Transthoracic Echocardiography: Nomenclature and Standard Views
- Meagan M. Wasfy, MD
- Michael H. Picard, MD
A compete transthoracic echocardiographic examination begins with scanning from the standard transducer positions ( Fig. 6.1 ): parasternal (right and left), apical, subcostal, and suprasternal windows. At each transducer position, angulation and rotation of the transducer produces different views of the heart in different planes. At a minimum, sagittal, transverse, and coronal planes through the heart are obtained ( Fig. 6.2 ). Each combination of transducer position and imaging plane has distinct advantages with regard to imaging specific cardiac structures. One goal of combining multiple image planes is to image structures that are perpendicular to the direction of the ultrasound beam to take advantage of the superior axial resolution. Additional views from nonstandard transducer positions and imaging planes, or off-axis views, may be necessary to optimally image pathologic structures or even normal structures in patients whose heart is located in an abnormal position.


Parasternal window
Images from the left parasternal window are obtained by placing the patient in the left lateral decubitus position and placing the transducer adjacent to the sternum the left third or fourth intercostal space. Initially, images should be recorded at a depth that includes at least the descending thoracic aorta to facilitate detection of pericardial and pleural effusions, and posterior mediastinal pathology such as masses.
Long Axis: Left Ventricle
The long-axis view bisects the heart from base to apex in a sagittal plane; in the left parasternal window, this view is obtained by orienting the transducer groove toward the patient’s right shoulder. The optimal image of the long-axis view of the left ventricle (LV) includes the mid and basal LV anteroseptal and inferolateral (or posterior) walls on the left half of the image, the mitral valve (MV) in the approximate center of the image, and the aortic valve (AV), left ventricular outflow tract (LVOT), aortic root, proximal ascending aorta, and left atrium (LA) on the right half ( Fig. 6.3 and Video 6.3). The true LV apex is not visualized. The coronary sinus can be seen in the atrioventricular groove posterior to the mitral annulus, and the descending aorta is seen posterior to the LA external to the pericardium. A portion of the proximal right ventricular (RV) outflow tract is anterior at the top of the image. Proper alignment in this view is essential to obtain accurate determination of the standard measurements of the LVOT, aorta, LA, LV wall thickness, and LV systolic and diastolic diameters, as well as single-dimension estimation of LV ejection fraction and fractional shortening. Better visualization of the ascending aorta may be obtained by moving the transducer up one or two intercostal spaces.

In the left parasternal long-axis view of the AV, the right coronary cusp is typically anterior, and the noncoronary cusp is typically posterior. The anterior and posterior mitral leaflets and their chordal attachments are well visualized. This view identifies any prolapse of the mitral leaflets past the annular plane or excessive anterior motion of the leaflets during systole. Color flow Doppler imaging in the parasternal long-axis view provides an initial assessment of mitral and aortic valve function.
Long Axis: Right Ventricular Inflow and Outflow
From the standard parasternal long-axis view of the LV, angulation of the transducer inferomedially with slight clockwise rotation and movement of the transducer towards the apex will yield a long-axis view of the inflow of the RV. The RV is located at the top left of the image and the right atrium (RA) at the bottom right ( Fig. 6.4 and Video 6.4). Optimal image orientation will exclude left-sided structures and will allow for visualization of the full excursion of the anterior and posterior leaflets of the tricuspid valve (TV). This view is one of several that allows Doppler interrogation of tricuspid regurgitation and its peak velocity for estimation of right ventricular systolic pressure. In addition, the entrance of the coronary sinus and inferior vena cava can be imaged.

The RV outflow view is obtained from the standard parasternal long-axis view of the LV by moving the transducer slightly toward the base and angulating laterally ( Fig. 6.5 and Video 6.5). Here, the long axis of the RV outflow tract, pulmonic valve (PV), and pulmonary artery (PA) are visualized, and velocities through the outflow tract and PV can be obtained. By adjusting the depth and angulation, the pulmonary artery bifurcation can be imaged.

Short Axis
The short-axis view cuts the heart transversely, perpendicular to the long-axis view. In the left parasternal window, the short-axis views are obtained by rotating the transducer 90 degrees from the standard long-axis view, such that the transducer groove is oriented toward the left shoulder.
The transducer is angulated superiorly to image the short axis of the base of the heart ( Fig. 6.6 and Video 6.6). The ideal image will center around the AV in short axis. When the aorta is seen in short axis, the PV is usually seen in long axis (i.e., the aortic and PVs are normally perpendicular to each other). When the AV is in cross section, all three leaflets are seen in the shape of a Y during diastole, and valve morphology can be determined in this view. The origins of the right and left main coronary arteries can often be seen just superior to the aortic annulus extending from the right and left coronary sinuses. Extending inferiorly from the noncoronary cusp of the AV is the interatrial septum, with the LA to its right and RA to its left. Moving clockwise from the RA around the AV are the structures of the right side of the heart: the TV to the left, the RV outflow tract anteriorly at the top of the sector, and the PV and PA to the right. The septal and anterior leaflets of the TV and the long axis of the PV are visualized; Doppler imaging is useful in this view to assess TV and PV function as well as estimate peak tricuspid regurgitation velocity. Slight adjustment of the transducer position may be necessary to optimally image these valves, the RV outflow tract, and the main PA and its branches. Standard measurements of the distal RV outflow tract and the main and branch PAs are made in this view.

When the transducer is angulated slightly inferiorly, the short axis of the mitral valve is visible ( Fig. 6.7 and Video 6.7). Each mitral leaflet has three portions, numbered laterally to medially (or right to left in this view, i.e., P1-3 and A1-3). Color Doppler may be useful in this view to identify the location of mitral regurgitant jets. The short axis of the base of the LV and RV also are in view at this level. Angulating the transducer further inferiorly and/or moving it laterally will image the midventricle with the anterolateral and posteromedial papillary muscles at approximately the 3 and 8 o’clock positions, respectively ( Fig. 6.8 and Video 6.8). Finally, the LV apex is imaged with further inferior angulation and/or lateral movement of the transducer ( Fig. 6.9 and Video 6.9). These three short-axis views are excellent for assessing segmental LV wall motion and thickening. In the ideal images, the normal LV is cut in cross section and is nearly circular; an ellipsoid appearance of the LV usually means that the imaging plane is not perpendicular to the long axis of the LV. In the short-axis views, the RV is typically ellipsoid or crescentic; septal motion and curvature can be assessed for evidence of RV pressure or volume overload.



Right Parasternal Window
To obtain images from the right parasternal window, the patient is positioned in the right lateral decubitus position. The ultrasound probe is placed between the third and fifth intercostal spaces near the costochondral junction and angulated medially. In this view, the proximal ascending aorta is visualized, and transaortic gradients for estimating the severity of valvular aortic stenosis may be obtained ( Fig. 6.10 and Video 6.10).

Apical window
Images from the apical window are obtained by positioning the patient in the left lateral decubitus position and placing the transducer over the palpated point of maximal impulse of the LV apex.
Four-Chamber View
In the apical four-chamber view, the heart is visualized in a plane perpendicular to both the short- and long-axis planes (see Fig. 6.2 ). The optimal position at the true LV apex will create an image in which the length of the LV is displayed from the base at the mitral annulus tapering to the more thin-walled apex ( Fig. 6.11 and Video 6.11); a foreshortened image of the LV will result if the transducer position is not correct. In such cases, the true LV apex will not be visualized. In an ideal four-chamber view, the long axis of the LV is displayed along with the largest dimensions of the annuli of both atrioventricular valves. In the four-chamber view, the inferoseptal and anterolateral walls of the LV are imaged. End-diastolic and end-systolic dimensions of the LV may be obtained in this view and combined with those from the two-chamber view to estimate LV ejection fraction via the biplane method of discs. LV systolic and diastolic function may be assessed in this view using tissue Doppler and other myocardial tracking methods.

The four-chamber view is also useful for determining biatrial size and atrioventricular valve function. The anterior mitral leaflet is adjacent to the septum and the posterior mitral leaflet is adjacent to the lateral wall. The TV annulus is typically displaced several millimeters apically as compared with the mitral valve annulus, with the septal and either the anterior or posterior leaflet visualized. Doppler interrogation of MV and TV function as well as pulmonary venous flow is performed in this view. The right and left inferior pulmonary veins are visualized entering the LA at the bottom of the image.
The RV is a difficult structure to image, given its variable shape and size, but the four-chamber view is one in which several standard RV dimensions and RV function are assessed. The transducer should be moved medially to focus on the RV. From there, the RV diameter at the base and midventricle, and the longitudinal dimension of the RV are measured. Several quantitative measurements of RV systolic function are also made in this view, including the tricuspid annular plane systolic excursion on M-mode, the fractional area change on two-dimensional imaging, and the tricuspid lateral annular systolic velocity (S′) on tissue Doppler imaging.
From the four-chamber view, if the transducer is angulated anteriorly, the LVOT, AV, and aortic root are imaged in the five-chamber view ( Fig. 6.12 and Video 6.12). Here, flow through the LVOT is approximately parallel to the ultrasound beam, which allows for Doppler interrogation of the AV to assess valvular function, including LVOT and transaortic velocities for calculation of AV area and gradients.

Two-Chamber View
The two-chamber view of the LA and LV is obtained via counterclockwise rotation of the transducer from the four-chamber view ( Fig. 6.13 and Video 6.13). The optimal image excludes all right-sided structures as well as the papillary muscles, which are located posterior to the imaging plane. The anterior LV wall is on the right side of the image, and the inferior wall is on the left. In a typical two-chamber view both anterior and posterior leaflets will be imaged.

Three-Chamber (Long-Axis) View
From the two-chamber view, further counterclockwise rotation of the transducer yields the three-chamber view, which is similar to the long-axis view from the parasternal window ( Fig. 6.14 and Video 6.14). In contrast to the parasternal view, in this view the apex is visualized, and the LVOT and AV are oriented such that Doppler interrogation of velocities is possible. In general, the apical views usually provide an intercept angle that is relatively parallel to flow for the AV, MV, and TV.

Subcostal window
To obtain images from the subcostal window, patients should be positioned supine with their knees bent to relax the abdominal wall. The transducer is positioned in the epigastric area and pointed approximately toward the patient’s left shoulder, with the transducer groove toward the patient’s left side. The optimal image in this position yields a view that includes all four cardiac chambers, with the RV at the top of the image and the atria toward the left ( Fig. 6.15 and Video 6.15). In this view, RV diastolic wall thickness can be assessed because it is typically perpendicular to the beam of ultrasound. The position of the interatrial septum perpendicular to the imaging plane also allows Doppler assessment of interatrial communications. This view is also particularly useful for initial evaluation for pericardial effusion and to determine if the effusion can be accessed by the typical needle path of a pericardiocentesis from a subxiphoid approach.

Rotation of the transducer about 90 degrees counterclockwise may yield short-axis views of the heart similar to those in the parasternal window ( Fig. 6.16 and Video 6.16). Imaging the short axis of the heart in the subcostal window may be particularly helpful when parasternal windows are inadequate. It may however be difficult to obtain on-axis subcostal short-axis images of multiple levels of the left ventricle.

From the initial transducer position in the subcostal window, angulation of the transducer inferiorly and toward the patient’s right side, with rotation of the groove of the transducer toward the patient’s right shoulder yields a long-axis view of the inferior vena cava (IVC) ( Fig. 6.17 and Video 6.17). Assessment of IVC diameter and respirophasic collapse, and Doppler interrogation of the hepatic veins as they drain into the IVC are useful for estimating RA pressure. From this view, angulation of the transducer medially will yield a long-axis view of the abdominal aorta. Doppler interrogation of the abdominal aorta in this view is useful to assess for aortic conditions such as aortic dissection, coarctation with persistent diastolic runoff, and for systolic flow reversal as may be observed in aortic regurgitation.

Suprasternal notch window
To obtain images from the suprasternal notch, the patient should be supine with the neck extended. Turning the head to either side may facilitate this view. The transducer is placed in the suprasternal notch and angulated so that the body of the transducer is nearly parallel and just to the left of the patient’s trachea, with the transducer groove pointed toward the right supraclavicular area. This yields a long-axis view of the aortic arch at the top of the image, with the ascending aorta to the left and the proximal descending aorta to the right; the arch branch vessels come off superiorly ( Fig. 6.18 and Video 6.18). The right PA can be seen just under the aortic arch. Doppler interrogation of the descending thoracic aorta is complementary to that of the abdominal aorta in the subcostal view. Clockwise rotation of the transducer with the groove facing posteriorly yields a short-axis view of the aortic arch in this view.

Technical Quality
- Bonita Anderson, DMU (Cardiac), M Appl Sc (Med Ultrasound)
Close attention to technical quality or image optimization in transthoracic echocardiography (TTE) is important for the acquisition of high-quality diagnostic images and to ensure that measurements are accurately performed. For this purpose, the echocardiographer must be familiar with all the controls on the ultrasound machine that can be manipulated to optimize the two-dimensional (2D) images, color Doppler images, and spectral Doppler traces.
Optimizing 2D images
Important controls that can be adjusted to optimize 2D images include the transducer frequency, focus position, sector width, image depth, overall gain, time gain compensation (TGC), and dynamic range. In particular, these controls can be used to optimize spatial, contrast, and temporal resolution. Spatial resolution refers to the ability of the ultrasound machine to detect structures that are anatomically separate and to display them as separate. The best spatial resolution occurs at higher transducer frequencies where the spatial pulse length (and wavelength) is smallest and at the focal zone where the beam is narrowest. Therefore the operator should use the highest possible transducer frequency that allows adequate depth penetration, and the focus should be positioned at the center of the region of interest. In particular, because higher frequency transducers improve spatial resolution, the measurement of small structures is more accurate, and the distinction between closely related anatomic structures is more apparent ( Fig. 7.1 and Video 7.1, A, B ).

Contrast resolution refers to the ability of the ultrasound machine to differentiate subtle differences in echogenicity between anatomic structures and to then display these as visually distinguishable structures. Controls that can be manipulated to improve contrast resolution include the gain and TGC, the dynamic range, and harmonic imaging. The overall gain essentially increases the amplitude (or gain) of the rather weak returning ultrasound signals. In addition, echoes returning from deeper structures become progressively weaker because the ultrasound beam is attenuated, and compensation for this effect is required. This is achieved via the adjustment of TGC, whereby deeper echo signals are amplified more than closer echo signals. The general aim of gain and TGC is to ensure that signals arising from tissues of similar acoustic properties are displayed at the same echo amplitude. The dynamic range (DR) refers to the ratio of the maximum to minimum signal level; that is, the DR is the ratio of the strongest to the weakest echo. The aim of adjusting the DR is to provide the optimal amount of gray-scale information so that the image is not too grainy, the contrast is not too high, and the image is not too hazy and soft. Essentially, the DR is increased when images are of a high quality (softens the images and increases the gray scale), and DR is decreased when image quality is poor (enhances the strongest echoes, eliminates the weaker signals, and reduces background noise). Harmonic imaging has really revolutionized 2D imaging by significantly reducing background noise and improving endocardial border definition. In particular, harmonic imaging with intravenous contrast imaging increases the measurement accuracy of the left ventricular ejection fraction. , Furthermore, in intravenous contrast echocardiographic studies, harmonic imaging enhances the ability to detect injected contrast and improve the duration of opacification.
Temporal resolution refers to the ability of the ultrasound machine to accurately show the position of moving structures at a particular instant in time. Temporal resolution is discussed in terms of the frame rate so that the higher the frame rate, the better the temporal resolution. High frame rates are desirable in echocardiography because of the dynamic nature of cardiac motion; in addition, high frame rates are required for advanced imaging modalities such as 2D speckle tracking. The frame rate can be increased by decreasing the image depth (increases the pulse repetition frequency [PRF]) and by narrowing sector width (decreases number of scan lines).
Optimizing spectral doppler traces
The most important technical factor that affects the optimal display of spectral Doppler signals and the accuracy of measurements is the alignment between blood flow and the ultrasound beam. When blood flow is parallel to the ultrasound beam, the maximum Doppler shift, and therefore the maximum velocity, is accurately detected. However, when blood flow is not parallel to the ultrasound beam, the Doppler shift is reduced, and therefore the derived velocity will be underestimated. Furthermore, because pressure gradients are derived from the Doppler velocity, the accuracy of gradient measurements also depends on the alignment between blood flow and the ultrasound beam. Therefore blood flow must be interrogated from echocardiographic views in which flow is aligned as parallel to the ultrasound beam as possible, and this often requires the use of multiple views, including off-axis imaging.
Other spectral Doppler controls that can be used to improve Doppler traces include the velocity scale and baseline, wall filters, and sweep speed. In particular, the velocity scale and baseline should be adjusted to ensure that the signal of interest fills the display. Wall filters, which allow the elimination of low velocity signals, should be set sufficiently high to eliminate low velocity signals but not so high that the beginning and end of flow is obscured or ambiguous. The sweep speed, which is the horizontal display rate of the spectral Doppler trace, can affect the accuracy of time-related measurements. Therefore a minimum sweep speed of 100 mm/sec is recommended for the measurement of time, time velocity integrals, and time velocity slopes. Slower sweep speeds are useful for averaging velocities that vary with respiration and for observing respiratory variation related to pathologies such as cardiac tamponade and constrictive pericarditis. Sample volume size is also important for pulsed-wave Doppler assessment ( Fig. 7.2 , A, B ).

Optimizing color doppler images
Key controls that are altered to optimize color Doppler images include color box size and length, color gain, and color velocity scale. The color box width affects the frame rate; increasing the color box width increases the processing time and therefore lowers the frame rate. The color box length affects the color velocity scale, such that a longer color box decreases the PRF and therefore lowers the color velocity scale. Because high frame rates are required and because the maximum color scale is necessary to assess high velocity flow, the color box should be adjusted to visualize the structure in question. In situations in which the color box is underfilled or when velocity flow is low, the color velocity is reduced until the color box is adequately filled ( Fig. 7.3 and Video 7.3, A, B ). Furthermore, the color velocity scale should be decreased when using various parameters such as the flow convergence radius, the vena contracta width, and color area ratios to evaluate the severity of valvular regurgitation.

Color Doppler gain, which adjusts the degree of amplification of received Doppler signals, should be set just below the threshold for background speckling or noise. The color gain should be optimized for every view.
Summary
Although many controls on ultrasound machines will be similar regardless of the manufacturer, it is important to also be aware of the different methods for optimizing images and traces for different ultrasound systems. Therefore the echocardiographer should be familiar with the various technicalities of the machine that they operate. Details regarding the effective manipulation of these controls for image optimization can usually be found in the user manual or may be explained by an applications specialist.
Transthoracic Echocardiography Tomographic Views
- Wendy Tsang, MD, MS
- Roberto M. Lang, MD
- Itzhak Kronzon, MD
- Roberto M. Lang, MD
This section describes the main set of echocardiographic images, which should be acquired for standardization and facilitation of image interpretation. Standard examination images are acquired from several transducer positions on the chest wall. Each window, angulation, and rotation of the transducer about its axis enables acquisition of several tomographic echocardiographic views. The sonographer acquiring the images may sit on either the left or the right side of the patient and scan with either the right or left hand, respectively. Nomenclature of the transthoracic views is based on three orthogonal planes: long axis, short axis, and four chamber. The views not encompassed by this nomenclature are named by the anatomic structures they visualize. Two-dimensional (2D) gray-scale images are typically acquired first, and then, while maintaining the image plane, color Doppler images are superimposed.
Parasternal window: long-axis, right ventricular inflow and outflow, and short-axis views
The parasternal long-axis view is typically the first image acquired in a transthoracic study ( Fig. 8.1 ). A high-depth image should be obtained to exclude pleural and pericardial effusion (see Fig. 8.1 , C ). A low-depth image should be subsequently obtained to assess the cardiac anatomy (see Fig. 8.1 , D ). From this view, the left atrium, left ventricle, right ventricular (RV) outflow tract, and aortic root are visualized. The right and noncoronary cusps of the aortic valve are seen, as well as the anterior and posterior mitral valve leaflets. The mitral valve and aortic valve should be inspected and interrogated with color Doppler to identify the presence of a regurgitant or stenotic valve.

Measurements obtained from this view include the linear left atrial anteroposterior dimension. It is measured from the trailing edge of the aortic root to the leading edge of the left atrium at end-systole, when the left atrium is largest. Also from this view, M-mode measurements of the aortic root are obtained, although measurements from 2D images are preferred. More commonly, left ventricular (LV) measurements are also obtained. The 2D targeted M-mode cursor should be placed at the level of the papillary muscle tips, oriented perpendicular to the LV walls. Left ventricular internal dimensions are measured at end-diastole (LVID d ) and end-systole (LVID s ). The leading edge to leading edge convention should be used. , From these measurements the fractional shortening can be computed as:
Intraventricular septal (IVS) and posterior wall thicknesses (PWT) (see Fig. 8.1 , D ) are measured at end-diastole. LV mass can be estimated using the formula:
where PWTd is posterior wall thickness at end-diastole and IVSd is interventricular septal wall thickness at end-diastole.
After the parasternal long-axis view, the right ventricular inflow view is obtained by tilting the end of the transducer toward the patient’s head (see Fig. 8.1 , E ). This allows the right atrium, right ventricle, and tricuspid valve to come into view. This view is primarily used for visualizing the anterior and posterior tricuspid valve leaflets and to determine the presence of tricuspid valve regurgitation with color Doppler. In contrast, by tilting the transducer toward the patient’s feet, the right ventricular outflow tract may be viewed (see Fig. 8.1 , F ). From this perspective, the pulmonic valve and the bifurcation of the pulmonary artery are visualized.
Lastly, by rotating the transducer 90 degrees, the parasternal short-axis views are obtained. With angulation of the transducer superiorly, structures at the aortic valve level are imaged ( Fig. 8.2 ). These structures include cusps of the aortic valve, pulmonary artery, pulmonic valve, RV outflow tract, septal and anterior leaflets of the tricuspid valve, right atrium, left atrium, and interatrial septum. By tilting the transducer anteriorly, the RV outflow tract can be seen (see Fig. 8.2 , B ). The mitral valve can be inspected by returning to the short-axis view of the aortic valve and then directing the transducer inferiorly (see Fig. 8.2 , C ). Continued inferior angulation of the transducer allows acquisition of multiple short-axis cuts of the left ventricle from base to apex, allowing assessment of overall LV performance, wall motion, and papillary muscle position (see Fig. 8.2 , D, E ).

Transthoracic apical window
The apical window is typically best imaged with the subject in a shallow left lateral decubitus position with the transducer placed at the apical cardiac impulse and then adjusted to obtain an optimal image ( Fig. 8.3 , A ). Care should be taken to prevent foreshortening of the left ventricle. From this transducer position, all four cardiac chambers should be well visualized (see Fig. 8.3 , B ). This view allows excellent Doppler interrogation of the mitral and tricuspid valves as well as the pulmonary veins. Anterior angulation of the transducer, while in the four-chamber view, allows the aortic valve to come into plane (see Fig. 8.3 , C ).

After acquiring the apical four-chamber view, the transducer is rotated counterclockwise approximately 60 degrees to obtain the apical two-chamber view, and then an additional 60 degrees to obtain the apical three-chamber view (see Fig. 8.3 , D, E ). The two-chamber view allows evaluation of the anterior and inferior walls of the left ventricle as well as color Doppler evaluation of the mitral valve. The apical three-chamber view is similar to the parasternal long-axis view, wherein the anteroseptal and inferolateral walls can be assessed. Color Doppler evaluation of the mitral and aortic valves should be repeated in the apical three-chamber view.
Subcostal window
Subcostal images are obtained with the subject supine, often with the knees bent to relax the abdomen. From this window, visualization of all four cardiac chambers is possible ( Fig. 8.4 ). This view is particularly helpful for evaluating the pericardium, RV free wall thickness, and the interatrial septum. A short-axis image of the left ventricle obtained from the subcostal view can provide images comparable with those obtained from the parasternal short-axis views. Because the subcostal short-axis images are acquired at greater depth, they are typically only recorded if the parasternal images are inadequate. Finally, from this view the inferior vena cava (IVC) and hepatic veins should be visualized (see Fig. 8.4 ). One additional structure that should be scanned routinely from the subcostal view is the abdominal aorta. Pulsed-wave Doppler images obtained in this view are also helpful for examining flow patterns of the abdominal aorta.

Transthoracic suprasternal window
With the patient supine and the neck hyperextended, the transducer is placed in the suprasternal notch. This allows visualization of the ascending aorta, aortic arch, and proximal descending aorta in the long axis and the right pulmonary artery in the short axis ( Fig. 8.5 ). Continuous-wave Doppler recordings from this transducer position can be used to measure the maximum velocity across a coarctation site. Also, pulsed-wave Doppler can be used to document the flow patterns of the proximal thoracic descending aorta.

Off-axis views
The views described previously can be acquired in the majority of patients. Causes for suboptimal images include the patient’s body habitus, inability of the patient to be positioned properly, congenital heart disease, patients’ postsurgical status, and variability in patients’ anatomy. One approach to these patients is to use nontraditional imaging windows such as the right parasternal position or off-axis views. Labeling the site from where images were obtained is important, especially in patients who require serial studies. In addition, off-axis views play a role in demonstrating findings such as pleural or pericardial effusions.
M-Mode Echocardiography
- Itzhak Kronzon, MD
- Gerard P. Aurigemma, MD
Historically, M-mode (motion mode) was the first effective modality for the ultrasonic evaluation of the heart. M-mode echocardiography provides an ice pick, one-dimensional (depth only) view of the heart. , The ultrasound echoes reflected from the various cardiac interfaces are represented as dots, and their intensities by brightness (B-mode). With the sweep of the screen (or the recording paper), the location of each interface is represented by a line, which provides information about its temporal location ( Fig. 9.1 ). The two-dimensional (2D) appearance of the tracing is the result of presenting depth (expressed as the up-down dimension of the tracing) and width (left to right dimension, which expresses time).
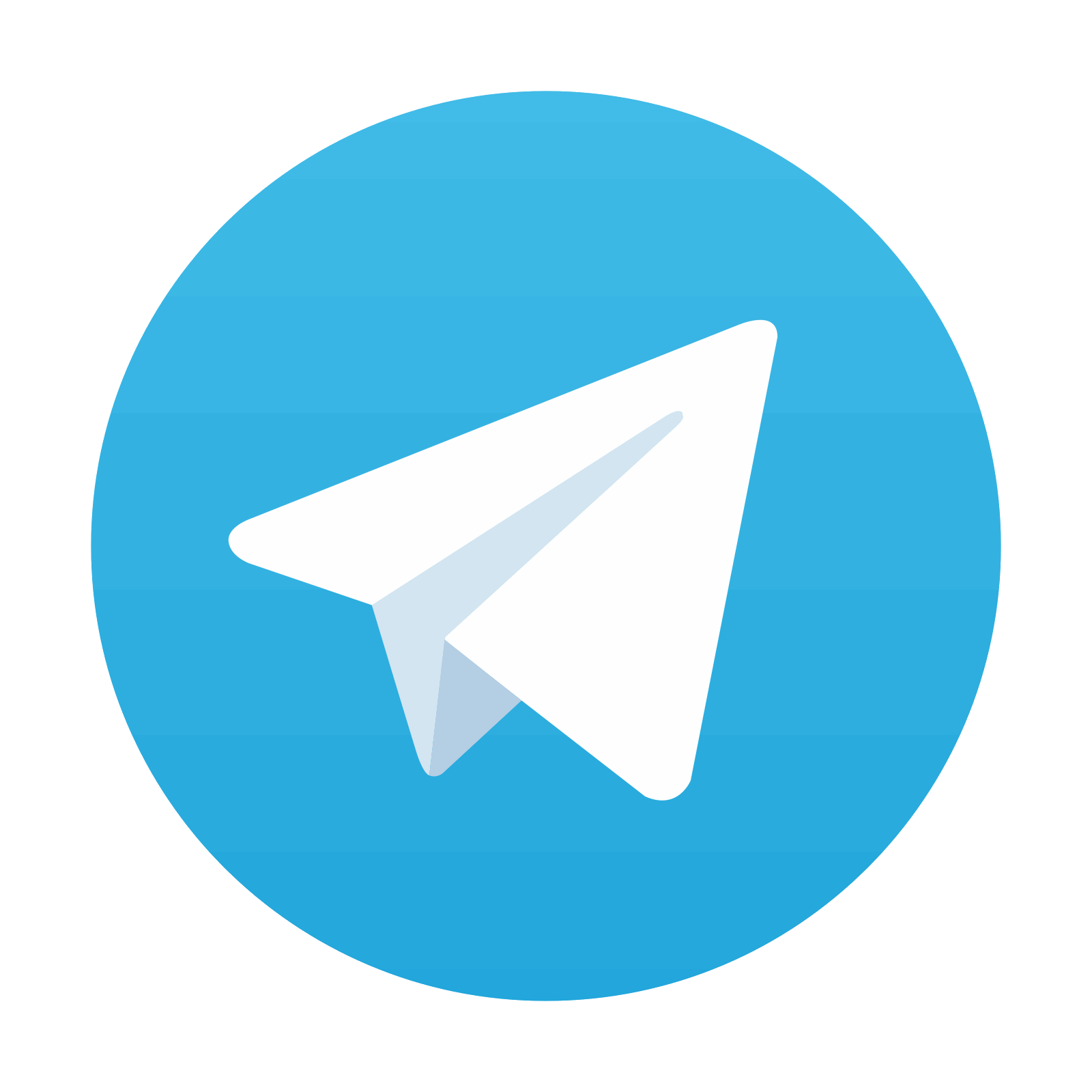
Stay updated, free articles. Join our Telegram channel

Full access? Get Clinical Tree
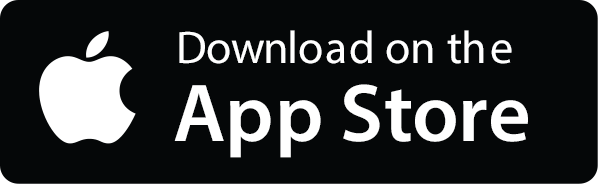
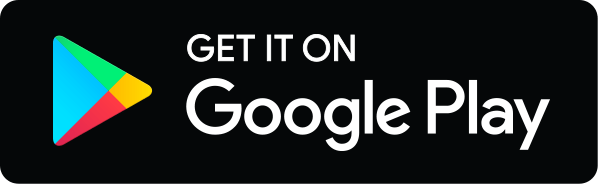
