Trioecy in the Marine Mussel Semimytilus algosus (Mollusca, Bivalvia): Stable Sex Ratios Across 22 Degrees of a Latitudinal Gradient
- 1Centro de Investigación Marina Quintay (CIMARQ), Universidad Andres Bello, Santiago, Chile
- 2Instituto de Ciencias Marinas y Limnológicas (ICML), Facultad de Ciencias Universidad Austral de Chile, Valdivia, Chile
- 3School of Biological Sciences, Victoria University of Wellington, Wellington, New Zealand
Trioecy, the co-occurrence of males, females and hermaphrodites in natural populations, is a relatively common sexual system in plants, but is rare and poorly understood in animals. Previously, the marine mussel, Semimytilus algosus, has been described as a simultaneous hermaphrodite and there are reports of unisex animals in the wild. Here, we confirm trioecy in this bivalve, the first report of trioecy in the phylum Mollusca. We examined the frequencies of females, males and hermaphrodites in seven natural populations along a latitudinal gradient of ∼2500 km and carried out a phylogenetic analysis with mussel species of the family Mytilidae with known sexual systems. Hermaphrodites (∼95.3%), females (∼3.6%), and males (∼1.1%) were found in all seven populations across the species’ range in Chile. The consistent sex ratios across all populations suggest that the sexual system is stable in space. Phylogenetic analysis (mtDNA) of members of the family Mytilidae indicates that this species alone has developed trioecy from dioecious ancestors. As unisex animals were the least common in the wild, it is likely that these animals, especially males, might be in the process of being lost in this species, and that trioecy might be an intermediate step towards gynodioecy or hermaphroditism. The reproductive characteristics of S. algosus make it an excellent model species for the study of the evolution of sexual systems in animals and also possibly the processes underlying sex determination in molluscs.
Introduction
One of the fundamental challenges of evolutionary biology is to understand breeding systems and to determine how and why different breeding systems exist (Darwin, 1876; Maynard Smith, 1978). Whilst a range of different breeding systems exist amongst the Animalia (reviewed by Weeks, 2012), there are two common sexual systems, dioecy (or gonochorism), in which individuals are either male or female during their reproductive lives but not both, and hermaphroditism, in which animals produce both eggs and sperm during their breeding lives (Avise and Mank, 2009; Leonard, 2010). Most animals are, however, dioecious and it is estimated that hermaphroditism occurs in only 5–6% of species (Jarne and Auld, 2006).
Mixed sexual systems are found in populations that contain a mixture of hermaphrodites and single-sex individuals, such as androdioecy (co-occurrence of males and hermaphrodites), gynodioecy (co-occurrence of females and hermaphrodites) and trioecy (males, female and hermaphrodites). Trioecy in natural populations is a rare and poorly understood sexual system in animals (Weeks, 2012; Armoza-Zvuloni et al., 2014). Two key questions related to breeding systems have long been of interest to evolutionary biologists. First, under what conditions do different sexual systems exist (Ghiselin, 1969; Maynard Smith, 1978)? And second, how stable are such sexual systems or are they simply transition states to another sexual systems (Charnov, 1982; Charlesworth, 1984)? With regard to the first question, a number of different factors have been identified or proposed as being important in promoting the existence of different breeding systems, in particular in plants (Barrett, 2002; Jones et al., 2013; Petry et al., 2016; Ansaldi et al., 2018), sometimes within populations of the same species in close geographic proximity. For example, Fleming et al. (1998) describe an example in the Sonoran Desert columnar cactus (Pachycereus pringlei) where trioecy occurs near the roosts of its major pollinator, the bat Leptonycteris curasoae, whilst gynodioecy occurs >50 km away from roosts. The abundance of the bat pollinators is suggested to play a critical role in the maintenance of this mixed sexual system. Significantly, in particular with regard to the second point, Fleming et al. (1998) note that trioecy can be a stable sexual system in this cactus when bat pollinators are limited in their abundance.
In their review of mixed sexual systems (which did not include trioecy) Eppley and Jesson (2008) tested the hypothesis that an organism’s ability to move to find a mate influences its mating system (e.g., Ghiselin, 1969; Heath, 1977; Charnov, 1979; Puurtinen and Kaitala, 2002). They showed that taxa with well developed mate search capabilities tend to have separate sexes, whereas sessile taxa and those species with poorly developed mate search capabilities tend to exhibit increased hermaphroditism. Thus, sessile organisms such as plants and many marine invertebrates may be expected to exhibit greater levels of hermaphroditism than motile organisms. Following from this, it is often considered that different reproductive strategies appear during the evolutionary transition between dioecy and hermaphroditism or vice versa (Charlesworth, 2006; Weeks, 2012; Weeks et al., 2014a, b). This sort of transition may have occurred multiple times and is better understood in plants than in animals (Weiblen et al., 2000; Weeks, 2012). Unlike in plants, transitions in animals are mostly from dioecious systems to hermaphrodites (Ghiselin, 1969; Eppley and Jesson, 2008; Weeks, 2012; Sasson and Ryan, 2017). This reproductive transition has received less attention both theoretically and empirically (Ghiselin, 1974; Charnov, 1982; Weeks, 2012), probably due to the lack of plausible models that allow for hypothesis testing. Therefore, the study of populations of a species where hermaphrodite and unisex animals co-occur is crucial to aid our understanding not only of the reproductive dynamics of the species, but also the evolution of the sexual system.
In bivalve molluscs, the presence of separate sexes (dioecy) is the most frequent reproductive strategy (Collin, 2013; Gosling, 2015). Only 9% of species exhibit hermaphroditism (Heller, 1993), and these are mainly associated with certain groups (e.g., oysters of the genera Ostrea and Crassostrea). However, hermaphroditic individuals have been found in species that are considered to be strictly dioecious (Ghiselin, 1969; Heller, 1993). For example, there are reports of hermaphroditic animals in some populations of mytilids (true mussels), but they usually correspond to isolated cases that represent <<1% of the population (Vinuesa, 1977; Saavedra et al., 1997; Alfaro et al., 2001; Barber et al., 2005; Montenegro et al., 2010; Al-Barwani et al., 2013; Abdel Razek et al., 2017; Torroglosa and Giménez, 2019). Whilst these occurrences of hermaphrodites amongst the Bivalvia may be natural but very rare, in other instances the phenomenon of hermaphroditism has been attributed to ecotoxic events (Nice et al., 2003; Ciocan et al., 2012; Dublinowska et al., 2016) or drastic population fluctuations (Adjei-Boateng and Wilson, 2016), which probably alter the mechanism of sexual differentiation (Yusa, 2009). However, only a few studies have performed an in-depth analysis of the causes behind the appearance of bisexual animals in dioecious species (Morton, 1991; Romo-Piñera et al., 2009; Dublinowska et al., 2016).
Semimytilus algosus (Gould 1850) is a marine bivalve mollusc belonging to the family Mytilidae, which inhabits the lower intertidal zone of the Pacific coastline of South America (Broitman et al., 2001; Navarrete et al., 2008). It has a wide distribution, from Manta, Ecuador (0°56′S) to Maicolpue, Chile (40°35′S) (personal observation, Pablo Oyarzún). González et al. (1980) first described the hermaphroditism of S. algosus from Concepción, near the Bíobío river, Chile (36°48′S), and reported that gonad tissue is located in two compartments: on one side of the mantle there is an intense yellow tissue corresponding to the male tissue, and on the opposite side there is the female tissue that has a dark brown colour. Subsequently, Garrido (1996) analysed the S. algosus population of Mehuín (southern Chile, 39°26′S) and noted that, in addition to hermaphrodites, some individuals exhibited gonadal tissue of a single-sex (i.e., unisex animals). This information, collected from two geographically distinct sites and at different times, raises the possibility that S. algosus has a mixed reproductive system – trioecy – and may therefore represent an opportunity to understand how an unusual reproductive system has evolved in a predominantly dioecious group, the family Mytilidae (true marine mussels). The objectives of the present work were: (1) to test our hypothesis that the mussel, S. algosus, has evolved a sexual system based on trioecy, (2) to examine the spatial stability of the sexual system across multiple independent (non-interbreeding) populations along a natural latitudinal gradient of ∼ 2500 km, and (3) determine the phylogenetic position of this mussel within the broader evolutionary context of the family Mytilidae of the phylum Mollusca, that is known to exhibit dioecy.
Materials and Methods
Sampling Sites
Semimytilus algosus specimens were collected haphazardly from seven intertidal rocky coastal sites in Chile, spanning approximately 22 degrees of latitude and nearly 2500 km (Table 1). In total, 4108 mussels were sampled between February 2017 and March 2018. Population-specific samples were collected in different months, during early spring to late autumn. Field observations indicate that gonads are developing or in spawning condition for most of the year (González et al., 1980) i.e., there is no tightly synchronised period of spawning activity as reported elsewhere for other mytilids. Specimens of Brachidontes rodriguezii (from Las Grutas, Argentina), Choromytilus chorus (from Colún, Chile), Mytilus chilensis (from Quillaipe, Chile) and Perumytilus purpuratus (from Calfuco, Chile) were also collected for molecular analysis only (details in subsequent section). This was to permit comparison of the sexual system type of S. algosus (a member of the family Mytilidae) to the reproductive strategies that these mytilid species all possess (dioecy), given the relatively close evolutionary affinities of all of the species. All mussels were kept alive in seawater at low temperature during their transfer to the Systematics Laboratory of the Instituto de Ciencias Marinas y Limnológicas (ICML) at the Universidad Austral de Chile, Valdivia.
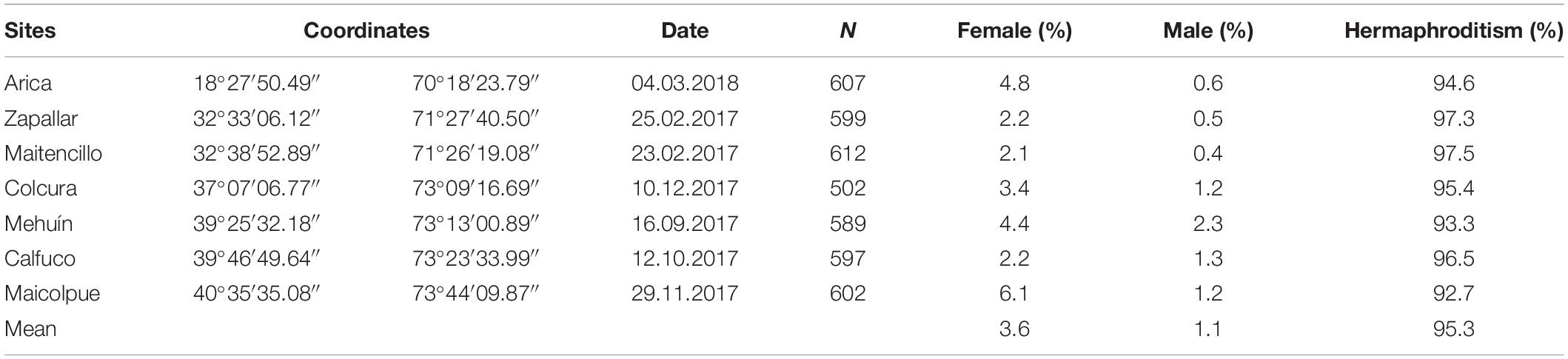
Table 1. Site survey information in Chile, number of Semimytilus algosus specimens collected (N), date of collection, geographical coordinates of sites and percentage of males, females and hermaphrodites.
Gonad Dissection, Histological Procedure and Analyses
All 4108 S. algosus mussels were dissected to analyse the gonad tissue (Table 1). Gonad was carefully removed from the shell; gonadal smears (Torrado and Mikhailov, 1998) and microscopic observation of germ cells were performed in the 4108 mussels analysed. Gonad colouration (females = brown, males = yellow – González et al., 1980) was also recorded in order to test this phenotypic characteristic.
For histological procedures, 20 hermaphroditic mussels per site were examined. All unisex animals (male: 2–14; female: 13–37 per sites) were also included in the histological procedure (see Table 1). A piece of the middle region of the gonad of each specimen was extracted and fixed in 10% formalin for 10 days. Alcoholic dehydration, inclusion, sectioning (7 μm) and staining (hematoxylin-eosin) were performed according to Oyarzún et al. (2018). Gonad development was quantified following standard terminology as applied to bivalve molluscs. Individual mussels were sexed (male, female, hermaphrodite) and sex ratios calculated for each of the seven populations.
A one-way ANOVA was performed to assess whether there were differences in maximum length (larger size = older age – Gray et al., 1997) with respect to the reproductive category (hermaphrodites, males and females) to assess whether sexual categories were related to or independent of size. Normality was tested using the Kolmogorov–Smirnov test corrected by Lilliefors; Levene’s test was used to test for equality of variances. A statistical power test was also performed. The χ2 test of independence (3 × 7 matrix) was used to assess whether the frequencies of hermaphrodites, females and males varied amongst sites. These analyses were carried out using the “corrplot” package implemented in R-project (R Development Core Team, 2008).
DNA Extraction, Amplification, Sequencing, and Alignment
Each S. algosus mussel was dissected and a small piece of mantle edge tissue was preserved in 95% ethanol and stored at 4°C. Approximately 25 mg of mantle tissue was isolated from each individual and total genomic DNA was extracted using a genomic E.Z.N.A.® Tissue DNA kit according to the manufacturer’s instructions. Partial cytochrome c oxidase subunit I (COI) mitochondrial gene sequence was amplified using the primers SemMytF1/HCO2198 (De Greef et al., 2014) for S. algosus, CO1aF/CO1aR (Trovant et al., 2013) for P. purpuratus, and LCO1490/HC02198 (Folmer et al., 1994) for the other three species. Standard PCR amplifications were carried out in 25 μL reaction mixtures containing 2 μL template DNA, 0.2 mM total dNTP, 2 mM MgCl2, 0.4 mM of each primer, 1U of Taq (InvitrogenTM), the manufacturer-supplied PCR buffer, and sterile distilled water. The PCR conditions involved an initial denaturation step at 93°C for 3 min, followed by 35 cycles at 93°C for 30 s, annealing at 52°C for 30 s (S. algosus 42°C × 30 s) and elongation at 72°C for 1 min, followed by further elongation at 72°C for 5 min.
All PCR amplifications products were scored on 2% agarose gels stained with SYBR® Safe DNA and photographed under a blue-light transilluminator (InvitrogenTM). For every gel, the sizes of amplified fragments were estimated from a 100 bp DNA ladder (InvitrogenTM). Amplicons were purified and sequenced by Macrogen (Seoul, South Korea). Both sequence directions were determined, using the individual primers from the original reaction. The DNA sequence (601 bp in length) was edited using Geneious® 11.0.4 (Biomatters Ltd., Auckland, New Zealand). All new sequences were deposited in GenBank under accession numbers MT103127-MT103143. Sequences of additional mytilids were obtained from GenBank (Supplementary Table S1). All nucleotide sequences were aligned using MAFFT v.7 (Katoh and Standley, 2013) under the iterative method of global pairwise alignment (G-INS-i) (Katoh et al., 2005), and default settings were chosen for all parameters.
Phylogenetic Analyses
Phylogenetic trees were constructed using Maximum Likelihood (ML) and Bayesian inference (BI). Evolutionary models and partitioning strategies were evaluated with PartitionFinder v2.1.1 (Lanfear et al., 2017), which chose the best partition under the BIC criterion (Schwarz, 1978). A ML tree was inferred using GARLI v2.0 (Bazinet et al., 2014) with branch support being estimated by non-parametric bootstrap (200 replicates). Bayesian analyses were performed using MrBayes v3.2 (Ronquist et al., 2012). Each Markov chain was started from a random tree and run for 5.0 × 107 generations with every 1000th generation sampled from the chain. Stationarity was checked as suggested in Nylander et al. (2004). All sample points prior to reaching the plateau phase were discarded as “burn-in”, and the remaining trees were combined to find the a posteriori probability of phylogeny. Analyses were repeated four times to confirm that they all converged on the same results. Posterior probability values > 0.90 were taken as statistical support for a clade being present on the true tree (Huelsenbeck and Rannala, 2004).
Results
Gonadal Analysis of S. algosus
In total, 4108 specimens of S. algosus with a shell length range of 11.44–38.70 mm were analysed. We had a probability 0.92 to detect (with threshold p = 0.05) an effect size of 4.97 with our sample size of n > 75 for each of three groups. The one-way ANOVA showed no significant differences amongst reproductive category type (hermaphrodites, males and females) as a function of mussel size (F[2,1402] = 2.776; P > 0.05) (see size frequency – Supplementary Figure S2). S. algosus exhibited typical gonadal characteristics of mussels, with the two gonadal lobes (one on the left and one on right) containing almost all of the gonad. In hermaphroditic animals, one mantle lobe was male gonad (yellow) whilst the other lobe was female (dark brown), so the gonad was separated into a male and female portion (Figure 1), in all cases. The histological sections and the smears corroborated that tissue coloration is a sexual characteristic (Figure 1).
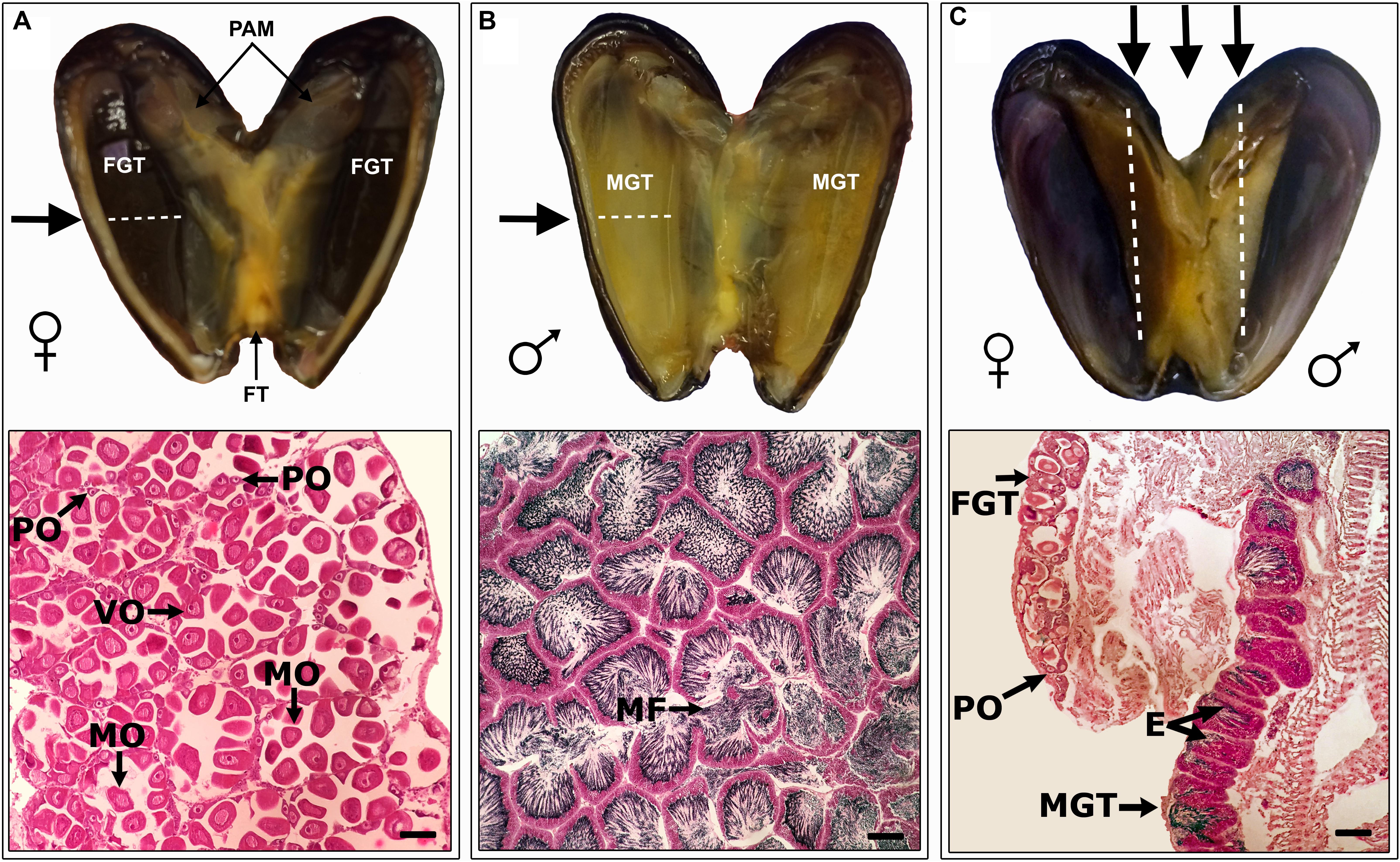
Figure 1. Examples of Semimytilus algosus mantle tissue coloration. Transverse section showing detail of (A – top panel) female and (B – top panel) male follicle in the mantle lobe. Longitudinal section (C – top panel) showing the details of an hermaphroditic individual. The arrows indicate the direction of the histological section. Bottom panels A-C show micrographs of histological sections (haematoxylin-eosin staining) of S. algosus, for a female, male and an hermaphrodite. FGT: Female Gonadal Tissue, MGT: Male Gonadal Tissue, MF: Male follicle, PO: Previtellogenic oocyte, VO: Vitellogenic oocyte, MO: Mature oocyte, and E: spermatozoa in the lumen, FT: Foot and PAM: Posterior Adductor Muscle. Scale bar (bottom right of each lower panel): A and B = 100 μm, C = 500 μm.
The gonads from unisex animals did not exhibit gametogenic differences compared to the hermaphroditic animals. In the female gonadal tissue, oocytes were connected to the follicular wall and were previtellogenic and/or vitellogenic (Figure 1A). Male tissue exhibited radially arranged spermatozoa in the lumen (Figure 1B). In both males and females, little connective tissue was observed (Figure 1).
Sex Ratios
Unisex animals (males and females) and simultaneous hermaphrodites were found at all sites (Table 1). Significant differences were observed in the frequencies of hermaphrodites, females and males amongst populations (χ2 = 41.967; df = 12; P-value < 0.05). However, these differences were strongly influenced by the values obtained for males from Mehuín (2.3%) and females from Maicolpue (6.1%) (refer to Supplementary Figure S1). Hermaphrodites occurred at greatest abundance in all populations (92.7–97.5%), females corresponded to 2.1–6.1% of the mussels, and males occurred at the lowest frequency in all populations (0.4–2.3%) (Table 1).
Phylogenetic Relationships
Testing for the best fit substitution model resulted in the selection of models TrN + I + G (1st, best fit), TVM + G (2nd) and TrN + G (3rd) for COI. The BI and ML analyses revealed almost identical topologies with two major clades strongly supported (Figure 2). Clade 1 included the subfamilies Arcuatulinae, Crenellinae, Musculinae, and Mytilinae. Clade 2 included S. algosus and members of the subfamily Brachidontinae (Mytilaster, Brachidontes, Ischadium, Geukensia, and Perumytilus) (Figure 2 and Supplementary Table S1), and within Clade 2, Brachidontes rodriguezii was sister to the rest of the genera. The clustering of S. algosus (trioecy) with P. purpuratus (dioecy) was strongly supported (a posteriori probability = 1.0; bootstrap support of 99%; Figure 2). Based on our phylogenetic reconstruction, S. algosus is placed for the first time as part of the monophyletic subfamily Brachidontinae.
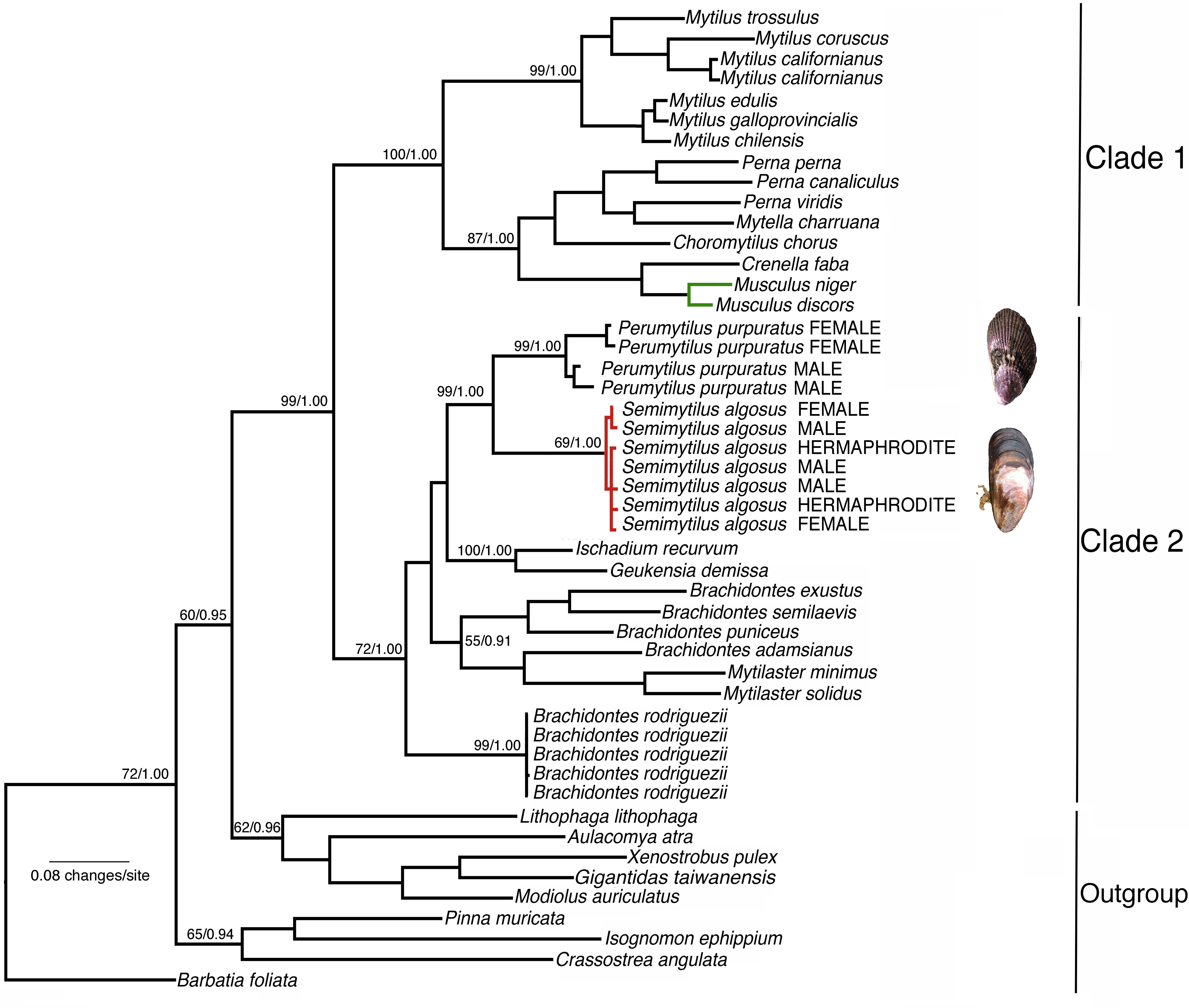
Figure 2. Maximum likelihood tree of the relationships amongst mussels of the family Mytilidae based on cytochrome oxidase subunit I (COI) sequence variation (Bayesian analysis returned the same topology). Numbers above the branches are maximum likelihood bootstrapping scores/Bayesian posterior probabilities. Green = hermaphrodite species; red = trioecy species. The GenBank accession numbers are given in Supplementary Table S1.
Discussion
Building on earlier reports of an unusual, but unconfirmed type of sexual system (González et al., 1980; Garrido, 1996), we report here, for the first time, the occurrence of trioecy in the South American intertidal marine mussel, S. algosus, which is also the first report of trioecy amongst members of the phylum Mollusca, the second-most speciose animal phylum (Kershaw, 1983). Animal trioecy is extremely rare, and, to the best of our knowledge, examples are limited to the sea anemones Aiptasia diaphana (Armoza-Zvuloni et al., 2014) and Bartholomea annulata (Jennison, 1981), the green hydra Hydra viridissima (Kaliszewicz, 2011) and the nematodes Auanema rhodensis, A. freiburgensis (Kanzaki et al., 2017) and Rhabditis sp. SB347 (Chaudhuri et al., 2011, 2015). Sex ratios differ considerably from one case to another. This can make it difficult to search for new cases of sexual trimorphism, because trioecy can be confused with the intersex state. This is a topic that needs further examination, although given the relatively rare occurrence of trioecy this may not be easy to achieve. Trioecy in animals is thought to be so rare because it represents an evolutionary unstable step from one mating system to another (Leonard, 2018), but this does not completely explain its greater rarity in animals than in plants.
The Trioecy of S. algosus
Analysis of gonad tissue from seven geographically distinct populations of the marine mussel, S. algosus, has identified the co-occurrence of hermaphrodites, females and males. In stark contrast to other reports of trioecy amongst animals where it is usually reported that hermaphrodites are scarce and/or exhibit abnormal development (Greve, 1996; Labruna et al., 2002; Maeno and Tanaka, 2007; Yoshizawa et al., 2009; Armoza-Zvuloni et al., 2014; Chaudhuri et al., 2015; Kanzaki et al., 2017), in S. algosus the hermaphroditic animals were the most abundant sex, whilst the unisex individuals combined represented ∼5% of each population. The gonadal tissues of the three sexual phenotypes showed normal gametogenic development, i.e., the presence of follicles with cells at different stages of development. Both male and female gametes had characteristics similar to the germ cells described in other mytilid species (González et al., 1980; Toro et al., 2002; Oyarzún et al., 2010, 2011).
One of the most striking points from our results is the relative constancy of the ratio of the three sexes across the seven populations, that span more than 22 degress of latitude, a distance of ∼2500 km, from the subtropical north to the cool temperate south of Chile. Across all populations, regardless of environment, the ratio of hermaphrodites:females:males was remarkably similar, at a mean of 95.3:3.6:1.1. This suggests that the trioecious state in S. algosus is stable over a large part of the range of the species. Our results are in contrast to those for others species, where it has been reported how factors such as pollinator density or environmental variation can influence the sexual system of a species, even over reasonably small spatial scales (Fleming et al., 1998; Barrett, 2002; Jones et al., 2013; Petry et al., 2016; Ansaldi et al., 2018). In addition, we rule out the possibility of S. algosus shows a different size in each reproductive category (ANOVA reveals that sexual category is independent of shell length, when length is a surrogate for age), as has been observed in other molluscs (e.g., Ostrea chilensis – DiSalvo et al., 1983).
Hermaphroditism in S. algosus
Hermaphroditism is a rarity amongst the species of the family Mytilidae (see Supplementary Table S1). Mytilid hermaphrodites are considered to be an exception produced by some abnormality in the determination of sex (Yusa, 2009; Dublinowska et al., 2016). However, the hermaphroditic individuals of S. algosus are expected to be the main sexual phenotype involved in a population’s reproductive output given that they are the most abundant group by far.
Species with simultaneous hermaphroditism are subject to the probability of self-fertilisation (Liu et al., 2011). The hypothesis of “reproductive assurance” suggests that self-fertilisation occurs because animals that are capable of reproducing on their own ensure the persistence of the population in environments where mixed sex individuals are not available (Theologidis et al., 2014). It has, however, also been suggested that self-fertilisation may be associated with low reproductive potential and, therefore, with a loss of biological fitness (Charlesworth and Charlesworth, 1987). As a newly-reported invasive species in South Africa, S. algosus exhibits a high reproductive output and a high recruitment rate, factors that are likely to have contributed to its invasion success (De Greef et al., 2014; Zeeman et al., 2018). These reproductive traits are believed to have given S. algosus an advantage over the endemic mytilids, Choromytilus meridionalis and Aulacomya atra, both of which are dioecious, resulting in a stable coexistence of the three sexual phenotypes for S. algosus and suggesting that S. algosus has an effective mechanism to avoid self-fertilisation (see later).
The gonads of S. algosus contained female and male gametes that were located independently. This gametic organisation is the most common form reported in hermaphroditic molluscs – it is known as a type I gonad (Sastry, 1979; Soria et al., 2002). The localisation of the gonads in separate compartments is probably a reproductive strategy to avoid self-fertilisation because the animal can control gametic emissions (spawning activity) independently (personal observation – P. Oyarzún). This suggestion is supported by the fact that Garrido (1996) observed an asynchrony in the spawning of the male and female gametes of the hermaphroditic mussels of S. algosus. Thus, S. algosus appears to employ a pre-zygotic mechanism (i.e., spawning asynchrony, possibly under direct control of gamete release) rather than a post-zygotic mechanism (e.g., some form of larval developmental incompatibility) to prevent self-fertilisation.
Trioecy as an Unstable Transitional State in Sexual Systems
It has been suggested that trioecy is an unstable state in a transition to another state such as dioecy, androdioecy or gynodioecy (Maurice and Fleming, 1995; Wolf and Takebayashi, 2004; Leonard, 2018). Supporting evidence for this suggestion has been obtained from experimental work on Caenorhabditis elegans that reverts to androdioecy after only a few generations (Stewart and Phillips, 2002; Cutter, 2005). However, the transition from dioecy to hermaphroditism, one of the reproductive states required to achieve trioecy in S. algosus, has had less attention than other changes (Weeks, 2012), despite having evolved several times within animals (Eppley and Jesson, 2008; Sasson and Ryan, 2017).
The transition from dioecious to hermaphrodite has been more common than vice versa in animals (Sasson and Ryan, 2017), although the transition mechanism is not well understood. There is evidence that androdioecious species (hermaphrodites + males) have evolved from dioecious species (Weeks et al., 2014b). Therefore, androdioecy is expected to be an intermediate reproductive system that emerges in the transition from dioecy to hermaphroditism. Such a transition may begin with the gain of an alternative sex in a unisex individual (male or female), then this mutant hermaphrodite is expected to invade the population (Ghiselin, 1974; Charnov, 1982; Weeks, 2012). However, this process is expected to be gradual (Leonard, 2018), suggesting that at the beginning of the transition, from one reproductive system to another, a sexual trimorphism – trioecy – is produced.
The reproduction of marine mussels is strongly rooted in dioecy (Gosling, 2015 and Supplementary Table S1), a reproductive strategy commonly found in most bivalve species (Heller, 1993; Collin, 2013). However, S. algosus shows a mixed reproductive system (hermaphrodites + females + males), where hermaphrodites are the most abundant and males the least abundant in each populations (mean value of ∼1.1%). We interpret this as evidence of selection against the male sexual phenotype. This suggests that the factors which regulate female abundance in trioecious populations are independent of the factors that regulate male abundance. Although we do not know how the three sexes can coexist, we speculate that the trioecy of S. algosus is a transient reproductive system close to gynodioecy (transition hypothesis = dioecy trioecy gynodioecy hermaphroditism). Further investigations are needed on the interaction between males, females and hermaphrodites in populations to address the causes that promote the transitions from one sexual system to another.
Interactions Between Ecology, Life-History Characteristics, and Sexual System
It has been suggested that key life-history characteristics such as motility (or lack of it), population density, and environmental variation may all affect a species’ breeding system. In many respects, S. algosus is a “typical” marine intertidal mussel. It is sessile as an adult, it occurs at high densities in wild populations (Broitman et al., 2001; Navarrete et al., 2008; Zeeman et al., 2018), and population numbers are very large (100,000s to millions of individuals). It co-occurs with other mytilid species such as Perumytilus purpuratus and Mytilus chilensis, both of which are dioecious species. It is tolerant of environmental variation, given that it occurs over ∼40 degrees of latitude. Despite analyses pointing to the importance of factors such as motility versus a sessile life form or breeding density within a population, which has relevance to gamete interactions (Eppley and Jesson, 2008; Avise and Mank, 2009), there is no obvious reason why S. algosus has evolved trioecy as a sexual system when all of its close relatives within the family Mytilidae have evolved and retained dioecy.
The Evolutionary Origin of Trioecy in S. algosus
The phylogenetic analysis performed in this study produced stable topologies that are congruent with other studies that use multiple genes (e.g., Trovant et al., 2015). Although the monophyly of the established subfamilies is not supported (specifically the Mytilinae subfamily), our results indicate for the first time that S. algosus is a member of the Brachidontinae subfamily. We are aware of the limitations of using only a single mitochondrial marker. However, mtDNA data has been very helpful in understanding the phylogenetic relationships in the Mytilidae (e.g., Hilbish et al., 2000; Terranova et al., 2007; Gérard et al., 2008). In fact, the largest genetic database for this group comes from mtDNA sequences (mainly COI and 16S). For this reason, we consider the use of COI was appropriate for our study, but we recognise that other markers and/or a greater number of markers may have yielded slightly different, but probably qualitatively similar, results.
Phylogenetic analysis of the mitochondrial DNA cytochrome c oxidase subunit I (COI) region indicates that S. algosus is closely related to dioecious species (e.g., P. purpuratus – within clade 2), and also reveals the independent evolution of sequential hermaphroditism (but not trioecy) in the bivalve genus Musculus (within clade 1). The question remains, however, why has trioecy developed in S. algosus, when other marine mussels with very similar ecologies and life-history characteristics are dioecious? We do not know the answer to this question (yet), but we speculate that it may be related to the mechanism of sex determination. For example, in Rhabditis nematodes, sex is genetically determined with males being XY and females XX; if XX larvae are exposed to environmental conditions that produce a dauer larva, they become simultaneous hermaphrodites as adults (Chaudhuri et al., 2015; Kanzaki et al., 2017). Therefore, environmental sex determination and genetic sex determination interact in this example of trioecy. Although there are several studies on the determination of sex in bivalves (reviewed by Breton et al., 2017), several aspects of the mechanism(s) involved remain unknown. The evolution of the sexes and/or origin of hermaphroditism are topics that researchers have struggled to resolve (i.e., Baldi et al., 2009; Geng et al., 2014), and these efforts have still not been sufficient to answer critical questions. S. algosus as a new model species may help shed new light on the evolution of sexual systems in general, and specifically in the Mollusca.
Data Availability Statement
This manuscript contains previously unpublished data. The access numbers of the molecular data (sequences) are available - MT103127–MT103143 (GenBank) and the graphical representation of the data can be found in the Supplementary Material.
Ethics Statement
This study was carried out in accordance with the principles of the Basel Declaration and recommendations of Universidad Austral de Chile committee. The protocol was approved by the biosafety institution of the Universidad Austral de Chile (No008/17). The animals involved in this study were minor invertebrates (Mollusca: Bivalves: Mytilidae).
Author Contributions
PO and JN conceived and designed the study, carried out the fieldwork and preparation of the manuscript. JT and JG conducted the analysis of the results and helped with preparing the final draft. All authors reviewed the manuscript.
Funding
This study was funded by the project FONDECYT 3170521 (PO) and FONDECYT 1170194 (JT).
Conflict of Interest
The authors declare that the research was conducted in the absence of any commercial or financial relationships that could be construed as a potential conflict of interest.
Acknowledgments
The authors thank Marcela Riveros, Valeska Vásquez, Felipe Toledo, Alex Illesca, and Carolina Briones for their support in the field and laboratory.
Supplementary Material
The Supplementary Material for this article can be found online at: https://www.frontiersin.org/articles/10.3389/fmars.2020.00348/full#supplementary-material
References
Abdel Razek, F. A., El-Deeb, R. S., Abdul-Aziz, K. K., Omar, H. A., and Khfage, A. R. (2017). Hermaphroditism in Brachidontes pharaonis (Fischer, 1876) (Bivalvia: Mytilidae) from the Alexandria Coast, Egypt. Egyp. J. Aquat. Res. 43, 265–268. doi: 10.1016/j.ejar.2017.10.002
Adjei-Boateng, D., and Wilson, J. G. (2016). Sexual strategy in the freshwater bivalve Galatea paradoxa (Donacidae) from the Volta River estuary, Ghana. Mol. Res. 36, 1–8. doi: 10.1080/13235818.2015.1054021
Al-Barwani, S. M., Arshad, A., Amin, S. M. N., and Bujang, J. S. (2013). Incidence of hermaphrodite in green mussel Perna viridis along the West Coast of Peninsular Malaysia. Asian J. An. Vet. Adv. 8, 376–382. doi: 10.3923/ajava.2013.376.382
Alfaro, A. C., Jeffs, A. G., and Hooker, S. H. (2001). Reproductive behaviour of the green-lipped mussel, Perna canaliculus, in northern New Zealand. Bull. Mar. Sci. 69, 1095–1108.
Ansaldi, B. H., Franks, S. J., and Weber, J. J. (2018). The influence of environmental factors on breeding system allocation at large spatial scales. AoB Plants 6:ly069. doi: 10.1093/aobpla/ply069
Armoza-Zvuloni, R., Kramarsky-Winter, E., Loya, Y., Schlesinger, A., and Rosenfeld, H. (2014). Trioecy, a unique breeding strategy in the sea anemone Aiptasia diaphana and its association with sex steroids. Biol. Repr. 90:122. doi: 10.1095/biolreprod.113.114116
Avise, J. C., and Mank, J. E. (2009). Evolutionary perspectives on hermaphroditism in fishes. Sex. Dev. 3, 152–163. doi: 10.1159/000223079
Baldi, C., Cho, S., and Ellis, R. E. (2009). Mutations in two independent pathways are sufficient to create hermaphroditic nematodes. Science 326, 1002–1005. doi: 10.1126/science.1176013
Barber, B. J., Fajans, J. S., Baker, S. M., and Baker, P. (2005). Gametogenesis in the non-native green mussel, Perna viridis, and the native scorched mussel, Brachidontes exustus, in Tampa Bay, Florida. J. Shel. Res. 24, 1087–1095.
Barrett, S. C. H. (2002). The evolution of plant sexual diversity. Nat. Rev. Genet. 3:274. doi: 10.1038/nrg776
Bazinet, A. L., Zwickl, D. J., and Cummings, M. P. (2014). A gateway for phylogenetic analysis powered by grid computing featuring GARLI 2.0. Syst. Biol. 63, 812–818. doi: 10.1093/sysbio/syu031
Breton, S., Capt, C., Guerra, D., and Stewart, D. (2017). Sex determining mechanisms in bivalves. Preprints 2017:2017060127. doi: 10.20944/preprints201706.0127.v1
Broitman, B. R., Navarrete, S. A., Smith, F., and Gaines, S. D. (2001). Geographic variation of southeastern Pacific intertidal communities. Mar. Ecol. Prog. Ser. 224, 21–34. doi: 10.3354/meps224021
Charlesworth, D. (1984). Androdioecy and the evolution of dioecy. Biol. J. Lin. Soc. 22, 333–348. doi: 10.1111/j.1095-8312.1984.tb01683.x
Charlesworth, D. (2006). Evolution of plant breeding systems. Curr. Biol. 16, R726–R735. doi: 10.1016/j.cub.2006.07.068
Charlesworth, D., and Charlesworth, B. (1987). Inbreeding depression and its evolutionary consequences. An. Rev. Ecol. Syst. 18, 237–268. doi: 10.1146/annurev.es.18.110187.001321
Chaudhuri, J., Bose, N., Tandonnet, S., Adams, S., Zuco, G., Kache, V., et al. (2015). Mating dynamics in a nematode with three sexes and its evolutionary implications. Sci. Rep. 5:17676.
Chaudhuri, J., Kache, V., and Pires-daSilva, A. (2011). Regulation of sexual plasticity in a Nematode that produces males, females, and hermaphrodites. Curr. Biol. 21, 1548–1551. doi: 10.1016/j.cub.2011.08.009
Ciocan, C. M., Cubero-Leon, E., Peck, M. R., Langston, W. J., Pope, N., Minier, C., et al. (2012). Intersex in Scrobicularia plana: transcriptomic analysis reveals novel genes involved in endocrine disruption. Env. Sci. Tech. 46, 12936–12942. doi: 10.1021/es302948g
Collin, R. (2013). Phylogenetic patterns and phenotypic plasticity of molluscan sexual systems. Int. Com. Biol 53, 723–735. doi: 10.1093/icb/ict076
Cutter, A. D. (2005). Mutation and the experimental evolution of outcrossing in Caenorhabditis elegans. J. Evol. Biol. 18, 27–34. doi: 10.1111/j.1420-9101.2004.00804.x
Darwin, C. R. (1876). The Effects of Cross and Self-fertilization in the Vegetable Kingdom. London, UK: John Murray.
De Greef, K., Griffiths, C. L., and Zeeman, Z. (2014). Deja vu? A second mytilid mussel, Semimytilus algosus, invades South Africa’s west coast. Afr. J. Mar. Sci. 35, 307–313. doi: 10.2989/1814232X.2013.829789
DiSalvo, L. H., Alarcon, E., and Martinez, E. (1983). Induced spat production from Ostrea chilensis Philippi 1845 in mid-winter. Aquaculture 30, 357–362.
Dublinowska, M., Smolarz, K., Zabrzańska, S., Larsson, J., and Czerniawska, J. (2016). Intersexuality in the blue mussel Mytilus edulis complex (Mytilidae) from the Baltic Sea and the Danish strait. Am. Mal. Bull. 34, 1–12. doi: 10.4003/006.034.0105
Eppley, S. M., and Jesson, L. K. (2008). Moving to mate: the evolution of separate and combined sexes in multicellular organisms. J. Evol. Biol. 21, 727–736. doi: 10.1111/j.1420-9101.2008.01524.x
Fleming, T. H., Maurice, S., and Hamrick, J. L. (1998). Geographic variation in the breeding system and the evolutionary stability of trioecy in Pachycereus pringlei (Cactaceae). Evol. Ecol. 12, 279–289.
Folmer, O., Black, M. B., Hoeh, W., Lutz, R., and Vrijenhoek, R. (1994). DNA primers for amplification of mitochondrial Cytochrome C oxidase subunit I from diverse metazoan invertebrates. Mol. Mar. Biol. Bio. 3, 294–299.
Garrido, O. (1996). Biología Comparada de la Reproducción en Molluscos Bivalvos Mytilidae. Ph.D. thesis, Universidad Austral de Chile, Valdivia.
Geng, S., De Hoff, P., and Umen, J. G. (2014). Evolution of sexes from an ancestral mating-type specification pathway. PLoS Biol. 12:e1002055. doi: 10.1371/journal.pbio.1001904
Gérard, K., Bierne, N., Borsa, P., Chenuil, A., and Feral, J. (2008). Pleistocene separation of mitochondrial lineages of Mytilus spp. mussels from Northern and Southern Hemispheres and strong genetic differentiation among southern populations. Mol. Phy. Evol. 49, 84–91.
Ghiselin, M. T. (1974). The Economy of Nature and the Evolution of Sex. Berkeley, CA: University of California Press.
González, J., Villagra, J., Hinojosa, M., and Becerra, R. (1980). Potencialidad del cultivo en balsa de Semimytilus algosus (Gould 1850), en la bahía de Concepción (Mollusca, Bivalvia, Mytilidae). Bol. Inst. Paulista Ocean 29, 187–189. doi: 10.1590/S0373-55241980000200038
Gosling, E. (2015). “Reproduction, settlement and recruitment,” in Marine Bivalve Molluscs, ed. E. Gosling (Hoboken, NJ: John Wiley & Sons, Ltd), 157–202.
Gray, A. P., Seed, R., and Richardson, C. A. (1997). Reproduction and growth of Mytilus edulis chilensis from the Falkland Islands. Sci. Mar. 61, 39–48.
Greve, J. H. (1996). An anomaly (Gynandromorphism) in Abbreviata sp. (Nematoda: Physalopteridae). J. Helminthol. Soc. Washington 63, 261–262.
Heath, D. J. (1977). Simultaneous hermaphroditism-cost and benefit. J. Theor. Biol. 64, 363–373. doi: 10.1016/0022-5193(77)90363-0
Heller, J. (1993). Hermaphroditism in Molluscs. Biol. J. Linn. Soc. 48, 19–42. doi: 10.1006/bijl.1993.1003
Hilbish, T. J., Mullinax, A., Dolven, S. I., Meyer, A., Koehn, R. K., and Rawson, P. D. (2000). Origin of the antitropical distribution pattern in marine mussels (Mytilus spp): routes and timing of transcuatorial migration. Mar. Biol. 136, 69–77.
Huelsenbeck, J. P., and Rannala, B. (2004). Frequentist properties of bayesian posterior probabilities of phylogenetic trees under simple and complex substitution models. Syst. Biol. 53, 904–913. doi: 10.1080/10635150490522629
Jarne, P., and Auld, J. R. (2006). Animals mix it up too: the distribution of self-fertilization among hermaphroditic animals. Evolution 60, 1816–1824. doi: 10.1111/j.0014-3820.2006.tb00525.x
Jennison, B. (1981). Reproduction in three species of sea anemones from Key West, Florida. Can. J. Zool. 59, 1708–1719.
Jones, N., Husband, B., and MacDougall, A. (2013). Reproductive system of a mixed-mating plant responds to climate perturbation by increased selfing. Proc. R. Soc. Lond. B Biol. Sci. 280:20131336. doi: 10.1098/rspb.2013.1336
Kaliszewicz, A. (2011). Interference of asexual and sexual reproduction in the green hydra. Ecol. Res. 26, 147–152.
Kanzaki, N., Kiontke, K., Tanaka, R., Hirooka, Y., Schwarz, A., Müller-Reichert, T., et al. (2017). Description of two three-gendered nematode species in the new genus Auanema (Rhabditina) that are models for reproductive mode evolution. Sci. Rep. 7:11135.
Katoh, K., Kuma, K., Toh, H., and Miyata, T. (2005). MAFFT version 5: improvement in accuracy of multiple sequence alignment. Nuc. Aci. Res. 33, 511–518. doi: 10.1093/nar/gki198
Katoh, K., and Standley, D. M. (2013). MAFFT multiple sequence alignment software version 7: improvements in performance and usability. Mol. Biol. Evol. 30, 772–780. doi: 10.1093/molbev/mst010
Kershaw, D. R. (1983). “Phylum mollusca,” in Animal Diversity, ed. D. R. Kershaw (Chapman & Hall), 169–191.
Labruna, M. B., Ribeiro, A. F., Cruz, M. V., Camargo, L. M. A., and Camargo, E. P. (2002). Gynandromorphism in Amblyomma cajennense and Rhipicephalus sanguineus (Acari:Ixodidae). J. Par. 88, 810–811.
Lanfear, R., Frandsen, P. B., Wright, A. M., Senfeld, T., and Calcott, B. (2017). Partitionfinder 2: new methods for selecting partitioned models of evolution for molecular and morphological phylogenetic analyses. Mol. Biol. Evol. 34, 772–773. doi: 10.1093/molbev/msw260
Leonard, J. L. (2010). “The evolution of sexes, anisogamy, and sexual systems: Natural versus sexual selection,” in The Evolution of Primary Sexual Characters in Animals, eds J. L. Leonard and A. Córdova-Aguilar (Oxford: Oxford University Press), 15–39.
Leonard, J. L. (2018). “The evolution of sexual systems in animals,” in Transitions between Sexual Systems: Understanding the Mechanisms of and Pathways Between, Dioecy, Hermaphroditism and other Sexual Systems, ed. J. L. Leonard (Berlin: Springer).
Liu, J., Liu, Z., and Sun, X. (2011). The effects of inbreeding on production traits of the Southern Bay Scallop Argopecten irradians concentricus. J. Shell. Res. 30, 109–113. doi: 10.2983/035.030.0116
Maeno, K., and Tanaka, S. (2007). Morphological and behavioural characteristics of a gynandromorph of the desert locust, Schistocerca gregaria. Phys. Ent. 32, 294–299. doi: 10.1111/j.1365-3032.2007.00573.x
Maurice, S., and Fleming, T. H. (1995). The effect of pollen limitation on plant reproductive systems and the maintenance of sexual polymorphisms. Oikos 74, 55–60. doi: 10.2307/3545674
Montenegro, V. D., Olivares, P. A., and González, M. T. (2010). Hermaphroditism in marine mussel Perumytilus purpuratus Lamarck, 1819), (Mollusca: Mytilidae). Int. J. Morph. 28, 569–573. doi: 10.4067/S0717-95022010000200038
Morton, B. (1991). Do the bivalvia demonstrate environment-specific sexual strategies? A Hong Kong model. J. Zool. 223, 131–142. doi: 10.1111/j.1469-7998.1991.tb04754.x
Navarrete, S. A., Broitman, B. R., and Menge, B. A. (2008). Interhemispheric comparison of recruitment to rocky intertidal communities: pattern persistence and scales of variation. Ecology 89, 1308–1322. doi: 10.1890/07-0728.1
Nice, H. E., Morritt, D., Crane, M., and Thorndyke, M. (2003). Long- term and transgenerational effects of nonylphenol exposure at a key stage in the development of Crassostrea gigas. Possible endocrine disruption? Mar. Ecol. Prog. Ser. 256, 293–300. doi: 10.3354/meps256293
Nylander, J. A. A., Ronquist, F., Huelsenbeck, J. P., and Nieves-Aldrey, J. L. (2004). Bayesian phylogenetic analysis of combined data. Syst. Biol. 53, 47–67. doi: 10.1080/10635150490264699
Oyarzún, P., Toro, J. E., Jaramillo, R., Guiñez, R., Briones, C., and Astorga, M. (2010). Análisis comparativo del ciclo gametogénico de Perumytilus purpuratus (Lamarck, 1819) (Bivalvia: Mytilidae), en las localidades de Taltal y Huasco, norte de Chile. Rev. Biol. Mar. Ocean 45, 43–58. doi: 10.4067/S0718-19572010000100004
Oyarzún, P. A., Toro, J. E., Garces-Vargas, J., Alvarado, C., Guiñez, R., Jaramillo, R., et al. (2018). Reproductive patterns of mussel Perumytilus purpuratus (Bivalvia: Mytilidae), along the Chilean coast: effects caused by climate change? J. Mar. Biol. Assoc. U. K. 98, 375–385. doi: 10.1017/S0025315416001223
Oyarzún, P. A., Toro, J. E., Jaramillo, R., Guiñez, R., and Briones, C. (2011). Ciclo gonadal del chorito Mytilus chilensis (Bivalvia: Mytilidae) en dos localidades del sur de Chile. L. Am. J. Aquat. Res. 39, 512–525. doi: 10.3856/vol39-issue3-fi1lltext-11
Petry, W. K., Soule, J. D., Iler, A. M., Chicas-Mosier, A., Inouye, D. W., Miller, T. E., et al. (2016). Sex-specific responses to climate change in plants alter population sex ratio and performance. Science 353, 69–71. doi: 10.1126/science.aaf2588
Puurtinen, M., and Kaitala, V. (2002). Mate-search efficiency can determine the evolution of separate sexes and the stability of hermaphroditism in animals. Am. Nat. 160, 643–660. doi: 10.1086/342821
R Development Core Team (2008). R: A Language and Environment for Statistical Computing. Vienna: R Foundation for Statistical Computing.
Romo-Piñera, A. K., Ceballos-Vásquez, B. P., García-Domínguez, F., and Arellano-Martínez, M. (2009). Unusual high frequency of hermaphroditism in the gonochoric bivalve Megapitaria squalida (Sowerby, 1835) (Veneridae). J. Shell. Res. 28, 785–789. doi: 10.2983/035.028.0407
Ronquist, F., Teslenko, M., van der Mark, P., Ayres, D. L., Darling, A., Hohna, S., et al. (2012). MrBayes 3.2: efficient Bayesian phylogenetic inference and model choice across a large model space. Syst. Biol. 61, 539–542. doi: 10.1093/sysbio/sys029
Saavedra, C., Reyero, M. I., and Zouros, E. (1997). Male-dependent doubly uniparental inheritance of mitochondrial DNA and female-dependent sex-ratio in the mussel Mytilus galloprovincialis. Genetics 145, 1073–1082.
Sasson, D. A., and Ryan, J. F. (2017). A reconstruction of sexual modes throughout animal evolution. BMC Evol. Biol. 17:242. doi: 10.1186/s12862-017-1071-3
Sastry, A. N. (1979). “Pelecypoda (excluding Ostreidae),” in Reproduction of Marine Invertebrates, eds A. C. Giese and J. S. Pearse (New York: Academic Press), 113–292.
Soria, R. G., Pascual, M. S., and Cartes, V. H. F. (2002). Reproductive cycle of the cholga paleta Atrina seminuda Lamarck 1819 (Bivalvia Pinnidae) from northern Patagonia, Argentina. J. Shell. Res. 21, 479–488.
Stewart, A. D., and Phillips, P. C. (2002). Selection and maintenance of androdioecy in Caenorhabditis elegans. Genetics 160, 975–982.
Terranova, M. S., Brutto, S. L., Arculeo, M., and Mitton, J. B. (2007). A mitochondrial phylogeography of Brachidontes variabilis (Bivalvia:Mytilidae) reveals three cryptic species. J. Zool. Syst. Evol. Res. 45, 289–298.
Theologidis, I., Chelo, I. M., Godoy, C., and Teotónio, H. (2014). Reproductive assurance drives transitions to self-fertilization in experimental Caenorhabditis elegans. BMC Biol. 12:93. doi: 10.1186/s12915-014-0093-1
Toro, J. E., Thompson, R. J., and Innes, D. J. (2002). Reproductive isolation and reproductive output in two sympatric mussel species (Mytilus edulis, M. trossulus) and their hybrids from Newfoundland. Mar. Biol. 141, 897–909.
Torrado, M., and Mikhailov, A. T. (1998). Male-associated polypeptide (MAP) expression in different compartments of the reproductive system of the mussel Mytilus galloprovincialis: immunocytochemical and Western blot study. Cell Tis. Res. 294, 537–547.
Torroglosa, M. E., and Giménez, J. (2019). Reproductive strategy of Brachidontes rodriguezii (Bivalvia: Mytiloida) in the South-western Atlantic Ocean. J. Mar. Biol. Assoc. U. K. 99, 155–162. doi: 10.1017/S0025315417001825
Trovant, B., Orensanz, J. M., Ruzzante, D. E., Stotz, W., and Basso, N. G. (2015). Scorched mussels (Bivalvia: Mytilidae: Brachidontinae) from the temperate coasts of South America: Phylogenetic relationships, trans-Pacific connections and the footprints of Quaternary glaciations. Mol. Phyl. Evol. 82, 60–74. doi: 10.1016/j.ympev.2014.10.002
Trovant, B., Ruzzante, D. E., and Basso, N. B. (2013). Distinctness, phylogenetic relations and biogeography of intertidal mussels (Brachidontes, Mytilidae) from the south-western Atlantic. J. Mar. Biol. Assoc. U. K. 93, 1843–1855.
Vinuesa, J. H. (1977). Sobre un caso de hermafroditismo en la cholga Aulacomya ater (Molina). Physis 37, 63–65.
Weeks, S. C. (2012). The role of androdioecy and gynodioecy in mediating evolutionary transitions between dioecy and hermaphroditism in the animalia. Evolution 66, 3670–3686. doi: 10.1111/j.1558-5646.2012.01714.x
Weeks, S. C., Benvenuto, C., Reed, S. K., Duff, R. J., Duan, Z. H., and David, P. (2014a). A field test of a model for the stability of androdioecy in the freshwater shrimp, Eulimnadia texana. J. Evol. Biol. 27, 2080–2095. doi: 10.1111/jeb.12459
Weeks, S. C., Brantner, J. S., Astrop, T. I., Ott, D. W., and Rabet, N. (2014b). The evolution of hermaphroditism from dioecy in crustaceans: selfing hermaphroditism described in a Fourth Spinicaudatan Genus. Evol. Biol. 41, 251–261.
Weiblen, G. D., Oyama, R. K., and Donoghue, M. J. (2000). Phylogenetic analysis of dioecy in monocotyledons. Am. Nat. 155, 46–58. doi: 10.1086/303303
Wolf, D., and Takebayashi, N. (2004). Pollen limitation and the evolution of androdioecy from dioecy. Am. Nat. 163, 122–137.
Yoshizawa, J., Mimori, K., Yamauchi, K., and Tsuchida, K. (2009). Sex mosaics in a male dimorphic ant Cardiocondyla kagutsuchi. Naturwissenschafte 96, 49–55. doi: 10.1007/s00114-008-0447-z
Yusa, Y. (2009). Causes of variation in sex ratio and modes of sex determination in the Mollusca - An overview. Am. Mal. Bull. 23, 89–98. doi: 10.4003/0740-2783-23.1.89
Keywords: Chile, gonochorism, intertidal mussels, mtDNA, Mollusca, reproduction, sexual ratio, simultaneous hermaphroditism
Citation: Oyarzún PA, Nuñez JJ, Toro JE and Gardner JPA (2020) Trioecy in the Marine Mussel Semimytilus algosus (Mollusca, Bivalvia): Stable Sex Ratios Across 22 Degrees of a Latitudinal Gradient. Front. Mar. Sci. 7:348. doi: 10.3389/fmars.2020.00348
Received: 12 November 2019; Accepted: 27 April 2020;
Published: 25 May 2020.
Edited by:
Leonardo Cruz Da Rosa, Federal University of Sergipe, BrazilReviewed by:
Patrice DAVID, UMR 5175 Centre d’Ecologie Fonctionnelle et Evolutive (CEFE), FranceThiago Mendes, Federal University of São Paulo, Brazil
Copyright © 2020 Oyarzún, Nuñez, Toro and Gardner. This is an open-access article distributed under the terms of the Creative Commons Attribution License (CC BY). The use, distribution or reproduction in other forums is permitted, provided the original author(s) and the copyright owner(s) are credited and that the original publication in this journal is cited, in accordance with accepted academic practice. No use, distribution or reproduction is permitted which does not comply with these terms.
*Correspondence: Pablo A. Oyarzún, pablo.oyarzun@unab.cl