length for anastomosis to the recipient’s portal vein, and an interpositioned venous graft will need to be placed.
Some venous anomalies are absolute contraindications to liver transplantation. Portal vein trifurcation is considered a contraindication to living related liver transplantation of the right lobe of the liver (segments V–VIII). Additionally, absence of the living donor’s right portal vein is a contraindication to liver transplantation.
Hepatic Vein Variants
Similar to portal venous variants, hepatic venous variants are most important for delineating aberrant drainage prior to transplantation. In evaluation of hepatic venous variants prior to right lobe living donor transplantation, the caliber of the right main hepatic vein should be determined. If the right main hepatic vein is small, portions of the right lobe may be drained via tributaries leading to the middle hepatic or inferior right hepatic veins (Fig. 19-22). Thus, viability of the donated right lobe will depend on preservation of all the venous drainage.
Common anatomic hepatic venous variants are an accessory inferior right hepatic vein, which drains Couinaud segment VI (18% of the normal population) (Fig. 19-23), and a middle right hepatic vein, which drains Couinaud segment V (5.5%) (90). The accessory inferior right hepatic vein is commonly seen deep to the posterior division of the right portal vein.
Clinical Applications
With 3D contrast MRA and multidetector spiral CTA, the main visceral vessels are displayed with excellent diagnostic accuracy. However, neither achieves the spatial resolution of selective catheter angiography, limiting visualization of smaller branch vessels.
Mesenteric Ischemia
Reflecting the dramatic increase of life expectation and a growing number of elderly patients, the last decades have seen an increasing frequency in the diagnosis of ischemic disorders of the intestines. Partly, this is due to a real increase in incidence of atherosclerotic changes of the arterial tree in an aging population. However, this is also a result of the increasing awareness (and thus, increasing diagnosis) of clinical syndromes that have secondary changes of the intestinal blood flow.
The demonstration of significant stenoses in two of the three main mesenteric vessels in conjunction with appropriate clinical symptoms underscores the diagnosis of mesenteric ischemia (63). However, many patients with stenoses in the mesenteric vessels are asymptomatic. Autopsy series in unselected patient populations document significant mesenteric atherosclerosis in 35% to 70% of patients (98). This incidence is greater in selected patient populations with risk factors for atherosclerosis, including diabetes, hyperlipidemia, smoking, and hypertension. In performing duplex sonography of the mesenteric arteries in 184 asymptomatic patients, Roobottom and Dubbins (1) found total occlusion or >70% stenosis in 3% of adult patients under age 65 and an 18% incidence in patients over age 65. In 11% of patients, the disease was found in only one vessel; 7% had disease in both the celiac or SMA (1). The most common site of significant atherosclerotic disease is the origin of the celiac artery (86%), followed by the IMA (70%) and SMA (55%) (98).
Heretofore, selective angiography has been considered the gold standard for establishing the diagnosis of this disease (99). However, CTA and CE MRA have the potential to become a definitive noninvasive tool for the diagnosis of CMI. These cross-sectional techniques not only can provide information about anatomy, patency, and stenosis of mesenteric vessels but are also becoming a modality of
choice in the selection of patients suspected of having mesenteric ischemia who may benefit from endovascular therapy or surgery.
choice in the selection of patients suspected of having mesenteric ischemia who may benefit from endovascular therapy or surgery.
Pathophysiology
Mesenteric ischemia results from any reduction in blood flow to the intestine, as in shock or heart failure, as well as from either local morphologic or functional changes. Such inadequate circulation may derive from focal atheromatous emboli, luminal narrowing of the mesenteric vessels, vasculitis as part of a systemic disease, mesenteric venous thrombosis, and mesenteric vasoconstriction (nonocclusive mesenteric ischemia). Independent of the cause, intestinal ischemia has the same end results, ranging from completely reversible functional changes to total hemorrhagic necrosis of the bowel. However, numerous collateral pathways exist between the small and large bowel. The major vascular supply of the bowel depends on the CA and the SMA, while the supply source for the lower intestine is the IMA. In case of stenosis or occlusion to one of these vessels, the development of a rich collateral circulation between these arteries can mostly prevent major abdominal symptoms (38,69,70) (Fig. 19-24).
Most patients will not become clinically symptomatic unless blood flow in at least two major vessels is compromised (63,100). Occlusion of two of the three vessels occurs frequently without evidence of ischemia, and total occlusion of all three vessels has also been observed in totally asymptomatic patients. Collateral pathways around occlusions of small arterial branches in the mesentery are provided by the primary, secondary, and tertiary arcades in the small bowel and the arc of Riolan and the marginal artery of Drummond in the colon. Within the bowel wall, a tight network of closely intercommunicating arteries provides a second level of arterial supply to short intestinal segments when extramural supply has been stopped.
At rest, intestinal blood flow is estimated between 50 and 1,200 mL/minute, which equates to approximately 10% to 20% of the cardiac output (101). This volume may modestly increase after eating or may decrease during exercise, but major changes are induced by the level of sympathetic activity. Short-term vascular constriction as modulated by alpha- and beta-adrenergic receptors can be tolerated by the intestine. Similarly, the lumen of the SMA might be reduced by 80% without appreciable diminution in blood flow. Interestingly, intestinal ischemia can be present without intestinal necrosis, and intestinal necrosis may be present with normal blood flow if blood flow is measured after a transient episode of ischemia has been relieved.
Splanchnic ischemia syndromes are classified for simplicity as either acute or chronic. However, the term chronic is somewhat of a misnomer, in that the majority of “chronic” cases are caused by acute mesenteric ischemic events that exhibit immediate or delayed effects of the circulatory insult.
Acute Mesenteric Ischemia
Acute obstruction of mesenteric arteries or their branches causes symptoms that are difficult to interpret clinically. Hence, the correct diagnosis is likely to be delayed, potentially with serious consequences. Abdominal pain of sudden onset is the most often clinical presentation of AMI. Occlusive emboli, thromboses, mesenteric vasoconstriction (nonocclusive ischemia), vasculitis, and mesenteric venous thrombosis are known causes for AMI. The clinical symptoms are basically the same for all causes and depend mainly on the duration of the ischemia. Concomitant renal artery or splenic artery occlusion with associated renal or splenic infarction has to be considered in such a clinical scenario. While occlusion of small peripheral arterial arcades or separate colic arteries cannot be visualized with CT or MR, indirect signs of mesenteric vascular occlusion have to be appreciated. Parts of the bowel wall prone to hemorrhagic necrosis are usually swollen, and the lumen is compressed. In addition, one might encounter effusion or hemorrhage in the compromised bowel and mesentery. Numerous lymph nodes with pathologically increased diameters can be appreciated. Mortality is extremely high in this disease. Treatment includes catheter angiography with intra-arterial administration of vasodilators or thrombolytic agents. In the majority of cases, patients with AMI are referred to a surgical procedure.
Emboli
As much as 50% of cases of AMI are caused by SMA emboli, which usually originate from a mural or an atrial thrombus in patients with atrial fibrillation (Fig. 19-25). While rheumatic valvular disease was most commonly associated with such thrombi in the past, atherosclerotic changes have become much more important today. Paradoxical embolism to the SMA from deep venous thrombosis has also been observed. Twenty-five percent of patients with acute onset of abdominal pain and suspected mesenteric emboli have a previous history of peripheral arterial embolism, and many patients will present with concomitant emboli in other arteries (101). Depending on the localization of the embolus, it can be visualized with CT or MR in the proximal part of the SMA. Using catheter angiography, emboli can be delineated as sharp, rounded filling defects. The artery may be completely occluded, but more often, the embolus only partially obstructs the blood flow. In addition, mild vasoconstriction might be detected proximal and distal to the embolus (102,103). Emboli tend to lodge at points of vascular divisions and narrowings—for example, distal to the origin of a major branch, such as the origin of the inferior pancreaticoduodenal artery, the origin of the middle colic artery, or the origin of the ileocolic artery. Lodging distal to the inferior pancreaticoduodenal artery or the middle colic artery is problematic, as it isolates the distal small bowel from collaterals that connect to these arteries.
Thrombosis
An acute thrombosis of the SMA may also lead to acute ischemia of the intestine. SMA thrombosis is less common than embolic disease. Thrombosis is almost always superimposed on severe atherosclerotic narrowing, most commonly in the region where the main artery arises. Since the acute onset of the episode represents the end stage of a chronic process of luminal narrowing, as much as 50% of patients have experienced abdominal pain during the preceding weeks or months. These patients typically suffer from severe atherosclerotic
changes throughout the arterial system, and a prior history of coronary, cerebral, or peripheral arterial ischemia is frequently present. Apart from local intestinal pathologies, both MRA and CTA nicely display the extent of atherosclerosis throughout the arterial tree. However, the differentiation between an older thrombus and an embolus may be demanding. The absence of dilated collateral vessels or the presence of inadequate contrast enhancement within the SMA indicates an acute occlusion. Prompt surgical intervention is indicated irrespective of additional findings in these cases.
changes throughout the arterial system, and a prior history of coronary, cerebral, or peripheral arterial ischemia is frequently present. Apart from local intestinal pathologies, both MRA and CTA nicely display the extent of atherosclerosis throughout the arterial tree. However, the differentiation between an older thrombus and an embolus may be demanding. The absence of dilated collateral vessels or the presence of inadequate contrast enhancement within the SMA indicates an acute occlusion. Prompt surgical intervention is indicated irrespective of additional findings in these cases.
Nonocclusive Mesenteric Ischemia
Nonocclusive mesenteric ischemia occurs in patients with low-flow syndromes. Thus, when it occurs, it is observed in elderly and/or extremely ill patients who are hospitalized in coronary/intensive care units. These patients develop vasoconstriction in response to a decrease in cardiac output, hypovolemia, dehydration, vasopressor agents, or arterial hypotension. Predisposing conditions include myocardial infarction, congestive heart failure, aortic or mitral insufficiency, renal and hepatic disease, and recent cardiac or major abdominal operations. Additionally, pulmonary edema, cardiac arrhythmia, or shock has been present. Digitalis, a commonly prescribed drug, has a constrictive effect on the mesenteric circulation and may produce bowel necrosis if given in too high a dose. In the majority of cases, the underlying cause of the inadequate blood flow is not amenable to interventional or surgical correction. Diagnosis of the nonocclusive subtype of AMI is demanding. Severe pancreatitis may display features like those of a nonocclusive mesenteric ischemia. On MRA or CTA imaging, findings suggestive of a nonocclusive etiology of mesenteric ischemia include findings of luminal irregularities of the splanchnic vasculature in conjunction with indirect imaging signs of mesenteric ischemia (i.e., ascites, impaired contrast enhancement of circumscribed bowel wall areas). If this diagnosis is suggested early, treatment is the optimization of the patient’s hemodynamic and volume status, with treatment of the underlying medical condition. Selective injection of the mesenteric vasculature with IV papaverine or glucagon may also increase blood flow to the bowel. If the patient demonstrates a surgically correctable lesion, an emergent operation should be performed.
Vasculitis and Iatrogenic Causes
In addition to embolus and thrombosis secondary to atherosclerosis, a wide variety of other vascular disorders may cause acute occlusion of small mesenteric arteries. High-resolution CTA and MRA can provide information about abnormalities due to lupus erythematosus, rheumatoid disease, and other types of vasculitis (104,105). Intimal arterial hyperplasia and thrombus formation in small mesenteric arteries may also cause infarction. Bowel ischemia secondary to abdominal surgery, catheterization, and trauma are other important causes of vascular occlusions that can be detected and defined by cross-sectional imaging.
Mesenteric Venous Thrombosis
Mesenteric vascular occlusion is usually subacute in presentation, with a vague clinical prodrome that may last days to weeks prior to disease recognition. The most common causes of venous thrombosis include inflammation, peritonitis, portal hypertension, and hypercoagulable states associated with various malignancies and the use of oral contraceptives. Paraneoplastic syndromes most commonly affect the SMV. Since the diagnosis of an SMV thrombus with angiography is notoriously difficult and the angiographic findings are indistinct, cross-sectional imaging techniques play an important role in diagnosing this form of mesenteric ischemia (Figs. 19-26, 19-27). Surgical therapy consists of thrombectomy, resection of infarcted bowel, and anticoagulation. Correction of the underlying medical condition (such as investigation for Factor V Leiden antibodies, antithrombin III deficiency, protein C and S deficiency, etc.) is essential. An extensive discussion on venous thrombosis can be found within Chapter 24).
Inferior Mesenteric Artery
The incidence of acute embolic occlusion of the IMA is much lower than occlusion of the SMA. The larger diameter of the SMA and its nearly parallel course to the aorta combine to invoke a much higher risk for collecting emboli. Acute occlusion of the IMA is generally well tolerated if limited to the proximal portion of the vessel and collateral flow from the SMA is sustained. If this is not the case, occlusion of the IMA may cause infarction of variable segments of the left colon. In some patients, IMA occlusion relates to preceding surgery; in others, it may be associated with atheromatous plaques, blood dyscrasias, saccular aortic aneurysms, and sepsis. Clinically, patients with an acute occlusion of the IMA present with sudden, severe, lower abdominal pain and often bloody rectal discharge. Iatrogenic ligation of the IMA alone during a surgical procedure (e.g., resection of abdominal aortic aneurysms [AAAs]) might be well tolerated when the marginal artery of Drummond is intact. However, if the hypogastric arteries are also ligated and the channels between IMA and SMA are not well established, the outcome may be disastrous.
With acute obstruction of the SMA, the IMA may maintain arterial supply through the aforementioned
marginal artery of Drummond; however, the supply is unlikely to be adequate in most cases.
marginal artery of Drummond; however, the supply is unlikely to be adequate in most cases.
Imaging Diagnosis of Acute Mesenteric Ischemia
Helical CT and CTA (especially when performed with multidetector row scanners) and MRI (particularly gadolinium-enhanced MRA) enable volumetric acquisitions in a single breath hold. MDCT should be considered the primary diagnostic modality for patients with a high clinical suspicion of AMI due to its wide availability, rapid acquisition, and ability to demonstrate air. Conventional angiography is reserved for equivocal cases at noninvasive imaging and is also used in conjunction with transcatheter therapeutic techniques in management of symptomatic portal and mesenteric venous thrombosis (15). With MRA, thrombus in the SMV can easily be delineated on contrast-enhanced T1-weighted transverse images as hypointense luminal filling defect with peripheral contrast uptake. The peripheral contrast enhancement and central hypodense demarcation of the thrombus can also be appreciated on CT images. In
addition to venous thrombosis detection, cross-sectional imaging often detects displacement of the SMV in relation to the SMA. Both CT and MR permit detection of the underlying pathologic condition—for example, external compression from pancreatic tumors or lymph nodes. Vascular compromise—particularly venous but also arterial—is also noted in bowel intussusception, strangulated bowel obstruction, intestinal volvulus, and midgut malrotation.
addition to venous thrombosis detection, cross-sectional imaging often detects displacement of the SMV in relation to the SMA. Both CT and MR permit detection of the underlying pathologic condition—for example, external compression from pancreatic tumors or lymph nodes. Vascular compromise—particularly venous but also arterial—is also noted in bowel intussusception, strangulated bowel obstruction, intestinal volvulus, and midgut malrotation.
Chronic Mesenteric Ischemia
The diagnosis of CMI includes a host of various conditions in which there is insufficient blood flow to the intestine to satisfy the demands of increased motility, secretion, and absorption that develop after meals. The clinical syndrome of CMI is rare. Symptoms include abdominal pain, weight loss, and food aversion (53,63,106,107). The pain is somewhat similar to that arising in the myocardium with angina pectoris or that in the calf muscles in patients with intermittent claudication. Atherosclerotic wall changes of the splanchnic arteries are reported to be the main pathophysiologic mechanism for CMI (40). Although partial or complete occlusions of the CA, SMA, and IMA have been identified frequently in autopsy and angiographic studies, there have been relatively few patients with clinically documented chronic intestinal ischemia. There are many patients with occlusion of two or even all three vessels who remain asymptomatic. Therefore, the diagnosis of mesenteric ischemia remains challenging in clinical practice. Reflecting the risks associated with arterial catheterization, the clinical diagnosis of mesenteric ischemia has been mostly one of exclusion. In addition, the clinical significance of the angiographic demonstration of one or more of these vessels being occluded remains controversial. The dearth of objective means of determining the inadequacy of intestinal blood flow before any morphologic changes of mesenteric ischemia is the major obstacle to identifying patients with CMI.
CMI has been reported with aneurysms of the aorta, the CA, the SMA, congenital and traumatic arteriovenous fistulas involving the hepatic arteries, coarctation of the aorta, and congenital anomalies of the splanchnic vessels. Such cases are rare, however, and atherosclerotic disease in the mesenteric vasculature is the usual cause of intestinal ischemia.
Atherosclerosis commonly affects the splanchnic arteries in individuals over 45 years of age. Luminal narrowing of the major mesenteric vessels is mostly due to a plaque at the aortic origin or within the first 1 to 3 cm of the artery, respectively. Severe stenosis is mostly associated with marked aortic atherosclerosis. As may be expected, patients with severe atherosclerotic changes of the mesenteric vessels have a higher incidence of coronary artery disease and diabetes mellitus (98).
Due to the lack of a specific and reliable diagnostic test for abdominal angina, the diagnosis is based on clinical symptoms, noninvasive cross-sectional imaging, and catheter angiography in selected cases and the exclusion of other GI disease. The median time span between clinical presentation and diagnosis of CMI is more than 18 months for patients with new symptoms and approximately 1 month for patients with recurrent symptoms. Symptoms of mesenteric ischemia may overlap with those of more common intestinal disorders such as peptic ulcer or chronic cholecystitis.
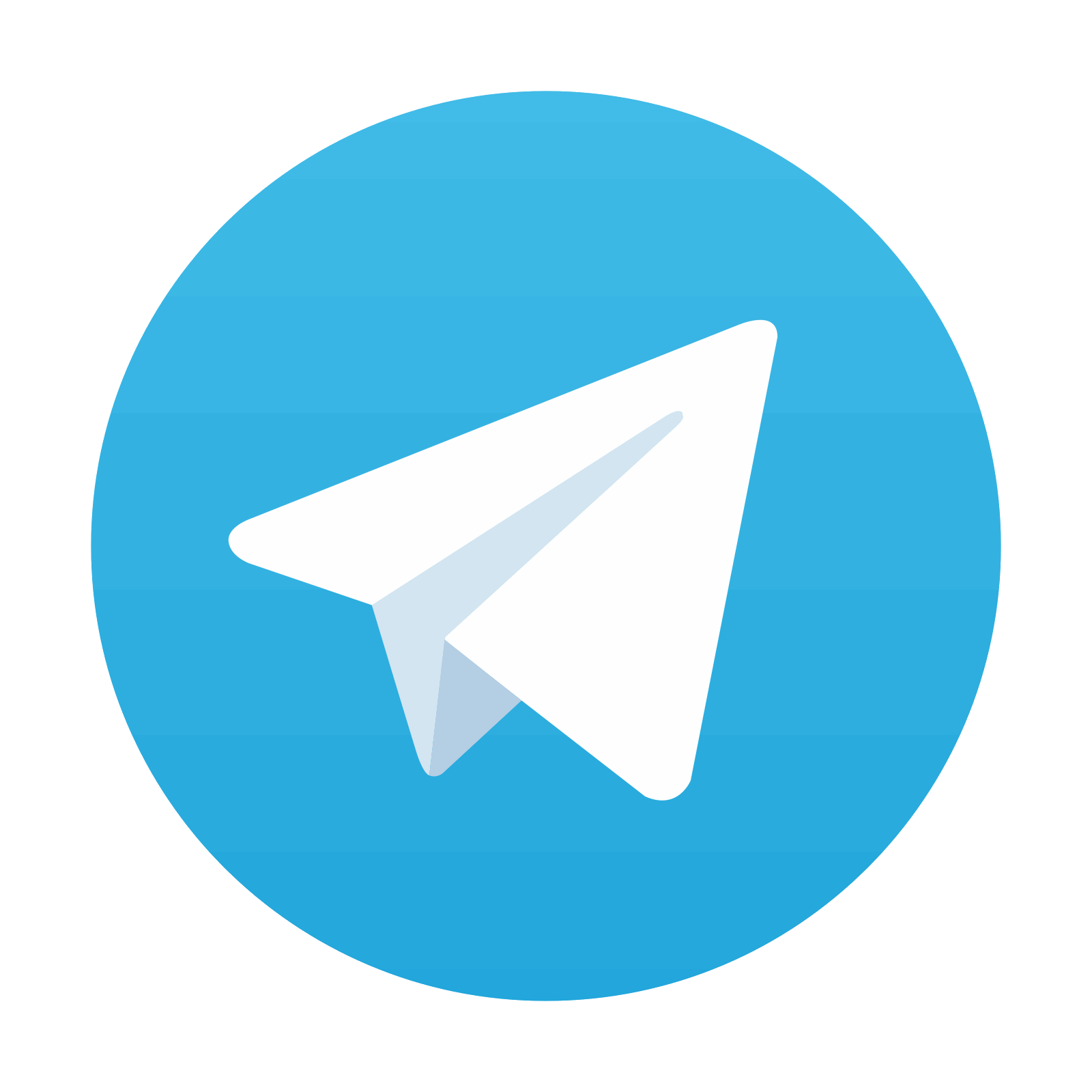
Stay updated, free articles. Join our Telegram channel

Full access? Get Clinical Tree
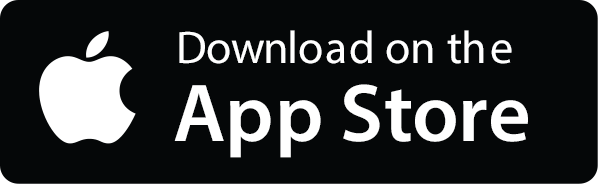
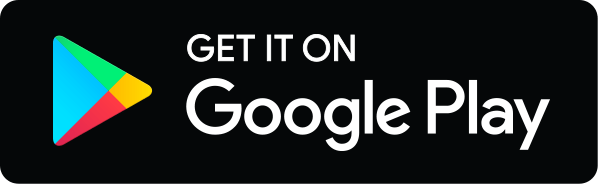
