Predictors, clinical impact, and management strategies for conduction abnormalities after transcatheter aortic valve replacement: an updated review
- Department of Cardiology, The Second Affiliated Hospital of Nanchang University, Nanchang, China
Transcatheter aortic valve replacement (TAVR) has increasingly become a safe, feasible, and widely accepted alternative surgical treatment for patients with severe symptomatic aortic stenosis. However, the incidence of conduction abnormalities associated with TAVR, including left bundle branch block (LBBB) and high-degree atrioventricular block (HAVB), remains high and is often correlated with risk factors such as the severity of valvular calcification, preexisting conditions in patients, and procedural factors. The existing research results on the impact of post-TAVR conduction abnormalities and permanent pacemaker (PPM) requirements on prognosis, including all-cause mortality and rehospitalization, remain contradictory, with varied management strategies for post-TAVR conduction system diseases across different institutions. This review integrates the latest research in the field, offering a comprehensive discussion of the mechanisms, risk factors, consequences, and management of post-TAVR conduction abnormalities. This study provides insights into optimizing patient prognosis and explores the potential of novel strategies, such as conduction system pacing, to minimize the risk of adverse clinical outcomes.
1 Introduction
Aortic stenosis (AS), a prevalent cardiac valve disease, is becoming increasingly common due to the increase in life expectancy and the growth of the elderly population (1, 2). Over the past two decades, transcatheter aortic valve replacement (TAVR) has emerged as a minimally invasive alternative, especially for patients with severe symptomatic AS and a high risk for surgical aortic valve replacement (SAVR) (1, 3, 4). Over time, improved surgical techniques, cumulative experience, updated transcatheter heart valve (THV) designs, and better patient selection have collectively enhanced surgical safety, effectively contributing to reductions in perioperative mortality and procedural complication rates (5, 6). However, there is still a relatively greater incidence of cardiac conduction abnormalities after TAVR than after SAVR, and this trend differs from the decreasing incidence of other postoperative complications (7, 8). In particular, the two most frequent types are new-onset left bundle branch block (LBBB) and high-grade atrioventricular block (HAVB), which necessitate permanent pacemaker implantation (PPI) (9, 10). The incidence of conduction abnormalities and PPI post-TAVR generally varies somewhat depending on the THV system used, and we exemplify in Table 1 the incidence of new PPI within 30 days after TAVR using the different THVs. Some of the current findings have tended to show that balloon-expandable valves are more effective in reducing the incidence of post-TAVR conduction abnormalities and permanent pacemaker implantation events than self-expandable valves, but the differences between generations of THVs are not significant in this regard (19, 28–30).
Extensive research indicates that TAVR-treated patients often experience positive clinical outcomes, a factor anticipated to facilitate the adoption of the procedure for more diverse patient groups, including younger individuals and those with low perioperative risks (8, 31–33), but the potential adverse effects of conduction abnormalities and long-term right ventricular pacing post-TAVR might mitigate its superiority. The aim of this review is to dissect the latest insights into the mechanisms, predictors, and types of conduction abnormalities that occur after TAVR, along with their clinical impacts and management tactics to elucidate the relationships and developmental trends between TAVR and new-onset conduction abnormalities and to ultimately provide more refined management approaches for TAVR.
2 The anatomical mechanisms underlying the occurrence of conduction abnormalities after TAVR
With the progressive popularization and advancement of TAVR, researchers begun to increasingly focus on accurately visualizing the anatomy of the aortic valve complex, specifically the cardiac conduction system. This emphasis is crucial for effectively preventing the occurrence of new conduction abnormalities after TAVR in the future. Comprehending the anatomical interplay between the aortic valve complex and the cardiac conduction system is also foundational in the study of new-onset conduction irregularities following TAVR.
The aortic valve, a tri-leaflet structure devoid of vascular supply, is attached to the aortic root via a fibrous annulus. Based on the position of the leaflets relative to the coronary orifices, they are identified as the right coronary cusp (RC), left coronary cusp (LC), and noncoronary cusp (NC) (Figure 1A) (34). Pertaining to the conduction system critical for TAVR, the atrioventricular node (AVN) is located in the right atrium, predominantly at the base of the atrial septum, and is typically identifiable by Koch's triangle, which is composed of Todaro's tendon, the coronary sinus orifice, and the insertion point of the tricuspid valve septal leaflet (36–38). Notably, the vertex of Koch's triangle is proximal to the cardiac central fibrous body. The central fibrous body, comprising the membranous septum (MS) and the right fibrous triangle formed by thickening of the end of the fiber continuity region, separates the subaortic area of the left ventricle from the right atrium and ventricle. It is also a region of the heart where the membranous septum, atrioventricular valve and aortic valve are connected by fibrous continuity (39). The AVN then tapers anteriorly, disengaging from the atrial myocardium and traversing through to the right fibrous triangle, forming the His bundle or atrioventricular bundle. The his bundle ascends inclined anteriorly and superiorly from the posteroinferior to the anterior, crossing the MS and emerging near the aortic root. Then, this bundle forms a bifurcation near the interventricular ridge, with the left branch forming the left bundle branch (LBB) at the interleaflet triangle base between the noncoronary and right coronary sinuses, and the right branch forming the right bundle branch (RBB) just below the medial papillary muscle of the tricuspid valve in the right ventricle (40).
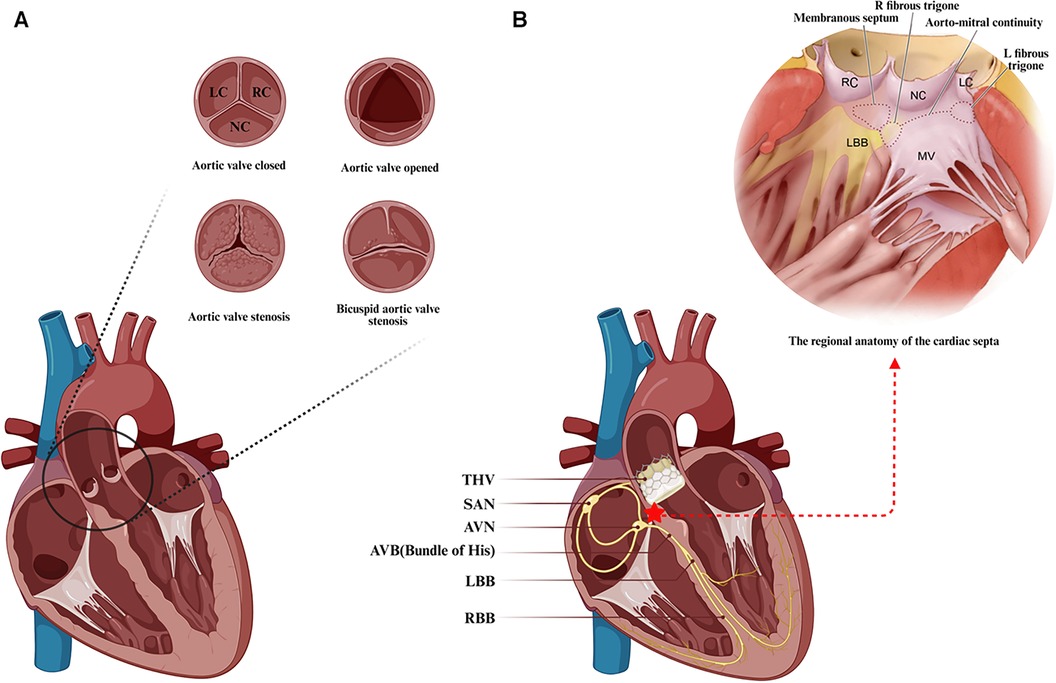
Figure 1. Anatomical features associated with transcatheter aortic valve replacement. (A) The anatomy and frequent types of aortic stenosis. (B) Positional relationship between the transcatheter aortic valve landing zone and the atrioventricular conduction system. Anatomical schematic of the membranous septal region in the heart from Samuel J et al. (35). LC, left coronary cusp; RC, right coronary cusp; NC, noncoronary cusp; SAN, sinoatrial node; AVN, atrioventricular node; AVB, atrioventricular bundle; LBB, left bundle branch; RBB, right bundle branch; THV, transcatheter aortic valve. (Created with BioRender.com).
Due to the close anatomical proximity of the conduction system to the distal landing zone of the transcatheter heart valve (THV) located in the left ventricular outflow tract (LVOT), particularly the direct exposure of the His bundle after crossing the membranous septum near the aortic root, the His bundle and the originating portion of the LBB are closely associated with the base of the NC and RC leaflets' apical triangle at the aortic valve (Figure 1B). Technical maneuvers near the aortic root, such as fragmenting the calcified valve or implanting a prosthesis, can pressure surrounding tissues, potentially causing edema, inflammation, ischemia, or hematoma, and consequently new conduction abnormalities, which are a concern during TAVR (38, 41–43). There is correlative evidence linking TAVR-induced conduction abnormalities to calcific aortic stenosis: calcium deposits may also affect the nearby conduction system, and aortic valve stenosis-associated left ventricular (LV) dysfunction may increase the risk of advanced AV block and LBBB (37). Furthermore, the majority of new conduction abnormalities were observed to occur in association with a series of operations prior to the actual implantation of the THV, mainly in the form of postoperative new-onset LBBB. The frequency of these conduction abnormalities during TAVR varies, with the highest likelihood occurring during balloon preexpansion, followed by THV expansion, THV positioning, balloon catheter positioning, and wire crossing of the aortic valve (41, 44). New conduction abnormalities are likely to occur not only during the procedure but also some time afterward, and late-onset new conduction abnormalities warrant further investigation to fully understand their underlying mechanisms (45).
Interestingly, there is notable individual variability in the anatomy of aortic valves and conduction systems. The bileaflet aortic valve (BAV) is the most common aortic valve malformation, often leading to aortic stenosis in younger patients, while unileaflet and quadricuspid aortic valves are rarer anatomical variants (46). Hence, investigating the relevance of conduction abnormalities after TAVR in patients with BAV stenosis is crucial. Anatomical aspects such as the anteroposterior relation of the AVN to Koch's triangle apex, the length of the penetrating portion of the His bundle, the length of the membranous septum, and variations in the position of the AVB and the proximal branching of the LBB show interindividual differences. These interindividual anatomical variations are likely significant factors affecting susceptibility to conduction system injuries. A previous study of 115 autopsies in elderly patients revealed that nearly half of the patients had relatively right-sided AV bundles, approximately 30% had comparatively left-sided AV bundles, and approximately 20% had AV bundles traveling under the septum below the endocardium. In the latter two variants, the location of the AV bundle is more exposed and vulnerable to damage from external forces, especially in patients with a shorter membranous septum, who are at greater risk for conduction abnormalities after TAVR (36, 40).
3 Predictors of conduction abnormalities after TAVR
3.1 Electrocardiogram-related factors
Numerous studies evaluating the predictors of conduction abnormalities or PPI after TAVR have identified preoperative right bundle branch block (RBBB) as a primary risk factor (47, 48). Additionally first-degree AVB and new-onset LBBB have also been revealed to be significant predictors. Research by Gonska et al. (49) and Keßler et al. (50) independently demonstrated that baseline RBBB and first-degree AVB were predictors of new PPI necessity post-TAVR. In a prospective study, Pavlicek et al. (51) reported that postoperative new-onset LBBB (OR: 15.72; 95% CI: 3.05–81.03; p = 0.001) and preoperative bundle-branch block (OR: 11.64; 95% CI: 2.87-47.20; p = 0.001) were independent predictors of high-grade atrioventricular block (HAVB) necessitating PPI after TAVR. Data from a Chinese TAVR cohort indicated that new-onset LBBB (p = 0.004) and lead I T-wave elevation (p = 0.016) were the primary predictors of PPI (52). It was also suggested in some studies that ΔPR (the difference between the postoperative and preoperative PR intervals) was probably an independent predictor of delayed late conduction disorders (≥48 h) after TAVR (53), with ΔPR > 40 ms linked notably to an increased risk of PPI (54). In particular, a study on delayed total atrioventricular block (DT-AVB) morbidity and potential predictors after TAVR highlighted that intraoperative HV interval prolongation (OR: 1.07; 95% CI: 1.02–1.14; p = 0.015) and PQ interval prolongation between the next day post-TAVR and baseline (OR: 1.04; 95% CI: 1.01–1.09; p = 0.032) might predict the occurrence of DT-AVB (55). Recently, Yagel et al. reported that the R-wave amplitude in the V1 lead of the baseline ECG appeared to predict the onset of HAVB in patients with new-onset LBBB post-TAVR, and patients with HAVB requiring PPI had a significantly lower baseline R-wave amplitude in the V1 lead than those who did not develop HAVB (0.029 ± 0.04 mV vs. 0.11 ± 0.14 mV, p = 0.0316) (56).
3.2 Anatomy-related factors
Asymmetrical calcification patterns of the aortic valve, as well as heightened calcium burdens in the left ventricular outflow tract or the valve implantation area, are pivotal anatomical factors for predicting PPI necessity post-TAVR. Fujita et al. investigated the impact of aortic valve calcium distribution on atrioventricular block necessitating PPI post-TAVR and revealed that increased calcium in the left coronary cusp (LCC) was an independent risk factor for PPI after TAVR, particularly in patients with LCC calcium loads exceeding 209 mm3 (16.7% vs. 2.6%; p = 0.003) (57). They hypothesized that this may be related to the significant shift of the balloon and THV with calcium loading from the LCC to the conjunction between the RCC and LCC during the operation. However, Mauri et al. reported that a heightened calcium volume in the LVOT below the LCC or in the RCC was independently correlated with the PPI requirement after TAVR (LVOT LC: p = 0.016) (LVOT RC: p = 0.005) (58). Another study from the Cedars-Sinai Heart Institute identified elevated calcium volume in the NCC-DLZ area (the region of valve implantation below the NCC) as the most relevant predictor for PPI after TAVR with the Sapien 3 valve (OR: 1.04; 95% CI: 1.02–1.06; p < 0.001) (59). Moreover, the membranous septum length has been recognized as a key predictor of post-TAVR conduction abnormalities, with shorter MS lengths seemingly correlating with greater PPI needs (60) Notably, a multicenter study revealed that shorter MS length was an independent predictor of PPI requirements after TAVR for various THV devices, excluding Accurate-THV (Sapien 3: OR: 0.87; 95% CI: 0.79–0.99; p < 0.01) (Evolut: OR: 0.91; 95% CI: 0.84–0.98; p = 0.03) (61). However, there is currently no evidence of any statistically meaningful effect of THV type on the relationship between MS length and new PPI. Severe mitral annular calcification (MAC) (62), the tapered LVOT (63), the large aortic valve area (AVA) (61), and the high ratio of postoperative to preoperative AVA (64) have also been identified as independent predictors of new PPI after TAVR for the first time in recent studies and are strongly associated with conduction abnormalities after TAVR.
3.3 Procedure-related factors
Intraoperative TAVR procedures such as guidewire insertion, balloon dilatation, and valve implantation may inflict direct mechanical injury to the surrounding tissues of the aortic root or induce changes such as inflammation, edema, ischemia, and necrosis. These alterations can compress or impair the conduction system. While no clinical indicators directly assess the extent of intraoperative mechanical injury, other potentially procedure-related risk factors include the THV diameter, THV implantation depth, and THV type. A large meta-analysis revealed that a larger prosthetic valve size (MD: 1.52%; p < 0.05) and lower implantation depth (MD: 0.95 mm; p < 0.05) were procedural predictors of PPI after TAVR (65). Almeida's et al. retrospective study confirmed that a lower THV implantation depth (OR: 1.16; 95% CI: 1.01–1.33; p = 0.035) was an independent predictor of conduction abnormalities after TAVR (66). A larger THV diameter/LVOT diameter was also found to significantly correlate with higher PPI rates after TAVR (67). However, some studies have suggested that both THV implantation depth and oversize are not consistently independent predictors of PPI necessity after TAVR (49). Recently, the differences between membranous septum length and THV implantation depth (ΔMSID) (68) and valve recapture (69) have been shown to predict the onset of post-TAVR conduction system disease, with a ΔMSID < 0 being deemed to be the strongest and most unique modifiable predictor (68). Further studies have shown that both the coronal ΔMSID measured on preoperative CT and the infra-annular ΔMSID measured on postoperative angiography are variable predictors of conduction abnormalities after TAVR, with the coronal ΔMSID being more predictive (95.9% vs. 87.2%; p = 0.002) (70). Additionally, the use of self-expanding THV has been identified as a predictive factor, with patients treated with BEV possessing seemingly lower new PPI rates than those receiving SEV (61, 71).
Therefore, it seems plausible that the emergence of conduction abnormalities post-TAVR is attributable to a synergistic interplay among patient-specific anatomical characteristics, baseline cardiac electrophysiological features, and procedural interventions. These factors can be broadly categorized into variable and nonvariable elements, and common predictors that have been identified are shown in Figure 2. However, owing to the indications for PPI or the different study methodologies used at different centers, the details of these predictors remain subject to debate. For instance, the cutoffs for membranous septum length or implantation depth may differ based on the study institution, leading to inconsistent findings. In the future, researchers could effectively reduce the risk of the need for postoperative PPI in patients who undergo TAVR by refining the implantation technique or updating the design of next-generation THVs without increasing the risk of perivalvular leakage or coronary artery obstruction.
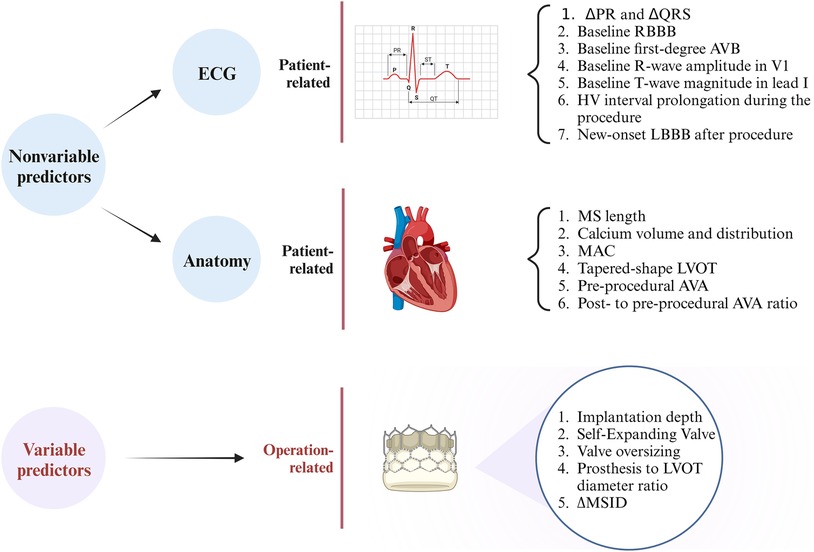
Figure 2. Summary of the predictors of conduction abnormalities after transcatheter aortic valve replacement. ΔPR, difference between postprocedural and preprocedural PR lengths; ΔQRS, difference between postprocedural and preprocedural QRS lengths; RBBB, right bundle branch block; LBBB, left bundle branch block; AVB, atrioventricular block; MS, membranous septum; MAC, mitral annular calcification; LVOT, left ventricular outflow tract; AVA, aortic valve area; ΔMSID, difference between the membranous septum length and implantation depth. (Created with BioRender.com).
4 The types and effects of conduction abnormalities after TAVR
4.1 Impact of new-onset left bundle branch conduction block after TAVR
As the left bundle branch is proximate to the interleaflet triangle between the right coronary and noncoronary cusps, new-onset LBBB is frequently observed after TAVR. However, its clinical impact shows heterogeneity across studies, influenced by its statistical power, follow-up duration, definition of new-onset LBBB, and patient demographics at various institutions (Table 2) (72–74). Additionally, new-onset LBBB has been found to vary dynamically, with some cases resolving over time, while others may persist or progress to HAVB requiring PPI (80–83). This variability may be related to LBBB pathogenesis, individual aortic root anatomy, extent of tissue damage, and recovery rate (84, 85). Short-term follow-up at the University of Minnesota Medical Center revealed no significant difference in all-cause mortality or the PPI rates at one year between patients with and without new-onset LBBB after TAVR, although the former group had a lower LVEF at one year (51.8 ± 11.2 vs. 57.6 ± 8.3; p = 0.002) and higher rates of PPI during the index hospitalization (14.9% vs. 0%; p < 0.001) (73). Chamandi et al. also observed that new-onset persistent left bundle branch block (NOP-LBBB) after TAVR was not correlated with increased all-cause mortality, cardiovascular mortality, or heart failure rehospitalization rates but potentially increased the risk of PPI (15.5% vs. 5.4%; adjusted HR: 2.45; 95% CI: 1.37–4.38; p = 0.002) and worsened left ventricular function (Δ1.9 ± 0.6% vs. Δ1.4 ± 0.9%; p < 0.001 for LVEF over time between groups) (74). Conversely, some studies revealed an association between new-onset LBBB after TAVR and increased all-cause mortality, PPI rates, and cardiac-related hospitalization rates during the follow-up period (86, 87). Intriguingly, Nazif et al., in the PARTNER trial in 2013, reported that new-onset LBBB after TAVR may be independent of 30-day or 1-year all-cause mortality and cardiovascular mortality but was significantly associated with higher repeat hospitalization and PPI rates (p = 0.01) and reduced LVEF improvement (p = 0.02) at 1 year after discharge (75). However, the findings of their later PARTNER II trial in 2019 indicated that new-onset LBBB after TAVR not only correlated with more repeat hospitalization, more PPI, and declining left ventricular function during the 2-year period after discharge but was also linked to increased all-cause mortality (adjusted HR: 1.98; 95% CI: 1.33–2.96; p < 0.001) and cardiovascular mortality (adjusted HR: 2.66; 95% CI: 1.67–4.24; p < 0.001) over two years (72). Figure 3 summarizes the clinical impact of new-onset LBBB after TAVR.
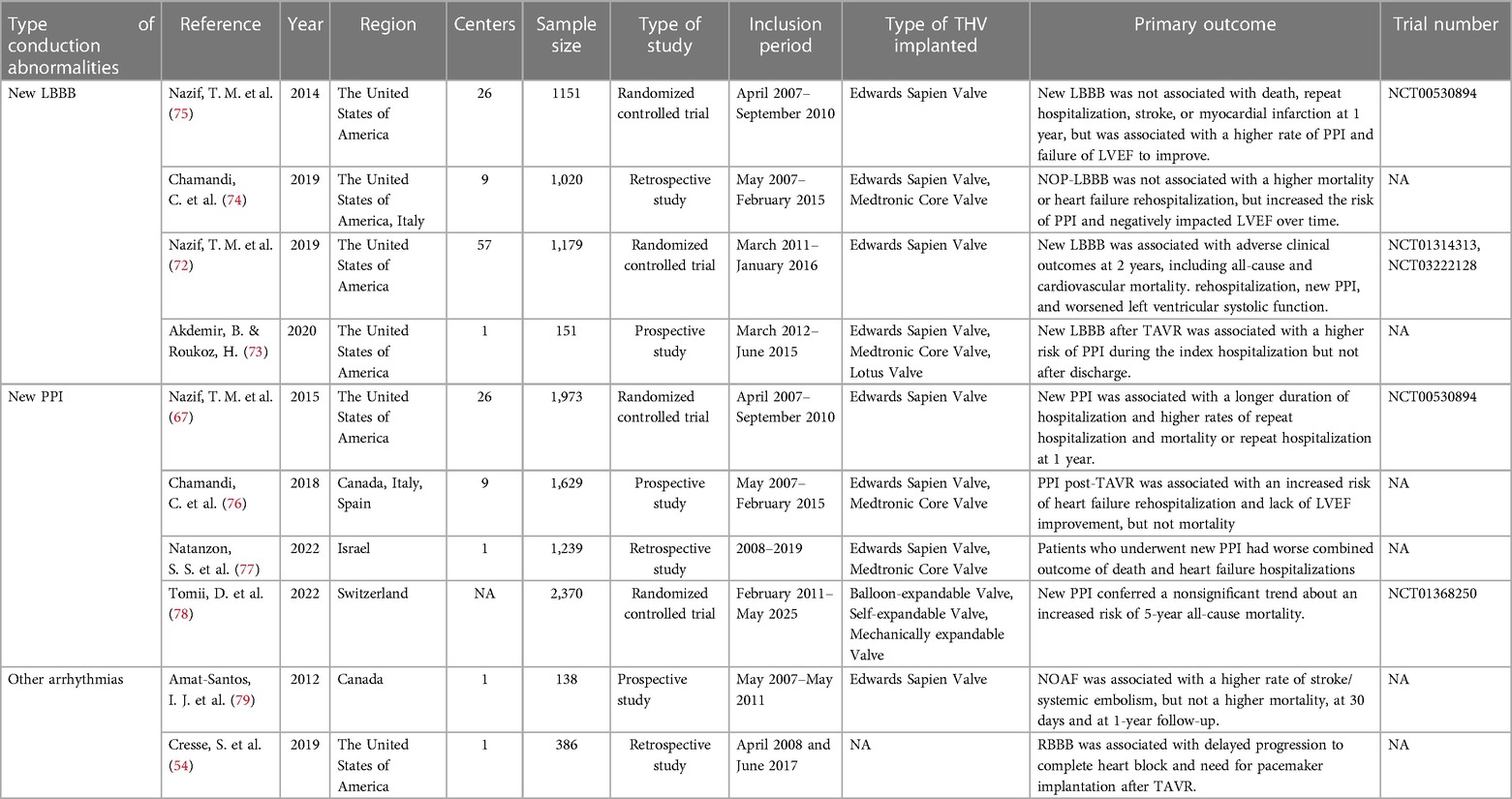
Table 2. Prognostic impact of different conduction abnormalities and permanent pacemaker implantation after TAVR.
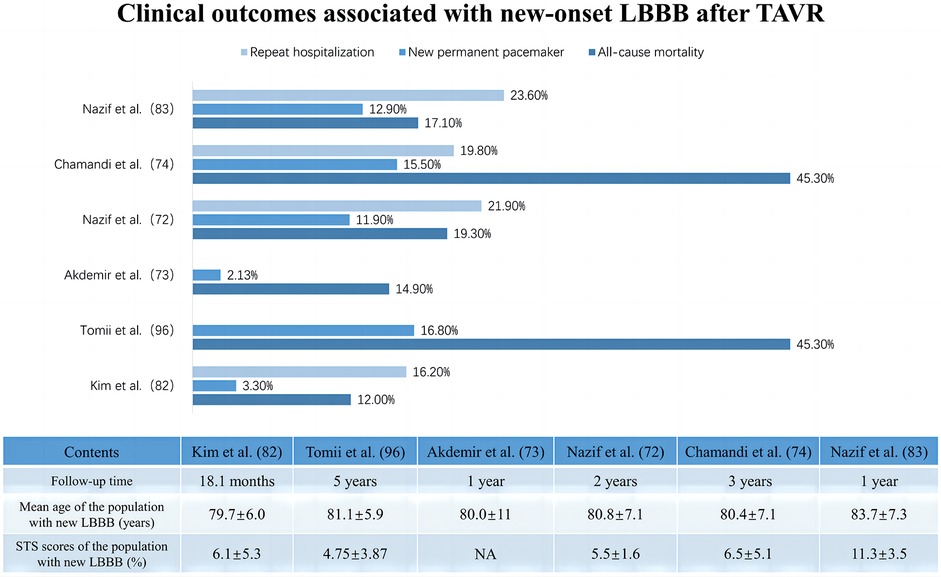
Figure 3. Clinical impact of new onset of left bundle branch block after transcatheter aortic valve replacement.
LBBB has long been recognized as a risk factor for mortality in the general population and in patients with various cardiovascular diseases. Most studies have revealed that the increased mortality risk in patients with LBBB may be attributed to induced ventricular dyssynchronous contractions, septal motion abnormalities, myocardial fibrotic microscopic remodeling, or mitral regurgitation, which leads to left ventricular dysfunction and ultimately to adverse cardiovascular events such as clinical heart failure (85, 88, 89). For patients with new-onset LBBB after TAVR, relevant studies exploring its impact on mortality and perioperative PPI requirements have yielded conflicting results. Compared to resolvable LBBB, new-onset persistent LBBB seems to result in higher PPI rates and limited LVEF improvement, possibly due to its own detrimental mechanisms and progression to HAVB necessitating PPI (75, 90, 91). This highlights the need for further research to substantiate these findings. Importantly, most existing studies have focused on high-risk or surgically untreatable patients who have a greater overall risk of mortality and multiple competing risk factors for death. This population's characteristics could obscure the realistic clinical impact of new-onset LBBB after TAVR (72).
4.2 Impact of the high degree of atrioventricular block and permanent pacemaker implantation after TAVR
Relative to LBBB, HAVB after TAVR is often closely linked to the need for PPI, with approximately 15% of patients developing HAVB within 30 days after TAVR and subsequently requiring PPI (92). Cardiac conduction abnormalities after TAVR are generally transient and influenced by the diversity of patient anatomy, the type of valve implanted, and the depth of implantation (93). Persistent HAVB has been proposed as one of the definitive indications for PPI (94). In a prospective study, Hochstadt et al. defined HAVB (second-degree or complete AVB), any symptomatic bradycardia (SB), and LBBB with HV > 65 ms as the main indications for needing PPI after TAVR (95). A similar study concluded that late second- or third-degree AVB inability to subside, sinus node dysfunction, and symptomatic bradycardia were indicated to require PPI after TAVR (74). It has even been suggested that persistent HAVB lasting more than 24 h is a recognized adaptation for PPI after TAVR (77). While the criteria for PPI after TAVR vary across institutions, most researchers suggest that the indications for PPI be strictly limited to those described in the international guidelines posed by the ACCF/AHA/HRS, which advocate for PPI in patients who present with persistent HAVB or sinus node dysfunction accompanied by symptomatic bradycardia after TAVR but not for those with isolated new-onset LBBB (96–98).
Most studies have focused on the prognostic impact of HAVB-associated permanent pacemaker implantation rather than HAVB alone. The available results on the clinical impacts of PPI after TAVR show a paradoxical trend. Some studies have shown that post-TAVR PPI may not be associated with increased adverse events, including all-cause mortality and cardiovascular death (78). A multicenter cohort study concerning the long-term outcomes of PPI after TAVR reported similar overall or cardiovascular mortality rates at 4 years between patients with and without PPI after TAVR but a significantly greater rehospitalization risk for heart failure (22.4% vs. 16.1%; adjusted HR: 1.42; 95% CI: 1.06–1.89; p = 0.019) and a trend toward lower LVEF (p = 0.051 for between-group LVEF change) in PPI patients, especially those with preexisting LV dysfunction (p = 0.005 for between-group LVEF change) (76). These findings are echoed in a meta-analysis by Mohananey et al. (99). Conversely, a meta-analysis enrolling 83,082 patients reported that increased long-term all-cause mortality (RR: 1.18; 95% CI: 1.09–1.28; p < 0.0001), heightened risk of heart failure rehospitalization (RR: 1.42; 95% CI: 1.18–1.71; p = 0.0002) and reduced LVEF improvement (95% CI: −4.88–2.26; p < 0.00001) were associated with PPI after TAVR (100). Nazif et al. (67) and Natanzon et al. (77) also concluded that patients who underwent PPI after TAVR seemed to have a clearly higher risk of 1-year mortality and heart failure hospitalization or repeat hospitalization than patients who did not undergo PPI. Figure 4 summarizes the clinical impact of PPI after TAVR.
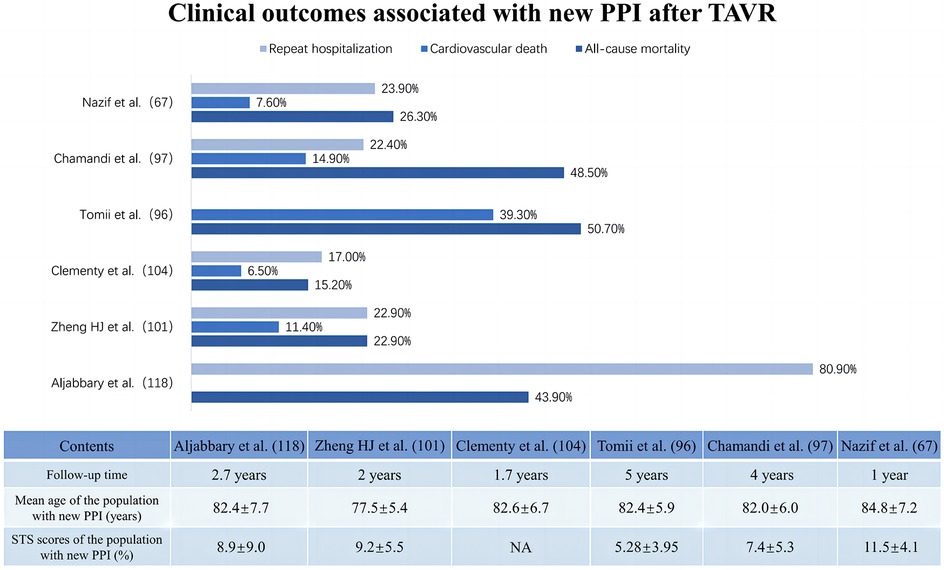
Figure 4. Clinical impact of new permanent pacemaker implantation after transcatheter aortic valve replacement.
It is unclear whether new PPI after TAVR is associated with poorer prognostic outcomes or simply indicative of patients at elevated risk. While the PPI effectively protects against severe atrioventricular block and life-threatening insidious chronic arrhythmias (101), early evidence indicates a potential link between persistent high-frequency right ventricular pacing (RVP) and an increased risk of cardiovascular death and heart failure rehospitalization (102). Bruno's multicenter study with a 6-month follow-up on patients who underwent PPI after TAVR revealed that those with RVP ≥ 40% had significantly higher risks of cardiovascular death and hospitalization for heart failure than patients with RVP < 40% (103). The underlying detrimental mechanism might involve the slow and myocardial depolarization caused by RVP, with asynchronous electrical and mechanical activity between ventricles. This asynchrony of electrical and mechanical activities results in delayed left ventricular activation compared to that of the right ventricle, potentially leading to left ventricular systolic dysfunction, diminished ejection fraction, and even negative left ventricular remodeling (104). Long-term complications associated with permanent pacemakers, especially infections, as well as the possibility that left ventricular electromechanical activation dyssynchrony due to right ventricular pacing may induce or exacerbate mitral and tricuspid regurgitation, which may all be reasons why PPI is associated with increased mortality rates (105–107).
4.3 Impact of other arrhythmias
New-onset atrial fibrillation (NOAF) is also a relatively common arrhythmia after TAVR, with prevalence rates ranging between 6.8% and 32% (79, 108–110). Some researchers have suggested that patients with NOAF after TAVR have increased risks of death, heart failure hospitalization, stroke, and hemorrhage compared to patients without AF or with preexisting AF (109, 110). Nontransfemoral artery access was considered the most powerful influencing factor for NOAF. However, Amat-Santos et al. reported no significant differences in overall or cardiac mortality between patients with and without NOAF after TAVR, although NOAF patients had higher incidences of stroke (13.6% vs. 3.2%) and systemic embolic events (15.9% vs. 3.2%) (79). Although the relationship between NOAF and mortality after TAVR is not consistently clear, NOAF frequently emerges as a predictor of adverse outcomes such as hemorrhage and ischemic cardiovascular events (108). Patients who develop NOAF after TAVR are typically older, have more comorbidities, poorer cardiac function, and larger left atrial volumes and are more likely to have undergone balloon dilatation or nonfemoral arterial access, particularly via the transapical route (109, 111). This mechanism is probably ascribed to pericardial and epicardial disruption (112) or an inflammatory response such as trauma (113). Interestingly, compared with single-chamber ventricular pacing (VVI), preoperative permanent pacemaker implantation for TAVR might reduce the incidence of postoperative NOAF, with single-chamber atrial pacing (AAI) or dual-chamber atrial pacing (DDD) associated with a lower AF risk, possibly due to synchronized atrial and ventricular pacing preventing atrial remodeling and inhibiting ectopic atrial foci that lead to AF (114). According to the Class IIa recommendations of the AHA/ACC/HRS guidelines on oral anticoagulants and amiodarone, antithrombotic management is critical for patients with NOAF after TAVR, emphasizing the need for tailored strategies to ensure safer outcomes (115). Notably, antithrombotic regimens for patients treated with TAVR across institutions and specific guidelines for the clinical application of NOAF management after TAVR are lacking. The potential benefits of alternatives such as left auricular occlusion in patients unsuitable for standard anticoagulant treatment remain to be further explored (109).
Although less attention has been given in existing studies to other arrhythmic events after TAVR, such as right bundle branch block (RBBB), left anterior fascicular block (LAFB), intraventricular conduction delay (IVCD), and severe bradycardia, these occurrences are clinically significant. As highlighted by Wang et al. in a case report, other new-onset conduction abnormalities beyond AVB or LBBB after TAVR pose risks of potentially progressing to severe conduction blocks or indicating significant damage to the cardiac conduction system, prompting the development of adverse events (116). Cresse et al., in a single-center retrospective study, reported that patients with new-onset RBBB after TAVR had a higher incidence of complete atrioventricular block (CHB) and PPI requirement than those without new-onset RBBB (OR: 13.2; 95% CI: 4.18–41.70; p < 0.0001) (54). Moreover, anatomic studies suggest that owing to early anatomic separation of the right and left bundle branches, the proximal branch of the right bundle branch may emerge first to the left of the interventricular septum, which is susceptible to valve-related damage (117). Sometimes, only right bundle branch damage can be detected after TAVR but may actually be accompanied by damage to the left bundle branch. Therefore, clinicians should be aware of this phenomenon in practice and prioritize the occurrence of arrhythmic events other than LBBB and AVB. Further research is needed to elucidate the relationship between these arrhythmias and the progression to HAVB or the increased rate of PPI.
5 Management of conduction abnormalities after TAVR
With the increasing prevalence of TAVR, cardiac conduction abnormalities following the procedure remain a concerning and persistently addressed complication (118). Telemetric ECG monitoring and temporary pacemaker implantation are widely accepted as clinical management strategies for post-TAVR conduction abnormalities. Telemetric monitoring can swiftly detect abnormal cardiac electrical activities, temporary pacemakers serve a provisional substitution role, and permanent pacemaker implantation is often advocated as a subsequent corrective measure (101). According to a class I recommendation of the 2013 ESC Guidelines on cardiac pacing and cardiac resynchronization therapy, clinical observation of up to 7 days is required for patients who develop high or complete AV block after TAVR to assess whether the rhythm disturbance is transient and resolves. However, in case of complete AV block with low rate of escape rhythm this observation period can be shortened since remission is unlikely. If apparent bradyarrhythmia does not resolve during the recommended observation period after TAVR, permanent cardiac pacing should be performed (96). Although the 2013 ESC guidelines address pacing after TAVR, there is no in-depth discussion of this topic. Later, in the 2018 ACC/AHA/HRS Guideline on the Evaluation and Management of Patients With Bradycardia and Cardiac Conduction Delay, regarding the management of conduction abnormalities after TAVR, the Class I recommendation is that permanent pacing before discharge is suggested for patients who develop new AV block with symptoms or hemodynamic instability after TAVR. The Class IIa recommendation is that careful monitoring of bradycardia should be recommended in patients with new persistent bundle branch block after TAVR. The Class IIb recommendation is that PPM implantation should be considered in patients who develop a new persistent LBBB after TAVR (94).
Recently, some studies have found that patients without LBBB, RBBB, or first-degree AVB and those with atrial fibrillation but no BBB or bradycardia after TAVR were less likely to develop delayed HAVB, thus they have been recommended to remove the temporary pacemaker early in the postoperative period and to avoid prolonged telemetric monitoring so as to minimize complications related to temporary pacemaker leads and to shorten hospitalization time. However, patients with conduction abnormalities such as LBBB or RBBB after TAVR are at a high risk of progressing to HAVB requiring PPI, and continuous telemetric ECG monitoring is essential (119, 120). Despite these approaches, uncertainties persist regarding the practical application of electrophysiological studies, the appropriate duration for telemetric monitoring, regulations surrounding temporary pacemakers, and decisions related to the selection and optimal timing of PPI, all of which require further comprehensive research to improve patient prognosis and refine management protocols after TAVR.
5.1 Telemetric ECG monitoring
Despite the current debates over the optimal duration for post-TAVR telemetric ECG monitoring, studies have recommended monitoring for up to 72 h to detect the occurrence of late malignant arrhythmic events (121). Gils et al. suggested extended monitoring for at least 6 days for patients with normal baseline conduction but persistent postoperative QRS prolongation (122). Some researchers advocate for up to 30 days of monitoring for a comprehensive assessment of arrhythmic events and timely intervention (81). Toggweiler et al., however, proposed that patients without any conduction abnormalities or with a stable ECG for 48 h after TAVR may be considered for earlier and safer hospital discharge (119). The debate on the appropriate duration of telemetric ECG monitoring after TAVR continues. Regardless of the presence of baseline or new conduction abnormalities after TAVR, routine daily 12-lead ECGs during hospitalization are recommended. Ultimately, the management of post-TAVR patients and decisions on hospital stay durations should prioritize safety without compromising care quality.
5.2 Pacemaker-related management
With the increasing incidence of post-TAVR conduction abnormalities, there is growing interest in research on the prognostic impact of permanent pacemaker implantation in TAVR patients. Despite its inherently low operative risk, the PPI tends to have less favorable clinical outcomes, particularly in patients with preexisting left ventricular dysfunction (11, 123). Some researchers have suggested the adoption of specialized algorithms to minimize the number of VPs, the exploration of additional physiological pacing patterns that are appropriate for patients with persistent CHB after TAVR, or the use of advanced pacing strategies such as the use of dual chamber pacemakers (DDDRs) and replacement strategies such as cardiac resynchronization therapy (CRT). These approaches might enhance overall outcomes for patients who are receiving PPI after TAVR (124, 125). However, while DDDR and CRT may reduce the risk of mortality and improve heart failure outcomes in patients with LBBB or severe LV systolic dysfunction, their benefits are not as pronounced in patients with preserved LV systolic function (126).
Recently, His-Purkinje conduction system pacing (HPCSP) has emerged as a more physiological pacing method that involves directly stimulating the His bundle or left bundle branch area. This technique drives stimulation down the physiological conduction pathway and promotes ventricular electrical activity synchronization, encompassing His bundle pacing (HBP) and left bundle branch pacing (LBBP) (42, 127). Compared to RVP, the HPCSP has been proven to be effective in shortening QRS intervals and improving cardiac function in TAVR patients (128). Specifically, HBP has been found to be potentially related to the normalization of QRS intervals and the progressive reestablishment of normal ventricular activation patterns in patients with new-onset LBBB after TAVR (129). However, compared with RVP and LBBP, HBP poses greater challenges in terms of implantation difficulty and pacing parameters. More studies are needed to thoroughly assess the benefits of HBP relative to conventional RVP in the future.
A minimalist approach including left ventricular guidewire rapid pacing, local anesthesia with conscious sedation, radial approach for secondary arterial access and echocardiographically guided vascular access, among others, has also recently been proposed to reduce the invasiveness and shorten the duration of the TAVR procedure for early discharge (130). Among these, left ventricular guidewire rapid pacing is considered a safer and more reliable alternative to temporary pacing, with lower complication rates than implantation of a temporary pacemaker in the right ventricle (131). There is a proposed algorithm for temporary pacing in TAVR proposed by some researchers, left ventricular guidewire rapid pacing is recommended as a priority for all patients with a previously implanted permanent pacemaker or without the history of PPI and high-risk factors such as baseline RBBB. However, for patients without a prior history of PPI but with a high risk for conduction disturbance, an upfront right ventricular pacing strategy via internal jugular (IJ) vein access may be safer because of the potential for CHB to be induced by left ventricular guidewire placement before establishing the left ventricular pacing circuit (132). Overall, left ventricular guidewire rapid pacing can be performed routinely in most cases, and upfront IJ vein pacing wire placement is considered a reasonable approach even in patients with high-risk features, but additional large randomized trials are needed to estimate the safety and efficacy of LV pacing as well as the cost-effectiveness of LV pacing in TAVR.
5.3 Electrophysiology (ESP)
According to the latest ESC guidelines for cardiac pacing and cardiac resynchronization therapy, patients with new-onset LBBB after TAVR, exhibiting QRS intervals ≥150 ms or PR intervals ≥240 ms, should undergo ambulatory electrocardiographic monitoring or EPS for early risk stratification. This approach aids in reducing the duration of long-term monitoring while providing valuable insights for decision-making and timing of PPI (80, 97). However, the optimal timing for EPS and the best cutoff value for HV intervals remain uncertain. Various HV interval thresholds, such as ≥55 ms (133), ≥65 ms (134), ≥70 ms (135), and the most commonly used ≥75 ms (121), are considered significant predictors of AVB after TAVR. According to the guidelines, placing a temporary pacemaker for 24–48 h in all patients who develop LBBB after TAVR is recommended, with EPS suggested for those exhibiting sustained dynamic changes in PR or QRS intervals within 48 h (101, 136). Although delayed damage to the conduction system may not be detectable on early ECGs, prophylactic implantation of permanent pacemakers in these patients is not universally endorsed.
5.4 Cusp-overlapping projection (COP)
Historically, the standard 3-cusp coplanar projection, in which the 3 coronary cusps are in the same plane at the time of release of the THV, has been the preferred surgical viewing projection for balloon-expandable THV, and the cusp-overlapping projection (COP) is a new technique based on a modification of the classical implantation technique (CIT). Moreover, the COP technique has the potential to become the gold standard for surgically observing projections of self-expanding THVs. Some studies have shown that compared with CIT, the COP technique significantly reduces the risk of PPI after the implantation of self-expanding valves for TAVR without increasing the incidence of adverse outcome events (137, 138). The COP technique offers equal safety and efficacy even in balloon-expandable and mechanically expandable TAVR procedures. Stephan et al. showed that the application of COP significantly reduced the incidence of LBBB after TAVR with repositionable mechanically expandable valves and the rate of PPI after TAVR with balloon-expandable valves compared with the standard TCC prediction (139). These potential advantages of the COP technique in reducing the occurrence of conduction disorders after TAVR may be related to its tendency to provide insights into the right anterior oblique and caudal projections, allowing the aortic annulus and delivery system to be in similar planes, which reduces parallax in the catheterization bands, better displays the left ventricular outflow tract and the aortic root, and provides relative release from the membranous septum, allowing for more precise measurements, better contiguous alignment, and greater THV implantation (138, 140). Although COP technology may provide potential benefits in reducing the PPI rate, data on the impact of COP technology on clinical outcomes are still scarce, and studies with larger samples are needed to assess its efficacy and safety.
6 Conclusion
The persistence of cardiac conduction abnormalities after TAVR remains a significant barrier to its broader application in younger and lower-risk cohorts. Addressing this challenge necessitates comprehensive preoperative assessments encompassing anatomical, electrocardiographic, and surgical risk factors. The intraoperative use of COP technique or vigilance in prosthesis width and implantation depth selection is crucial for better controlling valve positioning and release and minimizing tissue damage surrounding the LVOT, especially in severely calcified aortic valves. Postprocedure, more standardized ambulatory ECG monitoring, short-term temporary pacemaker implementation, timely introduction of electrophysiologic studies, and other measures are vital for those patients who are at high risk of developing HAVB requiring PPI, with careful monitoring of the PR, QRS, and HV intervals to optimally time permanent pacemaker implantation. Alternatively, strategies that may improve the long-term prognosis of patients, such as DDD or HPCSP, may be chosen. Overall, with the expanding indications for TAVR, researchers must continually identify the anatomical locations of the conduction system adjacent to the region of procedural manipulation and learn more about the predictors, developmental trends, prognostic consequences, and optimal management strategies of new conduction abnormalities after TAVR.
Author contributions
QY: Conceptualization, Data curation, Methodology, Project administration, Writing – original draft. QF: Conceptualization, Data curation, Methodology, Writing – original draft. YX: Software, Visualization, Writing – original draft. YW: Funding acquisition, Resources, Supervision, Validation, Writing – review & editing, Writing – original draft.
Funding
The authors declare financial support was received for the research, authorship, and/or publication of this article.
This research was funded by grants from the Jiangxi Provincial Key R&D Program (grant no. 20212BBG71004) and the Science and Technology Research Project of the Education Department (grant no. GJJ190021).
Acknowledgments
We acknowledge BioRender.com for providing the image materials.
Conflict of interest
The authors declare that the research was conducted in the absence of any commercial or financial relationships that could be construed as a potential conflict of interest.
Publisher's note
All claims expressed in this article are solely those of the authors and do not necessarily represent those of their affiliated organizations, or those of the publisher, the editors and the reviewers. Any product that may be evaluated in this article, or claim that may be made by its manufacturer, is not guaranteed or endorsed by the publisher.
References
1. Kanwar A, Thaden JJ, Nkomo VT. Management of patients with aortic valve stenosis. Mayo Clin Proc. (2018) 93(4):488–508. doi: 10.1016/j.mayocp.2018.01.020
2. Thaden JJ, Nkomo VT, Enriquez-Sarano M. The global burden of aortic stenosis. Prog Cardiovasc Dis. (2014) 56(6):565–71. doi: 10.1016/j.pcad.2014.02.006
3. Lindman BR, Clavel MA, Mathieu P, Iung B, Lancellotti P, Otto CM, et al. Calcific aortic stenosis. Nat Rev Dis Primers. (2016) 2:16006. doi: 10.1038/nrdp.2016.6
4. UK TAVI Trial Investigators, Toff WD, Hildick-Smith D, Kovac J, Mullen MJ, Wendler O, et al. Effect of transcatheter aortic valve implantation vs surgical aortic valve replacement on all-cause mortality in patients with aortic stenosis: a randomized clinical trial. JAMA. (2022) 327(19):1875–87. doi: 10.1001/jama.2022.5776
5. Thiele H, Kurz T, Feistritzer HJ, Stachel G, Hartung P, Eitel I, et al. Comparison of newer generation self-expandable vs. balloon-expandable valves in transcatheter aortic valve implantation: the randomized SOLVE-TAVI trial. Eur Heart J. (2020) 41(20):1890–9. doi: 10.1093/eurheartj/ehaa036
6. Tugaoen Z, Nguyen P, Arora S, Vavalle J. The selection of transcatheter heart valves in transcatheter aortic valve replacement. Trends Cardiovasc Med. (2022) 32(8):513–22. doi: 10.1016/j.tcm.2021.10.002
7. Siontis GCM, Overtchouk P, Cahill TJ, Modine T, Prendergast B, Praz F, et al. Transcatheter aortic valve implantation vs. surgical aortic valve replacement for treatment of symptomatic severe aortic stenosis: an updated meta-analysis. Eur Heart J. (2019) 40(38):3143–53. doi: 10.1093/eurheartj/ehz275
8. Kolte D, Vlahakes GJ, Palacios IF, Sakhuja R, Passeri JJ, Inglessis I, et al. Transcatheter versus surgical aortic valve replacement in low-risk patients. J Am Coll Cardiol. (2019) 74(12):1532–40. doi: 10.1016/j.jacc.2019.06.076
9. Auffret V, Puri R, Urena M, Chamandi C, Rodriguez-Gabella T, Philippon F, et al. Conduction disturbances after transcatheter aortic valve replacement: current Status and future perspectives. Circulation. (2017) 136(11):1049–69. doi: 10.1161/CIRCULATIONAHA.117.028352
10. Sammour Y, Krishnaswamy A, Kumar A, Puri R, Tarakji KG, Bazarbashi N, et al. Incidence, predictors, and implications of permanent pacemaker requirement after transcatheter aortic valve replacement. JACC Cardiovasc Interv. (2021) 14(2):115–34. doi: 10.1016/j.jcin.2020.09.063
11. Schymik G, Lefèvre T, Bartorelli AL, Rubino P, Treede H, Walther T, et al. European experience with the second-generation Edwards SAPIEN XT transcatheter heart valve in patients with severe aortic stenosis: 1-year outcomes from the SOURCE XT registry. JACC Cardiovasc Interv. (2015) 8(5):657–69. doi: 10.1016/j.jcin.2014.10.026
12. Pellegrini C, Freißmuth M, Rheude T, Graas D, Mayr NP, Syryca F, et al. Implantation depth of balloon-expandable transcatheter heart valves and risks for permanent pacemaker implantation and midterm adverse events. Catheter Cardiovasc Interv. (2023) 102(7):1301–10. doi: 10.1002/ccd.30870
13. Saia F, Gandolfo C, Palmerini T, Berti S, Doshi SN, Laine M, et al. In-hospital and thirty-day outcomes of the SAPIEN 3 ultra balloon-expandable transcatheter aortic valve: the S3U registry. EuroIntervention. (2020) 15(14):1240–7. doi: 10.4244/EIJ-D-19-00541
14. García-Gómez M, Delgado-Arana JR, Halim J, De Marco F, Trani C, Martin P, et al. Next-generation balloon-expandable myval transcatheter heart valve in low-risk aortic stenosis patients. Catheter Cardiovasc Interv. (2022) 99(3):889–95. doi: 10.1002/ccd.29923
15. Elkoumy A, Jose J, Terkelsen CJ, Nissen H, Gunasekaran S, Abdelshafy M, et al. Safety and efficacy of myval implantation in patients with severe bicuspid aortic valve stenosis-A multicenter real-world experience. J Clin Med. (2022) 11(2):443. doi: 10.3390/jcm11020443
16. Dowling C, Firoozi S, Doyle N, Blackman DJ, Malkin CJ, Cunnington MS, et al. Initial experience of a large, self-expanding, and fully recapturable transcatheter aortic valve: the UK & Ireland Implanters’ registry. Catheter Cardiovasc Interv. (2019) 93(4):751–7. doi: 10.1002/ccd.27934
17. Manoharan G, Grube E, Van Mieghem NM, Brecker S, Fiorina C, Kornowski R, et al. Thirty-day clinical outcomes of the evolut PRO self-expanding transcatheter aortic valve: the international FORWARD PRO study. EuroIntervention. (2020) 16(10):850–7. doi: 10.4244/EIJ-D-20-00279
18. Scotti A, Baggio S, Pagnesi M, Barbanti M, Adamo M, Eitan A, et al. Temporal trends and contemporary outcomes after transcatheter aortic valve replacement with evolut PRO/PRO+ self-expanding valves: insights from the NEOPRO/NEOPRO-2 registries. Circ Cardiovasc Interv. (2023) 16(1):e012538. doi: 10.1161/CIRCINTERVENTIONS.122.012538
19. Costa G, Saia F, Pilgrim T, Abdel-Wahab M, Garot P, Valvo R, et al. Transcatheter aortic valve replacement with the latest-iteration self-expanding or balloon-expandable valves: the multicenter OPERA-TAVI registry. JACC Cardiovasc Interv. (2022) 15(23):2398–407. doi: 10.1016/j.jcin.2022.08.057
20. Zaid S, Attizzani GF, Krishnamoorthy P, Yoon SH, Palma Dallan LA, Chetcuti S, et al. First-in-human multicenter experience of the newest generation supra-annular self-expanding evolut FX TAVR system. JACC Cardiovasc Interv. (2023) 16(13):1626–35. doi: 10.1016/j.jcin.2023.05.004
21. Möllmann H, Walther T, Siqueira D, Diemert P, Treede H, Grube E, et al. Transfemoral TAVI using the self-expanding ACURATE neo prosthesis: one-year outcomes of the multicentre “CE-approval cohort.”. EuroIntervention. (2017) 13(9):e1040–6. doi: 10.4244/EIJ-D-17-00187
22. Möllmann H, Holzhey DM, Hilker M, Toggweiler S, Schäfer U, Treede H, et al. The ACURATE neo2 valve system for transcatheter aortic valve implantation: 30-day and 1-year outcomes. Clin Res Cardiol. (2021) 110(12):1912–20. doi: 10.1007/s00392-021-01882-3
23. Möllmann H, Linke A, Holzhey DM, Walther T, Manoharan G, Schäfer U, et al. Implantation and 30-day follow-up on all 4 valve sizes within the portico transcatheter aortic bioprosthetic family. JACC Cardiovasc Interv. (2017) 10(15):1538–47. doi: 10.1016/j.jcin.2017.05.021
24. Reardon MJ, Chehab B, Smith D, Walton AS, Worthley SG, Manoharan G, et al. 30-day clinical outcomes of a self-expanding transcatheter aortic valve: the international PORTICO NG study. JACC Cardiovasc Interv. (2023) 16(6):681–9. doi: 10.1016/j.jcin.2023.02.002
25. Montone RA, Testa L, Fraccaro C, Montorfano M, Castriota F, Nerla R, et al. Procedural and 30-day clinical outcomes following transcatheter aortic valve replacement with lotus valve: results of the RELEVANT study. Catheter Cardiovasc Interv. (2017) 90(7):1206–11. doi: 10.1002/ccd.26914
26. Meredith Am IT, Walters DL, Dumonteil N, Worthley SG, Tchétché D, Manoharan G, et al. Transcatheter aortic valve replacement for severe symptomatic aortic stenosis using a repositionable valve system: 30-day primary endpoint results from the REPRISE II study. J Am Coll Cardiol. (2014) 64(13):1339–48. doi: 10.1016/j.jacc.2014.05.067
27. Armario X, Rosseel L, Kharbanda R, Khogali S, Abdel-Wahab M, Van Mieghem NM, et al. Transcatheter aortic valve replacement with the LOTUS edge system: early European experience. JACC Cardiovasc Interv. (2021) 14(2):172–81. doi: 10.1016/j.jcin.2020.09.044
28. Forrest JK, Kaple RK, Tang GHL, Yakubov SJ, Nazif TM, Williams MR, et al. Three generations of self-expanding transcatheter aortic valves: a report from the STS/ACC TVT registry. JACC Cardiovasc Interv. (2020) 13(2):170–9. doi: 10.1016/j.jcin.2019.08.035
29. Pagnesi M, Kim WK, Conradi L, Barbanti M, Stefanini GG, Zeus T, et al. Transcatheter aortic valve replacement with next-generation self-expanding devices: a multicenter, retrospective, propensity-matched comparison of evolut PRO versus acurate neo transcatheter heart valves. JACC Cardiovasc Interv. (2019) 12(5):433–43. doi: 10.1016/j.jcin.2018.11.036
30. Rheude T, Pellegrini C, Lutz J, Alvarez-Covarrubias HA, Lahmann AL, Mayr NP, et al. Transcatheter aortic valve replacement with balloon-expandable valves: comparison of SAPIEN 3 ultra versus SAPIEN 3. JACC Cardiovasc Interv. (2020) 13(22):2631–8. doi: 10.1016/j.jcin.2020.07.013
31. Popma JJ, Deeb GM, Yakubov SJ, Mumtaz M, Gada H, O’Hair D, et al. Transcatheter aortic-valve replacement with a self-expanding valve in low-risk patients. N Engl J Med. (2019) 380(18):1706–15. doi: 10.1056/NEJMoa1816885
32. Mack MJ, Leon MB, Thourani VH, Makkar R, Kodali SK, Russo M, et al. Transcatheter aortic-valve replacement with a balloon-expandable valve in low-risk patients. N Engl J Med. (2019) 380(18):1695–705. doi: 10.1056/NEJMoa1814052
33. Leon MB, Mack MJ, Hahn RT, Thourani VH, Makkar R, Kodali SK, et al. Outcomes 2 years after transcatheter aortic valve replacement in patients at low surgical risk. J Am Coll Cardiol. (2021) 77(9):1149–61. doi: 10.1016/j.jacc.2020.12.052
34. Dutta P, James JF, Kazik H, Lincoln J. Genetic and developmental contributors to aortic stenosis. Circ Res. (2021) 128(9):1330–43. doi: 10.1161/CIRCRESAHA.120.317978
35. Asirvatham SJ, Stevenson WG. Editor’s perspective: the interatrial septum. Circ Arrhythm Electrophysiol. (2013) 6(5):e75–6. doi: 10.1161/CIRCEP.113.000956
36. Lee MY, Yeshwant SC, Chava S, Lustgarten DL. Mechanisms of heart block after transcatheter aortic valve replacement—cardiac anatomy, clinical predictors and mechanical factors that contribute to permanent pacemaker implantation. Arrhythm Electrophysiol Rev. (2015) 4(2):81–5. doi: 10.15420/AER.2015.04.02.81
37. Muntané-Carol G, Guimaraes L, Ferreira-Neto AN, Wintzer-Wehekind J, Junquera L, Del Val D, et al. How does new-onset left bundle branch block affect the outcomes of transcatheter aortic valve repair? Expert Rev Med Devices. (2019) 16(7):589–602. doi: 10.1080/17434440.2019.1624161
38. Piazza N, de Jaegere P, Schultz C, Becker AE, Serruys PW, Anderson RH. Anatomy of the aortic valvar complex and its implications for transcatheter implantation of the aortic valve. Circ Cardiovasc Interv. (2008) 1(1):74–81. doi: 10.1161/CIRCINTERVENTIONS.108.780858
39. Barbanti M, Gulino S, Costa G, Tamburino C. Pathophysiology, incidence and predictors of conduction disturbances during transcatheter aortic valve implantation. Expert Rev Med Devices. (2017) 14(2):135–47. doi: 10.1080/17434440.2017.1282819
40. Kawashima T, Sato F. Visualizing anatomical evidences on atrioventricular conduction system for TAVI. Int J Cardiol. (2014) 174(1):1–6. doi: 10.1016/j.ijcard.2014.04.003
41. Chen S, Chau KH, Nazif TM. The incidence and impact of cardiac conduction disturbances after transcatheter aortic valve replacement. Ann Cardiothorac Surg. (2020) 9(6):452–67. doi: 10.21037/acs-2020-av-23
42. Zhang J, Pan Y, Wang B, Fu G. Current opinions on new-onset left bundle branch block after transcatheter aortic valve replacement and the search for physiological pacing. Rev Cardiovasc Med. (2022) 23(3):90. doi: 10.31083/j.rcm2303090
43. Moreno R, Dobarro D, López de Sá E, Prieto M, Morales C, Calvo Orbe L, et al. Cause of complete atrioventricular block after percutaneous aortic valve implantation: insights from a necropsy study. Circulation. (2009) 120(5):e29–30. doi: 10.1161/CIRCULATIONAHA.109.849281
44. van der Boon RM, Nuis RJ, Van Mieghem NM, Jordaens L, Rodés-Cabau J, van Domburg RT, et al. New conduction abnormalities after TAVI–frequency and causes. Nat Rev Cardiol. (2012) 9(8):454–63. doi: 10.1038/nrcardio.2012.58
45. Nuis RJ, Van Mieghem NM, Schultz CJ, Tzikas A, Van der Boon RM, Maugenest AM, et al. Timing and potential mechanisms of new conduction abnormalities during the implantation of the Medtronic CoreValve system in patients with aortic stenosis. Eur Heart J. (2011) 32(16):2067–74. doi: 10.1093/eurheartj/ehr110
46. Siu SC, Silversides CK. Bicuspid aortic valve disease. J Am Coll Cardiol. (2010) 55(25):2789–800. doi: 10.1016/j.jacc.2009.12.068
47. Cahill TJ, Chen M, Hayashida K, Latib A, Modine T, Piazza N, et al. Transcatheter aortic valve implantation: current status and future perspectives. Eur Heart J. (2018) 39(28):2625–34. doi: 10.1093/eurheartj/ehy244
48. Praz F, Siontis GCM, Verma S, Windecker S, Jüni P. Latest evidence on transcatheter aortic valve implantation vs. surgical aortic valve replacement for the treatment of aortic stenosis in high and intermediate-risk patients. Curr Opin Cardiol. (2017) 32(2):117–22. doi: 10.1097/HCO.0000000000000379
49. Gonska B, Seeger J, Keßler M, von Keil A, Rottbauer W, Wöhrle J. Predictors for permanent pacemaker implantation in patients undergoing transfemoral aortic valve implantation with the Edwards Sapien 3 valve. Clin Res Cardiol. (2017) 106(8):590–7. doi: 10.1007/s00392-017-1093-2
50. Keßler M, Gonska B, Seeger J, Rottbauer W, Wöhrle J. Predictors of permanent pacemaker implantation after transfemoral aortic valve implantation with the Lotus valve. Am Heart J. (2017) 192:57–63. doi: 10.1016/j.ahj.2017.07.011
51. Pavlicek V, Mahfoud F, Bubel K, Fries P, Ewen S, Böhm M, et al. Prediction of conduction disturbances in patients undergoing transcatheter aortic valve replacement. Clin Res Cardiol. (2023) 112(5):677–90. doi: 10.1007/s00392-023-02160-0
52. Zhang J, Chi C, Tian S, Zhang S, Liu J. Predictors of permanent pacemaker implantation in patients after transcatheter aortic valve replacement in a Chinese population. Front Cardiovasc Med. (2021) 8:743257. doi: 10.3389/fcvm.2021.743257
53. Mangieri A, Lanzillo G, Bertoldi L, Jabbour RJ, Regazzoli D, Ancona MB, et al. Predictors of advanced conduction disturbances requiring a late (≥48 H) permanent pacemaker following transcatheter aortic valve replacement. JACC Cardiovasc Interv. (2018) 11(15):1519–26. doi: 10.1016/j.jcin.2018.06.014
54. Cresse S, Eisenberg T, Alfonso C, Cohen MG, DeMarchena E, Williams D, et al. Cardiac conduction abnormalities associated with pacemaker implantation after transcatheter aortic valve replacement. Pacing Clin Electrophysiol. (2019) 42(7):846–52. doi: 10.1111/pace.13695
55. Reiter C, Lambert T, Kellermair J, Blessberger H, Fellner A, Nahler A, et al. Delayed total atrioventricular block after transcatheter aortic valve replacement assessed by implantable loop recorders. JACC Cardiovasc Interv. (2021) 14(24):2723–32. doi: 10.1016/j.jcin.2021.09.003
56. Yagel O, Belhassen B, Planer D, Amir O, Elbaz-Greener G. The R-wave amplitude in V1 on baseline electrocardiogram correlates with the occurrence of high-degree atrioventricular block following left bundle branch block after transcatheter aortic valve replacement. Europace. (2023) 25(5):euad066. doi: 10.1093/europace/euad066
57. Fujita B, Kütting M, Seiffert M, Scholtz S, Egron S, Prashovikj E, et al. Calcium distribution patterns of the aortic valve as a risk factor for the need of permanent pacemaker implantation after transcatheter aortic valve implantation. Eur Heart J Cardiovasc Imaging. (2016) 17(12):1385–93. doi: 10.1093/ehjci/jev343
58. Mauri V, Reimann A, Stern D, Scherner M, Kuhn E, Rudolph V, et al. Predictors of permanent pacemaker implantation after transcatheter aortic valve replacement with the SAPIEN 3. JACC Cardiovasc Interv. (2016) 9(21):2200–9. doi: 10.1016/j.jcin.2016.08.034
59. Maeno Y, Abramowitz Y, Kawamori H, Kazuno Y, Kubo S, Takahashi N, et al. A highly predictive risk model for pacemaker implantation after TAVR. JACC Cardiovasc Imaging. (2017) 10(10 Pt A):1139–47. doi: 10.1016/j.jcmg.2016.11.020
60. Abdelshafy M, Elkoumy A, Elzomor H, Abdelghani M, Campbell R, Kennedy C, et al. Predictors of conduction disturbances requiring new permanent pacemaker implantation following transcatheter aortic valve implantation using the evolut series. J Clin Med. (2023) 12(14):4835. doi: 10.3390/jcm12144835
61. Hokken TW, Muhemin M, Okuno T, Veulemans V, Lopes BB, Beneduce A, et al. Impact of membranous septum length on pacemaker need with different transcatheter aortic valve replacement systems: the INTERSECT registry. J Cardiovasc Comput Tomogr. (2022) 16(6):524–30. doi: 10.1016/j.jcct.2022.07.003
62. Abramowitz Y, Kazuno Y, Chakravarty T, Kawamori H, Maeno Y, Anderson D, et al. Concomitant mitral annular calcification and severe aortic stenosis: prevalence, characteristics and outcome following transcatheter aortic valve replacement. Eur Heart J. (2017) 38(16):1194–203. doi: 10.1093/eurheartj/ehw594
63. Ishizu K, Murakami N, Morinaga T, Hayashi M, Isotani A, Arai Y, et al. Impact of tapered-shape left ventricular outflow tract on pacemaker rate after transcatheter aortic valve replacement. Heart Vessels. (2022) 37(6):1055–65. doi: 10.1007/s00380-021-01999-5
64. Qi Y, Lin X, Pan W, Zhang X, Ding Y, Chen S, et al. A prediction model for permanent pacemaker implantation after transcatheter aortic valve replacement. Eur J Med Res. (2023) 28(1):262. doi: 10.1186/s40001-023-01237-w
65. Maier O, Piayda K, Afzal S, Polzin A, Westenfeld R, Jung C, et al. Computed tomography derived predictors of permanent pacemaker implantation after transcatheter aortic valve replacement: a meta-analysis. Catheter Cardiovasc Interv. (2021) 98(6):E897–907. doi: 10.1002/ccd.29805
66. Almeida JG, Ferreira SM, Fonseca P, Dias T, Guerreiro C, Barbosa AR, et al. Association between implantation depth assessed by computed tomography and new-onset conduction disturbances after transcatheter aortic valve implantation. J Cardiovasc Comput Tomogr. (2017) 11(5):332–7. doi: 10.1016/j.jcct.2017.08.003
67. Nazif TM, Dizon JM, Hahn RT, Xu K, Babaliaros V, Douglas PS, et al. Predictors and clinical outcomes of permanent pacemaker implantation after transcatheter aortic valve replacement: the PARTNER (placement of AoRtic TraNscathetER valves) trial and registry. JACC Cardiovasc Interv. (2015) 8(1 Pt A):60–9. doi: 10.1016/j.jcin.2014.07.022
68. Boonyakiatwattana W, Maneesai A, Chaithiraphan V, Jakrapanichakul D, Sakiyalak P, Chunhamaneewat N, et al. Preprocedural and procedural variables that predict new-onset conduction disturbances after transcatheter aortic valve replacement. BMC Cardiovasc Disord. (2022) 22(1):135. doi: 10.1186/s12872-022-02576-y
69. Castro-Mejía AF, Amat-Santos I, Ortega-Armas ME, Baz JA, Moreno R, Diaz JF, et al. Development of atrioventricular and intraventricular conduction disturbances in patients undergoing transcatheter aortic valve replacement with new generation self-expanding valves: a real world multicenter analysis. Int J Cardiol. (2022) 362:128–36. doi: 10.1016/j.ijcard.2022.05.014
70. Chen YH, Chang HH, Liao TW, Leu HB, Chen IM, Chen PL, et al. Membranous septum length predicts conduction disturbances following transcatheter aortic valve replacement. J Thorac Cardiovasc Surg. (2022) 164(1):42–51.e2. doi: 10.1016/j.jtcvs.2020.07.072
71. Rheude T, Pellegrini C, Allali A, Bleiziffer S, Kim WK, Neuser J, et al. Multicenter comparison of latest-generation balloon-expandable versus self-expanding transcatheter heart valves: ultra versus evolut. Int J Cardiol. (2022) 357:115–20. doi: 10.1016/j.ijcard.2022.03.043
72. Nazif TM, Chen S, George I, Dizon JM, Hahn RT, Crowley A, et al. New-onset left bundle branch block after transcatheter aortic valve replacement is associated with adverse long-term clinical outcomes in intermediate-risk patients: an analysis from the PARTNER II trial. Eur Heart J. (2019) 40(27):2218–27. doi: 10.1093/eurheartj/ehz227
73. Akdemir B, Roukoz H. A single-centre cohort and short-term follow-up of patients who developed persistent new onset left bundle branch block after transcatheter aortic valve replacement. Acta Cardiol. (2020) 75(4):360–5. doi: 10.1080/00015385.2020.1713520
74. Chamandi C, Barbanti M, Munoz-Garcia A, Latib A, Nombela-Franco L, Gutiérrez-Ibanez E, et al. Long-term outcomes in patients with new-onset persistent left bundle branch block following TAVR. JACC Cardiovasc Interv. (2019) 12(12):1175–84. doi: 10.1016/j.jcin.2019.03.025
75. Nazif TM, Williams MR, Hahn RT, Kapadia S, Babaliaros V, Rodés-Cabau J, et al. Clinical implications of new-onset left bundle branch block after transcatheter aortic valve replacement: analysis of the PARTNER experience. Eur Heart J. (2014) 35(24):1599–607. doi: 10.1093/eurheartj/eht376
76. Chamandi C, Barbanti M, Munoz-Garcia A, Latib A, Nombela-Franco L, Gutiérrez-Ibanez E, et al. Long-term outcomes in patients with new permanent pacemaker implantation following transcatheter aortic valve replacement. JACC Cardiovasc Interv. (2018) 11(3):301–10. doi: 10.1016/j.jcin.2017.10.032
77. Natanzon SS, Fardman A, Koren-Morag N, Fefer P, Maor E, Guetta V, et al. Pacing burden and clinical outcomes after transcatheter aortic valve replacement-A real-world registry report. Heart Rhythm. (2022) 19(9):1508–15. doi: 10.1016/j.hrthm.2022.04.030
78. Tomii D, Okuno T, Heg D, Pilgrim T, Windecker S. Long-term outcomes of new-onset conduction abnormalities following transcatheter aortic valve implantation. Arch Cardiovasc Dis. (2022) 115(4):214–24. doi: 10.1016/j.acvd.2022.04.005
79. Amat-Santos IJ, Rodés-Cabau J, Urena M, DeLarochellière R, Doyle D, Bagur R, et al. Incidence, predictive factors, and prognostic value of new-onset atrial fibrillation following transcatheter aortic valve implantation. J Am Coll Cardiol. (2012) 59(2):178–88. doi: 10.1016/j.jacc.2011.09.061
80. Badertscher P, Knecht S, Spies F, Auberson C, Salis M, Jeger RV, et al. Value of periprocedural electrophysiology testing during transcatheter aortic valve replacement for risk stratification of patients with new-onset left bundle-branch block. J Am Heart Assoc. (2022) 11(15):e026239. doi: 10.1161/JAHA.122.026239
81. Rodés-Cabau J, Urena M, Nombela-Franco L, Amat-Santos I, Kleiman N, Munoz-Garcia A, et al. Arrhythmic burden as determined by ambulatory continuous cardiac monitoring in patients with new-onset persistent left bundle branch block following transcatheter aortic valve replacement: the MARE study. JACC Cardiovasc Interv. (2018) 11(15):1495–505. doi: 10.1016/j.jcin.2018.04.016
82. See C, Wang Y, Huang H, Parise H, Yang Y, Tirziu D, et al. Impact of new-onset conduction disturbances following transcatheter aortic valve replacement on outcomes: a single-center study. J Interv Cardiol. (2023) 2023:5390338. doi: 10.1155/2023/5390338
83. Nishiyama T, Tanosaki S, Tanaka M, Yanagisawa R, Yashima F, Kimura T, et al. Predictive factor and clinical consequence of left bundle-branch block after a transcatheter aortic valve implantation. Int J Cardiol. (2017) 227:25–9. doi: 10.1016/j.ijcard.2016.11.063
84. van der Boon RMA, Houthuizen P, Urena M, Poels TT, van Mieghem NM, Brueren GRG, et al. Trends in the occurrence of new conduction abnormalities after transcatheter aortic valve implantation. Catheter Cardiovasc Interv. (2015) 85(5):E144–52. doi: 10.1002/ccd.25765
85. Smiseth OA, Aalen JM. Mechanism of harm from left bundle branch block. Trends Cardiovasc Med. (2019) 29(6):335–42. doi: 10.1016/j.tcm.2018.10.012
86. Regueiro A, Abdul-Jawad Altisent O, Del Trigo M, Campelo-Parada F, Puri R, Urena M, et al. Impact of new-onset left bundle branch block and periprocedural permanent pacemaker implantation on clinical outcomes in patients undergoing transcatheter aortic valve replacement: a systematic review and meta-analysis. Circ Cardiovasc Interv. (2016) 9(5):e003635. doi: 10.1161/CIRCINTERVENTIONS.115.003635
87. Kim K, Ko YG, Shim CY, Ryu J, Lee YJ, Seo J, et al. Impact of new-onset persistent left bundle branch block on reverse cardiac remodeling and clinical outcomes after transcatheter aortic valve replacement. Front Cardiovasc Med. (2022) 9:893878. doi: 10.3389/fcvm.2022.893878
88. Akhtari S, Chuang ML, Salton CJ, Berg S, Kissinger KV, Goddu B, et al. Effect of isolated left bundle-branch block on biventricular volumes and ejection fraction: a cardiovascular magnetic resonance assessment. J Cardiovasc Magn Reson. (2018) 20(1):66. doi: 10.1186/s12968-018-0457-8
89. Zannad F, Huvelle E, Dickstein K, van Veldhuisen DJ, Stellbrink C, Køber L, et al. Left bundle branch block as a risk factor for progression to heart failure. Eur J Heart Fail. (2007) 9(1):7–14. doi: 10.1016/j.ejheart.2006.04.011
90. Nijenhuis VJ, Van Dijk VF, Chaldoupi SM, Balt JC, Ten Berg JM. Severe conduction defects requiring permanent pacemaker implantation in patients with a new-onset left bundle branch block after transcatheter aortic valve implantation. Europace. (2017) 19(6):1015–21. doi: 10.1093/europace/euw174
91. Dolci G, Singh GK, Wang X, van der Kley F, de Weger A, Bootsma M, et al. Effects of left bundle branch block and pacemaker implantation on left ventricular systolic function after transcatheter aortic valve implantation. Am J Cardiol. (2022) 179:64–9. doi: 10.1016/j.amjcard.2022.06.014
92. Bagur R, Rodés-Cabau J, Gurvitch R, Dumont É, Velianou JL, Manazzoni J, et al. Need for permanent pacemaker as a complication of transcatheter aortic valve implantation and surgical aortic valve replacement in elderly patients with severe aortic stenosis and similar baseline electrocardiographic findings. JACC Cardiovasc Interv. (2012) 5(5):540–51. doi: 10.1016/j.jcin.2012.03.004
93. Rubín JM, Avanzas P, del Valle R, Renilla A, Ríos E, Calvo D, et al. Atrioventricular conduction disturbance characterization in transcatheter aortic valve implantation with the CoreValve prosthesis. Circ Cardiovasc Interv. (2011) 4(3):280–6. doi: 10.1161/CIRCINTERVENTIONS.111.961649
94. Kusumoto FM, Schoenfeld MH, Barrett C, Edgerton JR, Ellenbogen KA, Gold MR, et al. 2018 ACC/AHA/HRS guideline on the evaluation and management of patients with bradycardia and cardiac conduction delay: a report of the American college of cardiology/American heart association task force on clinical practice guidelines and the heart rhythm society. J Am Coll Cardiol. (2019) 74(7):e51–156. doi: 10.1016/j.jacc.2018.10.044
95. Hochstadt A, Merdler I, Meridor Y, Schwartz AL, Ingbir M, Ghantous E, et al. Effect of pacemaker implantation after transcatheter aortic valve replacement on long- and mid-term mortality. Heart Rhythm. (2021) 18(2):199–206. doi: 10.1016/j.hrthm.2020.10.013
96. Brignole M, Auricchio A, Baron-Esquivias G, Bordachar P, Boriani G, Breithardt OA, et al. 2013 ESC guidelines on cardiac pacing and cardiac resynchronization therapy: the task force on cardiac pacing and resynchronization therapy of the European society of cardiology (ESC). Developed in collaboration with the European heart rhythm association (EHRA). Eur Heart J. (2013) 34(29):2281–329. doi: 10.1093/eurheartj/eht150
97. Glikson M, Nielsen JC, Kronborg MB, Michowitz Y, Auricchio A, Barbash IM, et al. 2021 ESC guidelines on cardiac pacing and cardiac resynchronization therapy. Eur Heart J. (2021) 42(35):3427–520. doi: 10.1093/eurheartj/ehab364
98. Epstein AE, DiMarco JP, Ellenbogen KA, Estes NAM, Freedman RA, Gettes LS, et al. 2012 ACCF/AHA/HRS focused update incorporated into the ACCF/AHA/HRS 2008 guidelines for device-based therapy of cardiac rhythm abnormalities: a report of the American college of cardiology foundation/American heart association task force on practice guidelines and the heart rhythm society. J Am Coll Cardiol. (2013) 61(3):e6–75. doi: 10.1016/j.jacc.2012.11.007
99. Mohananey D, Jobanputra Y, Kumar A, Krishnaswamy A, Mick S, White JM, et al. Clinical and echocardiographic outcomes following permanent pacemaker implantation after transcatheter aortic valve replacement: meta-analysis and meta-regression. Circ Cardiovasc Interv. (2017) 10(7):e005046. doi: 10.1161/CIRCINTERVENTIONS.117.005046
100. Xu S, Zhang E, Qian Z, Sun J, Zou F, Wang Y, et al. Mid- to long-term clinical and echocardiographic effects of post-procedural permanent pacemaker implantation after transcatheter aortic valve replacement: a systematic review and meta-analysis. Front Cardiovasc Med. (2022) 9:911234. doi: 10.3389/fcvm.2022.911234
101. Rodés-Cabau J, Ellenbogen KA, Krahn AD, Latib A, Mack M, Mittal S, et al. Management of conduction disturbances associated with transcatheter aortic valve replacement: JACC scientific expert panel. J Am Coll Cardiol. (2019) 74(8):1086–106. doi: 10.1016/j.jacc.2019.07.014
102. Zheng HJ, Yan CJ, Lin DQ, Cheng YB, Yu SJ, Li J, et al. Prognostic impact of new permanent pacemaker implantation following transcatheter aortic valve replacement. Catheter Cardiovasc Interv. (2023) 102(4):743–50. doi: 10.1002/ccd.30788
103. Bruno F, Munoz Pousa I, Saia F, Vaira MP, Baldi E, Leone PP, et al. Impact of right ventricular pacing in patients with TAVR undergoing permanent pacemaker implantation. JACC Cardiovasc Interv. (2023) 16(9):1081–91. doi: 10.1016/j.jcin.2023.02.003
104. Tops LF, Schalij MJ, Bax JJ. The effects of right ventricular apical pacing on ventricular function and dyssynchrony implications for therapy. J Am Coll Cardiol. (2009) 54(9):764–76. doi: 10.1016/j.jacc.2009.06.006
105. Clementy N, Bisson A, Bodin A, Herbert J, Lacour T, Etienne CS, et al. Outcomes associated with pacemaker implantation following transcatheter aortic valve replacement: a nationwide cohort study. Heart Rhythm. (2021) 18(12):2027–32. doi: 10.1016/j.hrthm.2021.06.1175
106. Fanari Z, Hammami S, Hammami MB, Hammami S, Shuraih M. The effects of right ventricular apical pacing with transvenous pacemaker and implantable cardioverter defibrillator on mitral and tricuspid regurgitation. J Electrocardiol. (2015) 48(5):791–7. doi: 10.1016/j.jelectrocard.2015.07.002
107. Clémenty N, Fernandes J, Carion PL, de Léotoing L, Lamarsalle L, Wilquin-Bequet F, et al. Pacemaker complications and costs: a nationwide economic study. J Med Econ. (2019) 22(11):1171–8. doi: 10.1080/13696998.2019.1652186
108. Tarantini G, Mojoli M, Windecker S, Wendler O, Lefèvre T, Saia F, et al. Prevalence and impact of atrial fibrillation in patients with severe aortic stenosis undergoing transcatheter aortic valve replacement: an analysis from the SOURCE XT prospective multicenter registry. JACC Cardiovasc Interv. (2016) 9(9):937–46. doi: 10.1016/j.jcin.2016.01.037
109. Mentias A, Saad M, Girotra S, Desai M, Elbadawi A, Briasoulis A, et al. Impact of pre-existing and new-onset atrial fibrillation on outcomes after transcatheter aortic valve replacement. JACC Cardiovasc Interv. (2019) 12(21):2119–29. doi: 10.1016/j.jcin.2019.06.019
110. Vora AN, Dai D, Matsuoka R, Harrison JK, Hughes GC, Sherwood MW, et al. Incidence, management, and associated clinical outcomes of new-onset atrial fibrillation following transcatheter aortic valve replacement: an analysis from the STS/ACC TVT registry. JACC Cardiovasc Interv. (2018) 11(17):1746–56. doi: 10.1016/j.jcin.2018.05.042
111. Shahim B, Malaisrie SC, George I, Thourani VH, Biviano AB, Russo M, et al. Postoperative atrial fibrillation or flutter following transcatheter or surgical aortic valve replacement: pARTNER 3 trial. JACC Cardiovasc Interv. (2021) 14(14):1565–74. doi: 10.1016/j.jcin.2021.05.026
112. Tanawuttiwat T, O’Neill BP, Cohen MG, Chinthakanan O, Heldman AW, Martinez CA, et al. New-onset atrial fibrillation after aortic valve replacement: comparison of transfemoral, transapical, transaortic, and surgical approaches. J Am Coll Cardiol. (2014) 63(15):1510–9. doi: 10.1016/j.jacc.2013.11.046
113. Engelmann MDM, Svendsen JH. Inflammation in the genesis and perpetuation of atrial fibrillation. Eur Heart J. (2005) 26(20):2083–92. doi: 10.1093/eurheartj/ehi350
114. Sparks PB, Mond HG, Vohra JK, Jayaprakash S, Kalman JM. Electrical remodeling of the atria following loss of atrioventricular synchrony: a long-term study in humans. Circulation. (1999) 100(18):1894–900. doi: 10.1161/01.CIR.100.18.1894
115. January CT, Wann LS, Alpert JS, Calkins H, Cigarroa JE, Cleveland JC, et al. 2014 AHA/ACC/HRS guideline for the management of patients with atrial fibrillation: a report of the American college of cardiology/American heart association task force on practice guidelines and the heart rhythm society. Circulation. (2014) 130(23):e199–267. doi: 10.1161/CIR.0000000000000041
116. Wang D, Li H, Ye H, Jiang L. Outcomes of non-left bundle branch block conduction abnormalities after transcatheter aortic valve replacement. Pacing Clin Electrophysiol. (2021) 44(12):2124–6. doi: 10.1111/pace.14353
117. Wu S, Su L, Zheng R, Xu L, Huang W. New-onset intrinsic and paced QRS morphology of right bundle branch block pattern after atrioventricular nodal ablation: longitudinal dissociation or anatomical bifurcation? J Cardiovasc Electrophysiol. (2020) 31(5):1218–21. doi: 10.1111/jce.14469
118. Aljabbary T, Qiu F, Masih S, Fang J, Elbaz-Greener G, Austin PC, et al. Association of clinical and economic outcomes with permanent pacemaker implantation after transcatheter aortic valve replacement. JAMA Netw Open. (2018) 1(1):e180088. doi: 10.1001/jamanetworkopen.2018.0088
119. Toggweiler S, Stortecky S, Holy E, Zuk K, Cuculi F, Nietlispach F, et al. The electrocardiogram after transcatheter aortic valve replacement determines the risk for post-procedural high-degree AV block and the need for telemetry monitoring. JACC Cardiovasc Interv. (2016) 9(12):1269–76. doi: 10.1016/j.jcin.2016.03.024
120. Urena M, Rodés-Cabau J. Electrocardiographic monitoring following transcatheter aortic valve replacement: do we need to and for how long? JACC Cardiovasc Interv. (2016) 9(12):1277–9. doi: 10.1016/j.jcin.2016.05.004
121. Badertscher P, Knecht S, Zeljković I, Sticherling C, de Asmundis C, Conte G, et al. Management of conduction disorders after transcatheter aortic valve implantation: results of the EHRA survey. Europace. (2022) 24(7):1179–85. doi: 10.1093/europace/euac027
122. van Gils L, Baart S, Kroon H, Rahhab Z, El Faquir N, Rodriguez Olivares R, et al. Conduction dynamics after transcatheter aortic valve implantation and implications for permanent pacemaker implantation and early discharge: the CONDUCT-study. Europace. (2018) 20(12):1981–8. doi: 10.1093/europace/euy074
123. Tsushima T, Al-Kindi S, Palma Dallan LA, Fares A, Yoon SH, Wheat HL, et al. Clinical impact of right ventricular pacing burden in patients with post-transcatheter aortic valve replacement permanent pacemaker implantation. Europace. (2023) 25(4):1441–50. doi: 10.1093/europace/euad025
124. Pagnesi M, Kim WK, Baggio S, Scotti A, Barbanti M, De Marco F, et al. Incidence, predictors, and prognostic impact of new permanent pacemaker implantation after TAVR with self-expanding valves. JACC Cardiovasc Interv. (2023) 16(16):2004–17. doi: 10.1016/j.jcin.2023.05.020
125. Sweeney MO, Hellkamp AS, Ellenbogen KA, Greenspon AJ, Freedman RA, Lee KL, et al. Adverse effect of ventricular pacing on heart failure and atrial fibrillation among patients with normal baseline QRS duration in a clinical trial of pacemaker therapy for sinus node dysfunction. Circulation. (2003) 107(23):2932–7. doi: 10.1161/01.CIR.0000072769.17295.B1
126. Prinzen FW, Vernooy K, Auricchio A. Cardiac resynchronization therapy: state-of-the-art of current applications, guidelines, ongoing trials, and areas of controversy. Circulation. (2013) 128(22):2407–18. doi: 10.1161/CIRCULATIONAHA.112.000112
127. Vijayaraman P, Bordachar P, Ellenbogen KA. The continued search for physiological pacing: where are we now? J Am Coll Cardiol. (2017) 69(25):3099–114. doi: 10.1016/j.jacc.2017.05.005
128. Niu HX, Liu X, Gu M, Chen X, Cai C, Cai M, et al. Conduction system pacing for post transcatheter aortic valve replacement patients: comparison with right ventricular pacing. Front Cardiovasc Med. (2021) 8:772548. doi: 10.3389/fcvm.2021.772548
129. De Pooter J, Gauthey A, Calle S, Noel A, Kefer J, Marchandise S, et al. Feasibility of his-bundle pacing in patients with conduction disorders following transcatheter aortic valve replacement. J Cardiovasc Electrophysiol. (2020) 31(4):813–21. doi: 10.1111/jce.14371
130. Lefèvre G, Jégou A, Dambrin G, Picard F, Anconina J, Pouzet B, et al. Comparison of TransFemoral transcatheter aortic valve replacement performed with a minimally invasive simplified technique: “FAST” versus a standard approach. J Invasive Cardiol. (2019) 31(10):300–6.
131. Kleczynski P, Dziewierz A, Socha S, Rakowski T, Daniec M, Zawislak B, et al. Direct rapid left ventricular wire pacing during balloon aortic valvuloplasty. J Clin Med. (2020) 9(4):1017. doi: 10.3390/jcm9041017
132. Blusztein D, Raney A, Walsh J, Nazif T, Woods C, Daniels D. Best practices in left ventricular pacing for transcatheter aortic valve replacement. Struct Heart. (2023) 7(6):100213. doi: 10.1016/j.shj.2023.100213
133. Knecht S, Schaer B, Reichlin T, Spies F, Madaffari A, Vischer A, et al. Electrophysiology testing to stratify patients with left bundle branch block after transcatheter aortic valve implantation. J Am Heart Assoc. (2020) 9(5):e014446. doi: 10.1161/JAHA.119.014446
134. Rivard L, Schram G, Asgar A, Khairy P, Andrade JG, Bonan R, et al. Electrocardiographic and electrophysiological predictors of atrioventricular block after transcatheter aortic valve replacement. Heart Rhythm. (2015) 12(2):321–9. doi: 10.1016/j.hrthm.2014.10.023
135. Massoullié G, Ploux S, Souteyrand G, Mondoly P, Pereira B, Amabile N, et al. Incidence and management of atrioventricular conduction disorders in new-onset left bundle branch block after TAVI: a prospective multicenter study. Heart Rhythm. (2023) 20(5):699–706. doi: 10.1016/j.hrthm.2023.01.013
136. Lilly SM, Deshmukh AJ, Epstein AE, Ricciardi MJ, Shreenivas S, Velagapudi P, et al. 2020 ACC expert consensus decision pathway on management of conduction disturbances in patients undergoing transcatheter aortic valve replacement: a report of the American college of cardiology solution set oversight committee. J Am Coll Cardiol. (2020) 76(20):2391–411. doi: 10.1016/j.jacc.2020.08.050
137. Rawish E, Macherey S, Jurczyk D, Pätz T, Jose J, Stiermaier T, et al. Reduction of permanent pacemaker implantation by using the cusp overlap technique in transcatheter aortic valve replacement: a meta-analysis. Clin Res Cardiol. (2023) 112(5):633–44. doi: 10.1007/s00392-022-02150-8
138. Pascual I, Hernández-Vaquero D, Alperi A, Almendarez M, Avanzas P, Kalavrouziotis D, et al. Permanent pacemaker reduction using cusp-overlapping projection in TAVR: a propensity score analysis. JACC Cardiovasc Interv. (2022) 15(2):150–61. doi: 10.1016/j.jcin.2021.10.002
139. Stephan T, Krohn-Grimberghe M, von Lindeiner Genannt von Wildau A, Buck C, Baumhardt M, Mörike J, et al. Cusp-overlap view reduces conduction disturbances and permanent pacemaker implantation after transcatheter aortic valve replacement even with balloon-expandable and mechanically-expandable heart valves. Front Cardiovasc Med. (2023) 10:1269833. doi: 10.3389/fcvm.2023.1269833
Keywords: transcatheter aortic valve replacement, conduction abnormalities, pacemaker implantation, predictors, prognosis, management
Citation: Yu Q, Fu Q, Xia Y and Wu Y (2024) Predictors, clinical impact, and management strategies for conduction abnormalities after transcatheter aortic valve replacement: an updated review. Front. Cardiovasc. Med. 11:1370244. doi: 10.3389/fcvm.2024.1370244
Received: 14 January 2024; Accepted: 27 March 2024;
Published: 8 April 2024.
Edited by:
Julio Garcia, University of Calgary, CanadaReviewed by:
Michela Faggioni, University of Pennsylvania, United StatesFrancesco Cardaioli, University of Padua, Italy
© 2024 Yu, Fu, Xia and Wu. This is an open-access article distributed under the terms of the Creative Commons Attribution License (CC BY). The use, distribution or reproduction in other forums is permitted, provided the original author(s) and the copyright owner(s) are credited and that the original publication in this journal is cited, in accordance with accepted academic practice. No use, distribution or reproduction is permitted which does not comply with these terms.
*Correspondence: Yanqing Wu wuyanqing01@sina.com
†These authors have contributed equally to this work and share first authorship