Phenotypic Clumping Decreases With Flock Richness in Mixed-Species Bird Flocks
- 1Centre for Ecological Sciences, Indian Institute of Science, Bengaluru, India
- 2National Centre for Biological Sciences, Bengaluru, India
Animals that live in groups may experience positive interactions such as cooperative behavior or negative interactions such as competition from group members depending on group size and similarity between individuals. The effect of group size and phenotypic and ecological similarity on group assembly has not been well-studied. Mixed-species flocks are important subsets of bird communities worldwide. We examined associations within these in relation to flock size, to understand rules of flock assembly, in the Western Ghats of India. We examined the relationship between phenotypic clumping and flock richness using four variables—body size, foraging behavior, foraging height and taxonomic relatedness. Using a null model approach, we found that small flocks were more phenotypically clumped for body size than expected by chance; however, phenotypic clumping decreased as flocks increased in size and approached expected phenotypic variation in large flocks. This pattern was not as clear for foraging height and foraging behavior. We then examined a dataset of 55 flock matrices from 24 sites across the world. We found that sites with smaller flocks had higher values of phenotypic clumping for body size and sites with larger flocks were less phenotypically clumped. This relationship was weakly negative for foraging behavior and not statistically significant for taxonomic relatedness. Unlike most single-species groups, participants in mixed-species flocks appear to be able to separate on different axes of trait similarity. They can gain benefits from similarity on one axis while mitigating competition by dissimilarity on others. Consistent with our results, we speculate that flock assembly was deterministic up to a certain point with participants being similar in body size, but larger flocks tended to approach random phenotypic assemblages of species.
Introduction
Group living is widespread in different animal taxa and contexts across the world (Krause and Ruxton, 2002). While many species form single-species groups, mixed-species (i.e., heterospecific) groups are also commonly seen (Goodale et al., 2019). In each case, the drivers of group assembly and group size likely vary. Although there is both theoretical and empirical work on the drivers of group sizes in single-species groups, our understanding of group sizes in a mixed-species context is limited. Increase in group size in single-species groups is likely to lead to competition for resources (Janson and Van Schaik, 1988). Group size in single-species groups is therefore a trade-off between benefits of increasing group size and costs of competition. However, mixed-species groups may allow species to separate on different axes of ecological and morphological similarity (Sridhar and Guttal, 2018). In effect, mixed-species groups may be affected by the same factors as single-species groups but species can differ in the degree of similarity across traits, thus potentially mitigating the effects of competition. Therefore, understanding group assembly is critical to deciphering the processes that drive mixed-species grouping.
In general, negative interactions between species have traditionally dominated theory in community organization (Weiher and Keddy, 1999). Competition (Cody, 1974; Kelt et al., 1995; Koeppel and Wu, 2014; Letten et al., 2017) and predation (Forsman et al., 2001) have been most commonly invoked as drivers of community assembly (Sinclair, 1985; Weiher et al., 2011; Hiltunen and Laakso, 2013). Competitive interactions are known to influence species distribution and coexistence (MacArthur, 1958; Connell, 1961; also reviewed in Schoener, 1974, 1983; Rusterholz, 1981) although evidence for this is not unequivocal (Park and Lloyd, 1955). Predation and parasitism are known to affect the organization of communities at lower trophic levels by preventing monopolization of resources by dominant species (Paine, 1974; Lafferty et al., 2006). Competition and predation are not independent of each other and often work in synchrony or as complementary mechanisms of community organization (Menge and Sutherland, 1976).
In the last two decades, however, there have been a number of empirical and theoretical studies that have highlighted positive interactions in communities and their effects on species coexistence (Stachowicz, 2001; Bruno et al., 2003; Gross, 2008; Crotty and Bertness, 2015; Bulleri et al., 2016). Empirical research on positive interactions within communities has largely focused on interactions such as facilitation (Bertness and Callaway, 1994; Callaway et al., 2002; Butterfield, 2009) and mutualistic interactions (reviewed in Bronstein, 1994) between dissimilar species [e.g., nurse plants promoting seedling survival (Wood and Del Moral, 1987; Callaway, 1998) and non-obligate symbiosis in marine intertidal species (Mouritsen and Poulin, 2003)]. Inferring processes from community patterns can be unreliable given that trait overdispersion can be an effect of both competition (Gotelli and McCabe, 2002) or positive interactions between dissimilar species (Valiente-Banuet and Verdú, 2007).
However, positive interactions are also found between species that use similar resources or share a guild in multi-species communities (Crowley and Cox, 2011). Mixed-species social groups are an example of positive interactions among species that are ecologically similar (Sridhar et al., 2012a). Mixed-species groups are a dynamic functional subset of communities that form for the benefit of the participating species and function independently of the community while they are together. At the same time, there is often turnover of species such that groups are often fluid (Goodale et al., 2010). Interactions within them have more proximate, immediate consequences to the participants involved. For example, communal mobbing can deter predators (Caro, 2005), or species can benefit from the foraging of other group members by feeding on disturbed prey (McLean, 1984; Hino, 1998; Sridhar and Shanker, 2014b) or stealing prey (Satishchandra et al., 2007; Flower and Gribble, 2012).
Mixed-species groups are seen across taxa [e.g., fish (Lukoschek and McCormick, 2000), ungulates (Sinclair, 1985), primates (Struhsaker, 1981), and birds (Sridhar et al., 2009)]. In passerine bird communities across tropical and temperate forests, individuals of multiple species associate and move together while foraging. Such foraging groups of two or more species are known as mixed-species bird flocks (Greenberg, 2000). These flocks provide two main group-living benefits while reducing the costs of competition from conspecifics–(1) Better foraging efficiency, (2) Anti-predatory benefits (Greenberg, 2000; Sridhar et al., 2009). Mixed-species bird flocks are composed largely of insectivorous and sometimes frugivorous passerine birds that often exploit similar resources (Greenberg, 2000; Goodale et al., 2015).
Earlier research has shown that different types of interactions can take place within mixed-species flocks. In a study on a global dataset from different flock systems, species that were ecologically and phenotypically more similar to each other, were found to have higher association strengths (Sridhar et al., 2012a), suggesting positive interactions among phenotypically-similar species. On the other hand, some positive interactions within flocks were based on dissimilarity between partners with specialized feeding habits [e.g., sallying species in flocks are known to closely follow highly active gleaners for foraging benefits (Sridhar and Shanker, 2014b)]. Previous studies on flocks have also found evidence for competition in mixed-flocks. Graves and Gotelli (1993) found “checkerboard distributions” among Amazonian flocks where congeneric species did not cooccur. A more recent study found that both interspecific and inter-guild competition played a role in structuring flocks in the Andes (Colorado and Rodewald, 2015). Even in datasets that are phenotypically clumped overall, large flocks tend to contain very diverse species (Sridhar et al., 2012a; Goodale et al., 2017; Mammides et al., 2018). These contrasting patterns within flocks suggest that the rules of assembly may vary based on flock size and morphological and ecological traits.
Mixed species groups may offer a unique opportunity to solve the paradox that similarity both confers benefits and imposes costs. Unlike single-species groups, species in mixed-species groups can vary along different axes of phenotypic similarity. It is possible that species are similar to each other along phenotypic axes where similarity is important for mutual benefit and at the same time may differ along phenotypic/ecological axes where being similar to a social partner may lead to competitive interactions, thus delinking the matching of traits between participants (Seppanen et al., 2007; Sridhar and Guttal, 2018; Goodale et al., 2020). Associating with phenotypically similar species can have positive and negative consequences for flock participants. Similar individuals share predators and hence the benefits from dilution effects, heterospecific alarm calling (Magrath et al., 2015), relevant social information (Goodale et al., 2010; Fallow et al., 2011), and activity matching (Sridhar and Guttal, 2018) are expected to be higher in groups with higher overall similarity.
Flock size variation has been reported in different systems of mixed-species flocks across the world (Greenberg, 2000). We attempt to resolve seemingly contrary earlier findings (i.e., overall pattern of phenotypic clumping, concomitant with observations of phenotypic heterogeneity in large flocks), by examining how phenotypic heterogeneity changes with flock size. A community is said to be phenotypically clumped if it is composed of species phenotypically or ecologically more similar than expected by chance, as represented by a null model. Trait-heterogeneity in large flocks could come about through different local-scale processes as flocks assemble: (1) From a species pool, the most phenotypically similar species aggregate initially, followed by species that are most similar to the mean of the initial aggregation, and so on; (2) Joining order should not matter, and species are added in no particular order at each stage; (3) The most dissimilar species aggregate at the initial stages to form small groups, and these stages merge to form larger groups with time (demonstrated in Figure 1). In each case, heterogeneity of the final group may be similar, yet intermediate stages are expected to differ. Given previous findings, we expect to find the first pattern to be most common. We tested this using a primary dataset on flocks from the Western Ghats, India.

Figure 1. Hypothetical alternate routes of flock assembly leading to the same outcome. (1) Flocks formed by the coming together of phenotypically similar species. (2) Species additions to flocks were random with respect to phenotypic similarity. (3) Flocks formed by the coming together of phenotypically dissimilar species initially, and these subgroups merged later to form a large flock.
Given these expectations, we also examined variation in phenotypic clumping relative to flock richness using the dataset reported in Sridhar et al. (2012b) from sites across the world. We expected that sites with lower mean flock richness would exhibit greater phenotypic clumping. However, this effect is also likely to be affected by the availability of flock participant species from the local species pool (i.e., the total number of species recorded participating in flocks at any given study site). At sites with larger species pools, species were expected to have a greater choice of social partner species relative to sites with smaller species pools. Therefore, we expected that phenotypic clumping would be greatest at locations with smaller ratios of mean flock richness to mean pool richness. We tested this using a dataset of 55 flock data matrices from 24 sites across the world, from both temperate and tropical regions (Sridhar et al., 2012b).
Materials and Methods
Local Analysis
Study Area
The study was conducted in the Anshi Range of Kali Tiger Reserve, Karnataka, India. Anshi is low- to mid-elevation tropical semi-evergreen forest, with a matrix of open farmlands and scrub habitat closer to villages in the area. All study sites were located around Anshi Nature Camp (15.00°N, 74.39°E) and Anshi Village (14.99°N, 74.37°E). The data were collected in two field seasons (January–March 2010 and December 2016–March 2017). This overlapped with the non-breeding season of birds in the study area and represented the peak of migratory bird activity.
Data Collection
We collected data on flock composition by walking 12 forest trails. Each trail was approximately 3–5 km long. We sampled one trail per day and actively searched for mixed-species flocks. An active foraging group of birds, comprising two or more species moving together for at least 5 min, was classified as a mixed-species flock. An individual was said to be in the flock if it was within a 10 m radius from its nearest neighbor in the group. Once we encountered a flock, we followed it for 15 min and recorded all species present in the flock detected visually and acoustically. We set a 15 min cut-off for our observations based on our previous experience of observing flocks in these areas (Sridhar et al., 2013). Given the habitat structure and the ability of an observer to detect flocks, the 15 min cut-off allowed us to capture a “snapshot” of the flock while making sure we either visually or acoustically detected most species present in the flock.
Trait Data
We estimated the foraging height of an individual bird every time it was observed in the flock and assigned it to foraging height categories: 0–2, 2–4, 4–8, 8–16, 16–32, > 32 meters. First, for every flock where a species was observed, we ascribed the mid-point of the foraging height class to the observed individual as its foraging height. The species foraging height was the mean of these mid-points. We also conducted focal sampling on arbitrarily selected individuals of flocking species to obtain data on foraging behavior. Every time a foraging attempt was made, we classified it into five categories: sally, glean, sally-glean, bark probe, peck (modified from Remsen and Robinson, 1990). Species that foraged on the ground were categorized ground foragers. Species that did not exhibit a particular foraging behavior > 50% of the time were classified as “searcher.” Based on these criteria, each species that participated in a flock was classified as a sallyer/gleaner/sally-gleaner/bark feeder/searcher/ground forager. “Sally-gleaner” refers to birds that typically make short sallying flights from a perch and pick insects off a surface. Data on body masses were obtained from Dunning (2008).
Statistical Analyses
Approach
We used 15-min observational data on flocks of different richness to understand how species assemble in large mixed-species flocks. Flocks in our study area were dynamic with species joining and leaving at different times. Therefore, by looking at snapshots of flocks of different richness, we examined how flock composition, in relation to species similarity, changed with flock richness. We assumed that flocks of different richness represented different stages in flock formation.
Estimating group sizes in flocking species, especially of gregarious species, is very challenging in dense tropical forests. Mixed-species flocks are known to increase in size by addition of newer species rather than more individuals of the same species (Powell, 1985). We therefore used flock richness as a representative of flock size, since flock abundance and richness are largely known to be correlated in tropical flocks (see Goodale et al., 2009). Flocks with richness between 2 and 5 were classified as small flocks, flocks with richness between 6 and 9 were medium flocks and all flocks with richness greater than or equal to 10 species (maximum flock richness observed in our dataset was 22 species) were classified as large flocks.
Body Size
For each flock, we calculated the standard deviation in body size values of flock participant species, which we used as an index of variation for each flock. We calculated the mean index of variation (Vbs) for each flock richness category for our observed data.
Foraging Height
For each flock, we calculated mean foraging height for each species based on field observations described in the data collection section. In each flock, we calculated the standard deviation in the foraging height of participant species, which was used as an index of variation of foraging height for each flock. We calculated a mean index of variation (Vfh) for each flock richness category.
Foraging Behavior
Each species was assigned to one of the six foraging behavior categories or guilds (sallyer/gleaner/sally-gleaner/bark feeder/searcher/ground forager) based on field observations. We dropped members of the sallying guild from the analysis because sallying species within flocks forage by feeding on insects flushed up by other species within the flock or by kleptoparasitism (Sridhar and Shanker, 2014b). Sallyers, thus, are specialists that follow other members of the flock to facilitate their own foraging efficiency. These sallyers appear to be the only guild that increase direct foraging benefits by following other members of the flock in our study area (Sridhar and Shanker, 2014b). These complementary benefits are qualitatively different from the supplementary benefits that most other taxa within the flock accrue (Goodale et al., 2020).
To get an index of variation for foraging behavior, we calculated the number of different foraging behavior categories present in each flock. Larger numbers indicated higher variation in foraging habit for each flock. We then calculated mean number of categories (Vfb) for small-, medium-, and large-sized flocks.
Generating Null Flocks
The flock composition data were randomized to create null flocks that were then compared to the observed dataset. We randomized the species-by-flock matrix 1,000 times, treating flock sizes (column totals) constant and species occurrences (row totals) as probabilities (Gotelli, 2000). Since we were interested in examining the differences in phenotypic variation in flock richness categories, we held flock richness classes constant while setting up the null model using sim5 in EcoSimR implemented in R (Gotelli et al., 2015; R Core Team, 2018). This algorithm used a species-by-flock matrix and assigned sampling weights to each species proportional to their occurrence in flocks. It filled out columns in an empty matrix with probability of each cell being filled being proportional to row sums (species occurrence in our case). It also allowed for us to hold column totals fixed, which in this case was flock richness. This allowed us to randomize species (and as a result, the trait distribution in flocks, while keeping flock richness categories constant). We calculated the variation indices described above for each flock in each simulation. We then calculated mean of standard deviation and the variation around it (2 ∗ SD), for 1,000 simulations for each flock richness category. Our null model approach controlled for the potential effect of flock size on the average values of traits. The mean variation and the standard deviation around the variation were used for calculating the standardized effect sizes for small-, medium-, and large-sized flocks.
We compared the variation in our observed data with that of the randomized data to understand if the observed values varied more than or less than expected by chance. We calculated standardized effect sizes (SES) as follows:
where VObserved is the observed variation, VExpected is the mean over 1,000 simulations of expected variation and SD(VExpected) is the standard deviation around the mean expected variation.
Effect sizes can vary from positive to negative values where “positive” indicates values more than expected by chance and “negative” indicates values less than expected by chance. In general, effect sizes less than −1.96 and greater than +1.96 indicated statistical significance (Gotelli and McCabe, 2002). This is based on standardized effect sizes coming from a standard normal distribution (mean = 0, SD = 1) in which case 2.5% of observations lie below −1.96 and 2.5% lie above +1.96. In our case, effect sizes greater than +1.96 indicate significantly greater variation than expected by chance (i.e., no phenotypic clumping). Effect sizes less than −1.96 indicated variation significantly less than expected by chance (i.e., phenotypic clumping). We used these thresholds while making inferences about phenotypic clumping in the Results section. All the above analyses were performed on data from both seasons. We analyzed the data from the two seasons separately to check if the pattern was consistent across years.
Global Analysis
We expected that sites that have lower mean flock richness should show greater phenotypic clumping. However, this effect is also likely to be affected by the availability of flock participant species in the area.
We obtained estimates of phenotypic clumping for 55 species-by-flock matrices from 24 study sites across the world from Sridhar et al. (2012a). Phenotypic clumping in Sridhar et al. (2012a) was calculated as the effect size of the relationship between pairwise association strength and trait similarity for all species pairs. Phenotypic clumping was calculated separately for similarities in three traits: body size, foraging behaviour, and taxonomy (i.e., classification of every species pair as belonging to the same genus or different) from the study (Table 1 in Sridhar et al., 2012a; for detailed methods, refer to Supplementary Material 1). For each of these 55 datasets (Sridhar et al., 2012b), we calculated the mean flock richness and the total species pool richness, and examined the relationship between phenotypic clumping and ratio of mean flock richness: species pool richness using Spearman’s rank correlation. We expected phenotypic clumping to be higher in sites that had smaller mean flock richness: species pool richness ratios.
All analyses were implemented in R version 3.5.1 (R Core Team, 2018).
Results
Local Analysis
We recorded a total of 247 flocks in 2010 and 123 flocks in 2017, with flock richness ranging from 2 to 22 species in 2010 (mean 6.7 ± 3.9 SD species per flock) and 2–21 species in 2017 (mean 6.56 ± 4.09 SD species per flock). Overall, a total of 52 species participated in flocks across both years. The species in our study area varied in their intraspecific sociality. Some species were found in conspecific groups [e.g., brown-cheeked fulvetta (Alcippe poioicephala), orange minivet (Pericrocotus flammeus)] whereas others were found in pairs or were solitary. The intraspecifically gregarious species are known to be important for flock initiation and leadership in flocks (Sridhar and Shanker, 2014a).
Phenotypic Clumping: Flock Heterogeneity as a Function of Flock Richness
For 2010, we found that phenotypic clumping in body size (i.e., difference between observed and expected variation) was highest and statistically significant for small flocks (Table 1 and Figure 2) (i.e., small flocks were more phenotypically clumped in body size than expected by chance). The clumping was lower but statistically significant for medium-sized flocks and was not significant for large flocks. For 2017, phenotypic clumping in body size was highest and statistically significant in small flocks, lower but not significant in medium-sized flocks and was the least and not significant for large flocks (Figure 2 and Table 1). A similar trend of decreasing difference between observed and expected values from small to large flocks was observed for foraging height in 2010 (Figure 2 and Table 1). However, a clear trend was not observed in 2017 (Figure 2 and Table 1). We also did not see a clear pattern for foraging behavior. In 2010, the standardized effect size was smallest and statistically significant for the medium-sized flocks, followed by small, but not for large -sized flocks (Figure 2 and Table 1). The trend in increase in variation with increase in flock size was not clear. In 2017, we observed a decreasing trend in phenotypic clumping in foraging behavior (Figure 2 and Table 1).
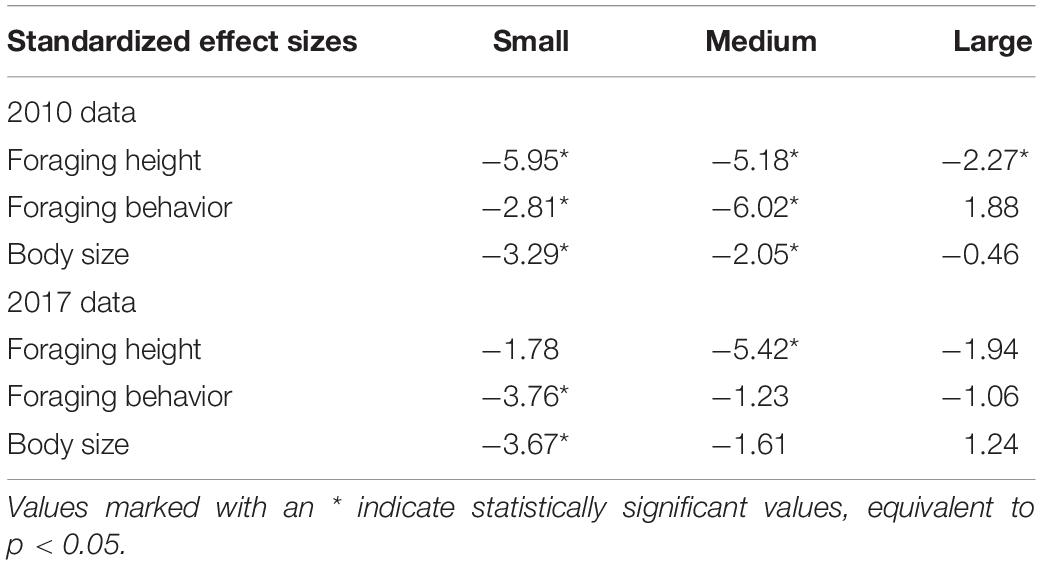
Table 1. Standardized effect size values for different phenotypic traits for different flock richness classes.
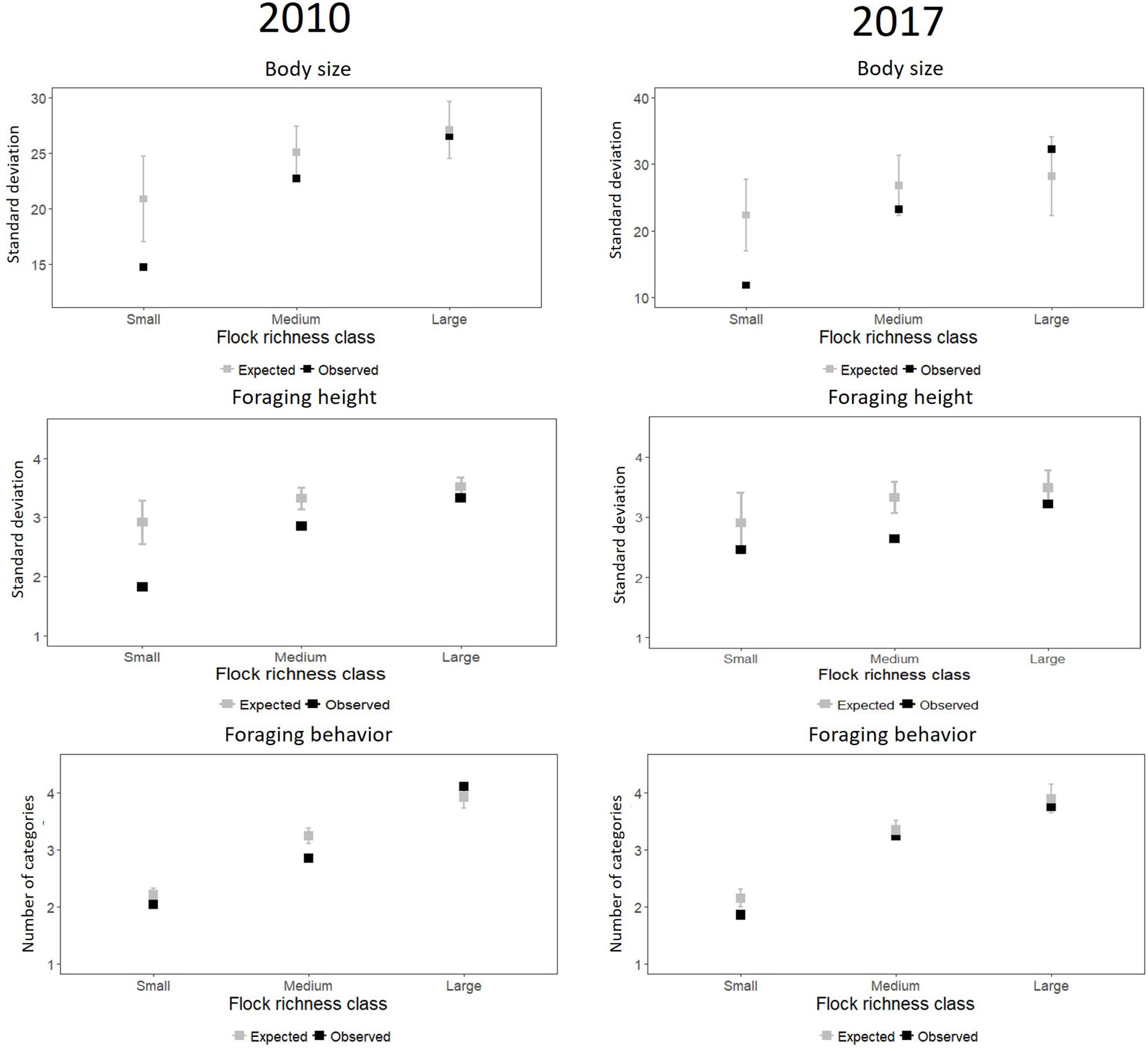
Figure 2. Observed and expected mean of variation in the three trait values (body size, foraging height, foraging behavior) for small-, medium-, and large-sized mixed-species bird flocks from Western Ghats, India. The gray points are the means of the standard deviation from 1,000 simulations and the gray lines indicate 2*standard deviation (obtained from the 1,000 simulations) for each size class. Black points are the observed standard deviation averaged over samples in each flock richness class.
Global Analysis
In the global analysis, we found a negative relationship between phenotypic clumping in body size and the ratio of flock richness: species pool (Spearman’s rho = −0.54, p = 0.001, Figure 3). We found that communities with smaller flock richness tended to be phenotypically more clumped in body size compared to communities with larger flock richness. However, this pattern was slightly weaker for foraging behavior (Spearman’s rho = −0.48, p = 0.001, Figure 3) and not statistically significant for taxonomic relatedness between species pairs (Spearman’s rho = −0.093, p = 0.531, Figure 3).
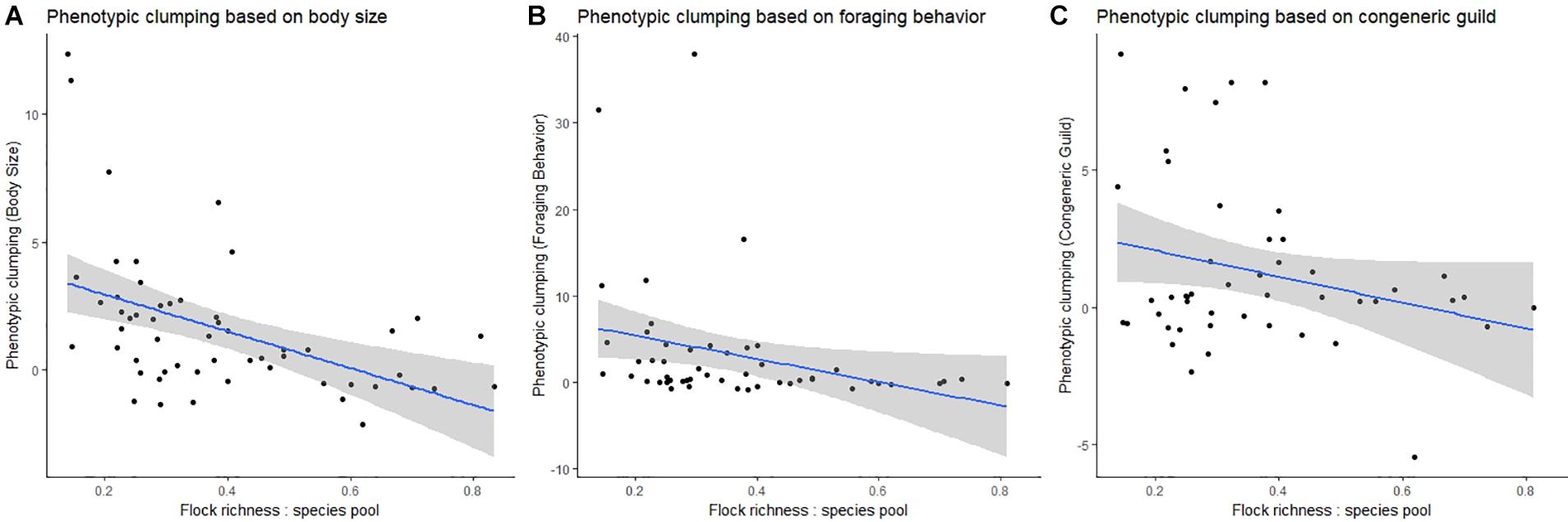
Figure 3. Relationship between different measures of phenotypic clumping (y-axis in each plot) and the ratio of mean flock richness: species pool richness (x-axis). Each represents the mean for flocks from a single locality. (A) Phenotypic clumping based on body size, (B) phenotypic clumping based on foraging behavior, and (C) phenotypic clumping based on congeneric guild.
Discussion
In this study, we examined rules of flock assembly and explored patterns of phenotypic and ecological heterogeneity in relation to flock size in mixed-species bird flocks. In the primary dataset, we found that small flocks were phenotypically more clumped than expected by chance, especially with respect to body size. Similarity between flock participants approached the expected (random) variation in flocks of larger richness. In the analysis with the global dataset, there was greater phenotypic clumping based on body size in communities where mean flock richness was smaller while controlling for the total species pool. However, this pattern was weaker for foraging behavior. Clumping based on congeneric guild was not correlated with mean flock size.
Role of Species Similarity in Flock Assembly
We found that phenotypic clumping was reduced with increase in flock richness. In large flocks, similarity-based benefits may be less important (e.g., adjustments to match rate of activities may be a smaller cost compared to the benefits of being in larger groups). Larger flocks might also be more accommodating of different foraging behaviors by opening up different niche spaces (Harrison and Whitehouse, 2011). Flock size or richness represents an important change in flock context and could affect the nature of interactions in flocks. There are benefits of both group size and similarity in group-living species, but there may be a trade-off between the two. Especially in a mixed-species group context, where an increase in phenotypic heterogeneity is inevitable with an increase in number of species, individual participants may have to compromise on similarity related benefits. Such benefits associated with group augmentation may be related to better foraging opportunities, food finding and social information. In large flocks, every species pair may not interact directly but there may be some species within them that may interact directly with each other. This may lead to different tradeoffs between flock competition and benefits from being similar, based on context.
Global Patterns
Although some studies find that flocks are based on positive associations between similar species (Sridhar et al., 2012a), others have found evidence for flock assembly being governed primarily by competition between similar species (Graves and Gotelli, 1993; Colorado and Rodewald, 2015). Colorado and Rodewald (2015), on the one hand, find evidence for the guild proportionality rule—where species from different foraging guilds are found in equal proportions across flocks (Ricklefs, 1987; Wilson, 1989) but, on the other hand, also find patterns of aggregation in body size in the Andean mixed-species flock community. Globally, there is known to be variation in patterns of assembly in flocks (Graves and Gotelli, 1993; Gomez et al., 2010; Sridhar et al., 2012a; Colorado and Rodewald, 2015).
Results from the primary data suggested that the assembly of flocks varied with flock richness. We speculate that this may also be reflected in the phenotypic assembly of flocks from different sites. We found that there was greater phenotypic clumping in body size in areas where mean flock richness was small. This relationship was weaker for clumping with respect to foraging behavior and mean flock richness. We found no relationship between phenotypic clumping in congeneric guilds and the ratio of flock richness: species pool. Congeneric species pairs are likely to have the most overlap in traits because phylogenetically conserved species’ traits would lead to greater competition (Graves and Gotelli, 1993). Many studies that test for competition in community assembly use cooccurrence of congeneric species pairs as a measure of competition (Graves and Gotelli, 1993; Sfenthourakis et al., 2006). We did not find evidence for a relationship between flock richness and clumping based on relatedness (i.e., species pairs belonging to the same genus or not) in datasets from around the world.
Thus, we found a pattern of similarity in small-sized flocks along the body-size axis, a weaker pattern for foraging behavior and no pattern for the taxonomic similarity axes. It is possible that species of similar body size benefit from flocking together because they share the same predators. However, in our study site, we know very little about the diets of avian predators, the most likely predators of birds in flocks. Another possible explanation for stronger association between similarly sized species is better matching of foraging activities (Sridhar and Guttal, 2018). The risk of being an outlier in a group is higher at smaller group sizes and this effect disappears in larger groups (Landeau and Terborgh, 1986). Both taxonomic and foraging behavioral similarity can potentially lead to competitive interactions between flock participants (Peiman and Robinson, 2010). Our study demonstrates that species similarity along different trait axes may be delinked in mixed-species flocks.
Why Does Clumping Reduce as Flock Size Increases?
In a recent theoretical study on phenotypically heterogenous populations, there was greater homogeneity in smaller groups (Nair et al., 2019). The authors found that when heterogeneity was based on different phenotypes, small groups assorted based on such phenotypes. Ecologically, phenotypes are the values that different traits take, and phenotypic similarity along each trait could influence interactions in the group in a different manner. In our study, we examined three axes of phenotypic similarity in flocks—body size, foraging height and foraging behaviour—to assess the change in phenotypic heterogeneity as flocks get larger at our primary study site. We found that small flocks were more phenotypically clumped than expected by chance for all of the traits that we examined. However, the pattern of decrease in phenotypic clumping with increase in group size was most prominent for body size in our study. Reasons for this pattern may include increased phenotypic heterogeneity in large flocks, which could reflect a reduction in availability of phenotypically-similar flock associates in large flocks. Although larger flocks are expected to be less clumped than small flocks, we consider phenotypic clumping in comparison to what may be expected by chance. At the initial stages of flock formation, species can choose similar partners from a large pool, but as more species are added to the group, the variation available from the species pool reduces and, eventually, dissimilar species are added to the flock. Thus, if similarity was the most important factor for benefits to participants, one would expect to find multiple small flocks (i.e., the availability of similar species in the pool would constrain flock richness and large flocks would be rare). However, our findings suggest that variation in large flocks is not different from what is expected by chance and that there are potential benefits to being in large flocks while compromising on the similarity of flocking partners. Mixed-species flocks may also exhibit niche construction, and open up more niche spaces as they get larger (Harrison and Whitehouse, 2011), which can be occupied by a greater diversity of species; additional niches may also become more accessible to the existing participants. In general, species niches are then likely to be shaped by a greater diversity of species in areas with large flocks as opposed to areas with smaller flocks. In each of these cases, the participant species may receive different kinds of benefits (e.g., in small flocks, the benefits may come largely from similar species grouping for protection while in diverse flocks with more dissimilarity, some species may gain foraging benefits from tracking foraging locations or feeding on flushed insects).
As flock richness increases, the benefits that species derive from participating in flocks may change, and phenotypic similarity may not be an important factor in choosing flock partners. As a result, species might become less choosy at higher flock richness. At smaller group sizes, supplementary benefits (Goodale et al., 2020) based on similarity of flock partners might be more important since the overall group size is small. Every addition of an individual to the group accounts for a significant decrease in threat from predators because of the dilution effect in smaller groups, while this benefit may diminish at larger flock sizes (Hamilton, 1971). One would therefore expect higher similarity at smaller values of flock richness. Phenotypic-heterogeneity, while accounting for the null expectation in mixed-species flocks is likely to increase at higher flock richness. The nature of benefits in large groups may thus be different from those in small groups. If traits are delinked, we expect that this pattern will be observed more strongly when trait matching confers benefit (i.e., in body size) than when it results in competition (e.g., in the case of foraging habits) (Sridhar and Guttal, 2018; Goodale et al., 2020).
Groups are expected to be larger when there is increased risk of predation and smaller in a foraging context (Hoare et al., 2004). In heterospecific groups, as flock richness increases, competition between similar individuals is likely to increase. Therefore, at later stages in flock formation, competition could be mitigated by the addition of dissimilar species where in fact increased foraging can manifest through complementary foraging habits. Individuals are also known to engage in more risky behavior while foraging at larger group sizes where the predation risk is diluted, compared to when in smaller groups (Grand and Dill, 1999). In fact, in many group-living species, there is an increase in food intake rate with an increase in group size although this depends on the dispersion of resources (Beauchamp, 1998). We did not see a clear decreasing pattern in phenotypic clumping in foraging behavior and foraging height with flock size as observed for the other traits. Within the insectivorous guild of birds that made up the flocking species pool, there was variation in search strategy for insects through the use of different manoeuvers. Foraging behavior and height both represented axes of resource utilization and resource overlap on these axes could lead to competition between similar species cooccurring in small flocks (MacArthur, 1958; Morse, 1970; Eguchi et al., 1993; Wheeler and Calver, 1996). Therefore, trait dispersion may occur at initial flock formation, even in small mixed- species flocks. In our primary dataset, however, we find that small flocks are phenotypically more clumped as opposed to larger flocks.
One of the limitations of this study is that we do not include the abundance of species in the analysis. These numbers are often difficult to estimate in tropical flocks. However, flocks often increase in size, mainly by addition of new species rather than an increase in conspecific group size of existing species in the flock (Powell, 1985). Also, we arrive at the mechanism of flock assembly indirectly by addressing questions about phenotypic composition. A more direct measure would be to follow flocks from their conception and track changes in composition in real time. The intermediate flock stages in our study may be a result of flocks that are in the process of assembly or flocks that are disintegrating. We assume that, irrespective of this, flock size would be representative of the heterogeneity of that stage.
Conclusion
This study provides empirical support for the idea that mixed-species flocking provides a way to delink the costs and benefits of social grouping. We use the approach of examining snapshots of flocks to understand flock formation and examine changes in heterogeneity across flocks of different richness. Our approach provides insights into how trait values accumulate in flocks. Observing the sequence of flock formation is often difficult in the wild, especially for flocks where species turnover is observed over small timescales. Our approach can further be extended and applied to other heterospecific animal groups. We propose that phenotypic assembly of flocks changes with flock richness. We also found that the rules of phenotypic assembly depend on the particular phenotypic trait.
Mixed-species flocks are an important part of bird communities across the world. Interactions in mixed-species flocks and associations between species are likely to have important consequences for local bird communities where flocking species constitute more than 50% of the bird fauna in some habitats, especially in the tropics (Goodale et al., 2015). The role of behavioral interactions in community organization is often overlooked and the need for incorporating behavior in community ecology has been highlighted (Gordon, 2011; Toms, 2013). Our results suggest that the nature of biotic interactions—whether they are positive, neutral or negative—depends on group size. Therefore, one needs to be careful about making inferences about community-level processes based on species association matrices. Against this background, our study emphasizes the importance of considering context and interactions between species in community organization.
Data Availability Statement
The datasets generated for this study are available as Supplementary Material.
Ethics Statement
The animal study was reviewed and approved by the Karnataka Forest Department which includes ethical treatment of animals; where required, projects are further referred to the “Committee for the Purpose and Control and Supervision of Experiments on Animal (CPCSEA)” of the Ministry of Environment and Forests, Government of India.
Author Contributions
PB, KS, and HS conceptualized the study. PB and HS collected primary field data and conducted the analysis. HS collated the global datasets. PB wrote the first draft of the manuscript. All authors contributed to revisions of the draft.
Funding
Fieldwork was supported by the funding from the Department of Biotechnology—Indian Institute of Science Partnership Programme and a grant to HS from the International Foundation for Science (grant no. D/4910-1). HS was a researcher in Vishwesha Guttal’s research group and was supported by a fellowship from the Indian National Science Academy during the period of this project.
Conflict of Interest
The authors declare that the research was conducted in the absence of any commercial or financial relationships that could be construed as a potential conflict of interest.
Acknowledgments
We would like to thank Karnataka Forest Department for permits to work in Anshi National Park, Karnataka, India. We thank Prakash, Nagesh, Narayan, Chandrakant, Sadanand, Sandeep, Bala, Prasad, Pandurang, Prashant, and Ramdas for help with fieldwork. We would like to thank two reviewers for their valuable inputs on the manuscript. PB thanks Shashank Johri for help with creating Figure 1.
Supplementary Material
The Supplementary Material for this article can be found online at: https://www.frontiersin.org/articles/10.3389/fevo.2020.537816/full#supplementary-material
References
Beauchamp, G. (1998). The effect of group size on mean food intake rate in birds. Biol. Rev. 73, 449–472. doi: 10.1017/S0006323198005246
Bertness, M. D., and Callaway, R. (1994). Positive interactions in communities. Trends Ecol. Evol. 9, 191–193. doi: 10.1016/0169-5347(94)90088-4
Bronstein, J. L. (1994). Our current understanding of mutualism. Q. Rev. Biol. 69, 31–51. doi: 10.1086/418432
Bruno, J. F., Stachowicz, J. J., and Bertness, M. D. (2003). Inclusion of facilitation into ecological theory. Trends Ecol. Evol. 18, 119–125. doi: 10.1016/s0169-5347(02)00045-9
Bulleri, F., Bruno, J. F., Silliman, B. R., and Stachowicz, J. J. (2016). Facilitation and the niche: implications for coexistence, range shifts and ecosystem functioning. Funct. Ecol. 30, 70–78. doi: 10.1111/1365-2435.12528
Butterfield, B. J. (2009). Effects of facilitation on community stability and dynamics: synthesis and future directions. J. Ecol. 97, 1192–1201. doi: 10.1111/j.1365-2745.2009.01569.x
Callaway, R. M. (1998). Are positive interactions species-specific? Oikos 82, 202–207. doi: 10.2307/3546931
Callaway, R. M., Brooker, R. W., Choler, P., Kikvidze, Z., Lortie, C. J., Michalet, R., et al. (2002). Positive interactions among alpine plants increase with stress. Nature 417, 844–848. doi: 10.1038/nature00812
Caro, T. (2005). Antipredator Defenses in Birds and Mammals. Chicago, IL: University of Chicago Press.
Cody, M. L. (1974). Competition and the Structure of Bird Communities (Monographs in Population Biology - Volume 7). Princeton, NJ: Princeton University Press.
Colorado, G. J., and Rodewald, A. D. (2015). Assembly patterns of mixed-species avian flocks in the Andes. J. Anim. Ecol. 84, 386–395. doi: 10.1111/1365-2656.12300
Connell, J. H. (1961). The influence of interspecific competition and other factors on the distribution of the barnacle Chthamalus Stellatus. Ecology 42, 710–723. doi: 10.2307/1933500
Crotty, S. M., and Bertness, M. D. (2015). Positive interactions expand habitat use and the realized niches of sympatric species. Ecology 96, 2575–2582. doi: 10.1890/15-0240.1
Crowley, P. H., and Cox, J. J. (2011). Intraguild mutualism. Trends Ecol. Evol. 26, 627–633. doi: 10.1016/j.tree.2011.07.011
Eguchi, K., Yamagishi, S., and Randrianasolo, V. (1993). The composition and foraging behaviour of mixed-species flocks of forest-living birds in Madagascar. Ibis 135, 91–96. doi: 10.1111/j.1474-919X.1993.tb02814.x
Fallow, P. M., Gardner, J. L., and Magrath, R. D. (2011). Sound familiar? Acoustic similarity provokes responses to unfamiliar heterospecific alarm calls. Behav. Ecol. 22, 401–410. doi: 10.1093/beheco/arq221
Flower, T. P., and Gribble, M. (2012). Kleptoparasitism by attacks versus false alarm calls in fork-tailed drongos. Anim. Behav. 83, 403–410. doi: 10.1016/j.anbehav.2011.11.009
Forsman, J. T., Monkkonen, M., and Hukkanen, M. (2001). Effects of predation on community assembly and spatial dispersion of breeding forest birds. Ecology 82, 232–244. doi: 10.1890/0012-9658(2001)082[0232:eopoca]2.0.co;2
Gomez, J. P., Bravo, G. A., Brumfield, R. T., and Cadena, C. D. (2010). A phylogenetic approach to disentangling the role of competition and habitat filtering in community assembly of Neotropical forest birds. J. Anim. Ecol. 79, 1181–1192. doi: 10.1111/j.1365-2656.2010.01725.x
Goodale, E., Bangal, P., and Sridhar, H. (2019). Mixed-Species Bird Flocks: A Bibliography. Available online at: http://www.animal-ecology-guangxi.com/content/mixed-species-animal-groups.
Goodale, E., Beauchamp, G., Magrath, R. D., Nieh, J. C., and Ruxton, G. D. (2010). Interspecific information transfer influences animal community structure. Trends Ecol. Evol. 25, 354–361. doi: 10.1016/j.tree.2010.01.002
Goodale, E., Beauchamp, G., and Ruxton, G. D. (2017). Mixed-Species Groups of Animals: Behaviour, Community Structure and Conservation. London: Academic Press.
Goodale, E., Ding, P., Liu, X., Martínez, A., Si, X., Walters, M., et al. (2015). The structure of mixed-species bird flocks, and their response to anthropogenic disturbance, with special reference to East Asia. Avian Res. 6:14. doi: 10.1186/s40657-015-0023-0
Goodale, E., Nizam, B. Z., Robin, V. V., Sridhar, H., Trivedi, P., and Kotagama, S. W. (2009). Regional variation in the composition and structure of mixed-species bird flocks in the Western Ghats and Sri Lanka. Curr. Sci. 97, 648–663.
Goodale, E., Sridhar, H., Sieving, K. E., Bangal, P., Colorado, Z. G. J., Farine, D. R., et al. (2020). Mixed company?: a framework for understanding the composition and organization of mixed-species animal groups. Biol. Rev. 95, 889–910. doi: 10.1111/brv.12591
Gordon, D. M. (2011). The fusion of behavioral ecology and ecology. Behav. Ecol. 22, 225–230. doi: 10.1093/beheco/arq172
Gotelli, N. J. (2000). Null model analysis of species co-occurrence patterns. Ecology 81, 2606–2621. doi: 10.1890/0012-9658(2000)081[2606:nmaosc]2.0.co;2
Gotelli, N. J., Hart, E., and Ellison, A. (2015). EcoSimR: Null Model Analysis for Ecological Data. R package Version 0.1.0. Available online at: http://github.com/gotellilab/EcoSimR.
Gotelli, N. J., and McCabe, D. J. (2002). Species co-occurrence: a meta-analysis of J. M. diamond’s assembly rules model. Ecology 83, 2091–2096. doi: 10.1890/0012-9658(2002)083[2091:scoama]2.0.co;2
Grand, T., and Dill, L. (1999). The effect of group size on the foraging behaviour of juvenile coho salmon: reduction of predation risk or increased competition? Anim. Behav. 58, 443–451. doi: 10.1006/anbe.1999.1174
Graves, G. R., and Gotelli, N. J. (1993). Assembly of avian mixed-species flocks in Amazonia. Proc. Natl. Acad. Sci. U.S.A. 90, 1388–1391. doi: 10.1073/pnas.90.4.1388
Greenberg, R. (2000). “Birds of many feathers: the formation and structure of mixed- species flocks of forest birds,” in On the Move: How and Why Animals Travel in Groups, eds S. Boinski and P. A. Garber (Chicago. IL: The University of Chicago Press), 521–558.
Gross, K. (2008). Positive interactions among competitors can produce species-rich communities. Ecol. Lett. 11, 929–936. doi: 10.1111/j.1461-0248.2008.01204.x
Harrison, N. M., and Whitehouse, M. J. (2011). Mixed-species flocks: an example of niche construction? Anim. Behav. 81, 675–682. doi: 10.1016/j.anbehav.2011.01.013
Hiltunen, T., and Laakso, J. (2013). The relative importance of competition and predation in environment characterized by resource pulses - an experimental test with a microbial community. BMC Ecol. 13:29. doi: 10.1186/1472-6785-13-29
Hino, T. (1998). Mutualistic and commensal organization of avian mixed-species foraging flocks in a forest of Western Madagascar. Jounal Avian Biol. 29, 17–24. doi: 10.2307/3677336
Hoare, D. J., Couzin, I. D., Godin, J. G. J., and Krause, J. (2004). Context-dependent group size choice in fish. Anim. Behav. 67, 155–164. doi: 10.1016/j.anbehav.2003.04.004
Janson, C. H., and Van Schaik, C. P. (1988). Recognizing the many faces of primate food competition: methods. Behaviour 105, 165–186. doi: 10.1163/156853988X00502
Kelt, D. A., Taper, M. L., and Meserve, P. L. (1995). Assessing the impact of competition on community assembly?: a case study using small mammals. Ecology 76, 1283–1296. doi: 10.2307/1940935
Koeppel, A. F., and Wu, M. (2014). Species matter: the role of competition in the assembly of congeneric bacteria. ISME J. 8, 531–540. doi: 10.1038/ismej.2013.180
Lafferty, K. D., Dobson, A. P., and Kuris, A. M. (2006). Parasites dominate food web links. Proc. Natl. Acad. Sci. U.S.A. 103, 11211–11216. doi: 10.1073/pnas.0604755103
Landeau, L., and Terborgh, J. (1986). Oddity and the ‘confusion effect’ in predation. Anim. Behav. 34, 1372–1380. doi: 10.1016/s0003-3472(86)80208-1
Letten, A. D., Ke, P. J., and Fukami, T. (2017). Linking modern coexistence theory and contemporary niche theory. Ecol. Monogr. 87, 161–177. doi: 10.1002/ecm.1242
Lukoschek, V., and McCormick, M. I. (2000). “A review of multi-species foraging associations in fishes and their ecological significance,” in Proceedings of the 9th International Coral Reef Symposium, Vol. 1, Bali, 467–474.
MacArthur, R. H. (1958). Population ecology of some warblers of northeastern coniferous forests. Ecology 39, 599–619. doi: 10.2307/1931600
Magrath, R. D., Haff, T. M., Fallow, P. M., and Radford, A. M. (2015). Eavesdropping on heterospecific alarm calls: from mechanisms to consequences. Biol. Rev. 90, 560–586. doi: 10.1111/brv.12122
Mammides, C., Chen, J., Goodale, U. M., Kotagama, S. W., and Goodale, E. (2018). Measurement of species associations in mixed-species bird flocks across environmental and human disturbance gradients. Ecosphere 9:e02324. doi: 10.1002/ecs2.2324
McLean, I. (1984). Feeding association between fantails and saddlebacks: who benefits? N. Z. J. Ecol. 7, 165–168.
Menge, B. A., and Sutherland, J. P. (1976). Species diversity gradients: synthesis of the roles of predation, competition, and temporal heterogeneity. Am. Nat. 110, 351–369. doi: 10.1086/283073
Morse, D. (1970). Ecological aspects of some mixed-species foraging flocks of birds. Ecol. Monogr. 40, 119–168. doi: 10.2307/1942443
Mouritsen, K. N., and Poulin, R. (2003). The mud flat anemone-cockle association: mutualism in the intertidal zone? Oecologia 135, 131–137. doi: 10.1007/s00442-003-1183-x
Nair, G. G., Senthilnathan, A., Iyer, S. K., and Guttal, V. (2019). Fission-fusion dynamics and group-size-dependent composition in heterogeneous populations. Phys. Rev. E 99:032412. doi: 10.1103/PhysRevE.99.032412
Paine, R. T. (1974). Intertidal community structure - Experimental studies on the relationship between a dominant competitor and its principal predator. Oecologia 15, 93–120. doi: 10.1007/BF00345739
Park, T., and Lloyd, M. (1955). Natural selection and the outcome of competition. Am. Nat. 89, 235–240. doi: 10.1086/281887
Peiman, K. S., and Robinson, B. W. (2010). Ecology and evolution of resource-related heterospecific aggression. Q. Rev. Biol. 85, 133–158. doi: 10.1086/652374
Powell, G. V. N. (1985). Sociobiology and adaptive significance of interspecific foraging flocks in the neotropics. Ornithol. Monogr. 36, 713–732. doi: 10.2307/40168313
R Core Team (2018). R: A Language and Environment for Statistical Computing. Vienna: R Foundation for Statistical Computing.
Remsen, J. V., and Robinson, S. K. (1990). A classification scheme for foraging behaviour of birds in terrestrial habitats. Stud. Avian Biol. 13, 144–160.
Ricklefs, R. E. (1987). Community diversity?: relative roles of local and regional processes. Science 235, 167–171. doi: 10.1126/science.235.4785.167
Rusterholz, K. A. (1981). Competition and the structure of an avian foraging guild. Am. Nat. 118, 173–190. doi: 10.1086/283813
Satishchandra, S. K. H., Kudavidanage, E. P., Kotagama, S. W., and Goodale, E. (2007). The benefits of joining mixed-species flocks for greater racket-tailed drongo Dicrurus paradiseus. Forktail 23, 145–148.
Schoener, T. W. (1974). Resouce partitioning in ecological communities: research on how similar species divide resources helps. Science 185, 27–39.
Schoener, T. W. (1983). Field experiments on interspecific competition. Am. Nat. 122, 240–285. doi: 10.1086/284133
Seppanen, J. T., Monkkonen, M., and Thomson, R. L. (2007). Social information use is a process across time, space, and ecology, reaching heterospecifics. Ecology 88, 1622–1633. doi: 10.1890/06-1757.1
Sfenthourakis, S., Tzanatos, E., and Giokas, S. (2006). Species co-occurrence: the case of congeneric species and a causal approach to patterns of species association. Glob. Ecol. Biogeogr. 15, 39–49. doi: 10.1111/j.1466-822X.2005.00192.x
Sinclair, A. R. E. (1985). Does interspecific competition or predation shape the African ungulate community? J. Anim. Ecol. 54, 899–918. doi: 10.2307/4386
Sridhar, H., Beauchamp, G., and Shanker, K. (2009). Why do birds participate in mixed-species foraging flocks? A large-scale synthesis. Anim. Behav. 78, 337–347. doi: 10.1016/j.anbehav.2009.05.008
Sridhar, H., and Guttal, V. (2018). Friendship across species borders: factors that facilitate and constrain heterospecific sociality. Phil. Trans. R. Soc. B Biol. Sci. 373:20170014. doi: 10.1098/rstb.2017.0014
Sridhar, H., Jordán, F., and Shanker, K. (2013). Species importance in a heterospecific foraging association network. Oikos 122, 1325–1334. doi: 10.1111/j.1600-0706.2013.00101.x
Sridhar, H., and Shanker, K. (2014a). Importance of intraspecifically gregarious species in a tropical bird community. Oecologia 176, 763–770. doi: 10.1007/s00442-014-3045-0
Sridhar, H., and Shanker, K. (2014b). Using intra-flock association patterns to understand why birds participate in mixed-species foraging flocks in terrestrial habitats. Behav. Ecol. Sociobiol. 68, 185–196. doi: 10.1007/s00265-013-1633-3
Sridhar, H., Srinivasan, U., Askins, R. A., Canales-delgadillo, J. C., Chen, C., Ewert, D. N., et al. (2012a). Positive relationships between association strength and phenotypic similarity characterize the assembly of mixed-species bird flocks worldwide. Am. Nat. 180, 777–790. doi: 10.1086/668012
Sridhar, H., Srinivasan, U., Askins, R. A., Delgadillo, J. C. C., Chen, C.-C., Ewert, D. N., et al. (2012b). Data from: positive relationships between association strength and phenotypic similarity characterize the assembly of mixed-species bird flocks worldwide. Dryad, Dataset doi: 10.5061/dryad.th198
Stachowicz, J. J. (2001). Mutualism, facilitation, and the structure of ecological communities. Bioscience 51, 235–246. doi: 10.1641/0006-3568(2001)051[0235:mfatso]2.0.co;2
Struhsaker, T. T. (1981). Polyspecific associations among tropical rain-forest primates. Z. Tierpsychol. 57, 268–304. doi: 10.1111/j.1439-0310.1981.tb01928.x
Toms, J. D. (2013). Linking behavior and community ecology: interspecific aggression provides evidence for competition between a migrant and resident warbler. Ethology 119, 1057–1066. doi: 10.1111/eth.12166
Valiente-Banuet, A., and Verdú, M. (2007). Facilitation can increase the phylogenetic diversity of plant communities. Ecol. Lett. 10, 1029–1036. doi: 10.1111/j.1461-0248.2007.01100.x
Weiher, E., Freund, D., Bunton, T., Stefanski, A., Lee, T., and Bentivenga, S. (2011). Advances, challenges and a developing synthesis of ecological community assembly theory. Philos. Trans. R. Soc. B Biol. Sci. 366, 2403–2413. doi: 10.1098/rstb.2011.0056
Weiher, E., and Keddy, P. (1999). Ecological Assembly Rules: Perspectives, Advances, Retreats. Cambridge: Cambridge University Press.
Wheeler, A. G., and Calver, M. C. (1996). Resource partitioning in an island community of insectivorous birds during winter. Emu 96, 23–31. doi: 10.1071/mu9960023
Wilson, B. J. (1989). A null model of guild proportionality, applied to stratification of a New Zealand temperate rain forest. Oecologia 80, 263–267. doi: 10.1007/bf00380161
Keywords: phenotypic clumping, bird communities, community assembly, group assembly, mixed-species flocks
Citation: Bangal P, Sridhar H and Shanker K (2021) Phenotypic Clumping Decreases With Flock Richness in Mixed-Species Bird Flocks. Front. Ecol. Evol. 8:537816. doi: 10.3389/fevo.2020.537816
Received: 25 February 2020; Accepted: 26 November 2020;
Published: 13 January 2021.
Edited by:
Eric L. Walters, Old Dominion University, United StatesReviewed by:
Matthew Silk, University of Exeter, United KingdomRyan C. Burner, Norwegian University of Life Sciences, Norway
Copyright © 2021 Bangal, Sridhar and Shanker. This is an open-access article distributed under the terms of the Creative Commons Attribution License (CC BY). The use, distribution or reproduction in other forums is permitted, provided the original author(s) and the copyright owner(s) are credited and that the original publication in this journal is cited, in accordance with accepted academic practice. No use, distribution or reproduction is permitted which does not comply with these terms.
*Correspondence: Priti Bangal, pritibangal@gmail.com
†Present address: Priti Bangal, Nature Conservancy Foundation, Mysore, India