Effects of larval trematode parasitism on the reproductive capacity of Manila clam Ruditapes philippinarum in a sandy-mud tidal flat on the west coast of Korea
- 1Department of Marine Life Science (BK21 FOUR) and Marine Science Institute, Jeju National University, Jeju, South Korea
- 2Marine Science Institute, Jeju National University, Jeju, South Korea
- 3Tidal Flat Research Center, National Institute of Fisheries Science (NIFS), Kunsan, South Korea
Gonad intrusion of the larval trematodes often results in substantial damage to the reproductive capacity of some economically important marine bivalves. Such detrimental impacts of the larval trematode infection are observed in Manila clam Ruditapes philippinarum in the coastal Yellow Sea, although the effects on the host’s reproduction are poorly understood. Accordingly, this study attempts to understand the impacts of parasitism on clam reproduction using histology. Of the 1,255 adult clams collected in early June from a tidal flat on the west coast of Korea, 68 individuals (5.4%) were infected by three different larval trematodes, including Cercaria tapidis, Cercaria pectinata, and Parvatrema duboisi. Histology revealed that the uninfected clams were in the late developing or ripe stage, exhibiting fully developed gametes in the expended follicles. In contrast, clams infected by the larval trematode showed retarded gonad maturation. Some of those clams remained sexually indifferent (13/68 infected clams) or early developing (9/68 infected clams). We categorized the infection level into uninfected (0), light (1), moderate (2), heavy (3), and very heavy (4), according to the area of sporocysts occupying the histology section. The image analysis indicated that approximately 50% of the infected clams were in “heavy” and “very heavy” conditions, and they occupied 16.7% and 26.9% of the total cross-section area, respectively. The nonparametric Kruskal–Wallis’s test and the post-hoc Tukey’s test indicated that the condition index (CI) of clams in heavy and very heavy infection were significantly lower than uninfected clams. In conclusion, the larval trematodes infected about 5.4% of the clam population in the tidal flat on the west coast of Korea, and we estimated that 32.4% of the infected clams might fail to reproduce during a spawning period due to the severe infection.
Introduction
Parasitic infection has been one of the hurdles in the global finfish and shellfish aquaculture industries. According to Shinn et al. (2015), numerous types of parasites impair the global fish aquaculture industry; the world fish aquaculture may lose US$1.05 to 9.58 billion if the parasitism damages 1% to 10% of the market-sized fish. Different types of parasitic organisms such as micro- (i.e., bacteria and protozoa) and macroparasites (e.g., trematodes) also hamper the world shellfish aquaculture industry, though the economic losses caused by the parasites are yet to be known (Bower, 2006; Paladini et al., 2017). Digenean trematodes are among the largest group of metazoan parasites that infect various invertebrates and vertebrates with a complex life history (Rhode, 2005). The digeneans in a sporocyst stage generally utilize mollusks as the first intermediate host and invade a variety of vertebrates and invertebrates in the form of metacercaria (Littlewood, 2006). The metacercaria infection often results in retarded growth (Wegeberg and Jensen, 2003; Thieltges, 2006a); increased energy demand (Magalhães et al., 2019); manipulation of host behavior, leading the host to become conspicuous to their predators (Lafferty and Morris, 1996; Thomas and Poulin, 1998; Eliuk et al., 2020); and mortalities (Gordon and Rau, 1982; Jacobson et al., 2008; Magalhães et al., 2015). However, most metacercaria infections pose a relatively minor impact on hosts, as they do not reproduce in the host (Bower et al., 1994; Rhode, 2005). In contrast, a sporocyst gives rise asexually to tailed cercaria, mainly in the gonad of the mollusks such as bivalves and gastropods (Poulin, 1998; Galaktionov et al., 2006). Such continuous asexual multiplication of daughter sporocysts and cercariae increases the number and size of the larval trematodes, causing host tissue deterioration. This destruction or alteration of host tissue, distinctly in the gonad, refers to parasitic castration (Noble and Noble, 1971; Baudoin, 1975). Several studies have reported trematode infections and their impacts on some commercial marine bivalves, including southern Australian scallop Pecten fumatus by Bucephalus sp. (Heasman et al., 1996), edible cockle Cerastoderma edule by Bucephalus minimus (Magalhães et al., 2015), wedge clam Donax trunculus by Bacciger bacciger (de Montaudouin et al., 2014), and blue mussel Mytilus edulis by Proctoeces maculatus (Markowitz et al., 2016).
Such infection by trematode sporocysts has been observed in Manila clam, Ruditapes philippinarum, inhabiting fine sediments in the shallow to intertidal zones of temperate regions around the world. In the Northwest Pacific, at least three different species of sporocysts (i.e., Cercaria tapidis, C. pectinata, and Parvatrema duboisi) have been morphologically identified (Kim and Chun, 1981; Shimura et al., 1982; Ponurovsky and Yakovlev, 1992; Ngo and Choi, 2004; Park et al., 2008; Yanagida et al., 2009; Jung et al., 2021), while sporocyst infection has not been observed in Manila clam populations in the introduced regions, such as North America (Bower et al., 1992) and Europe, despite rigorous field surveys (Navas et al., 1992; de Montaudouin et al., 2000; Lassalle et al., 2007; Dang et al., 2009). Park et al. (2010) and Hong et al. (2016) reported that the infection prevalence (i.e., percentage of the infected individuals) of sporocysts ranged from 4.2% to 6.7% in Manila clams in sandy-mud tidal flats on the west coast of Korea. In histology, the heavily infected clams exhibited gonad castrations as the ovaries and testis were completely void during the spawning season. Accordingly, the gonad castration by sporocyst infection is expected to cause substantial damage to the clam population. However, the degree and impact of parasitic castration on the Manila clam at a population level are yet to be understood clearly.
The larval trematode infection level in marine bivalves can be evaluated directly by counting the number of sporocysts or metacercariae after extracting them from the host tissue by squeezing or dissecting (Karatayev et al., 2012; Fretas et al., 2014; Galaktionov et al., 2015; Magalhães et al., 2015; Sohn et al., 2017). Alternatively, the presence and intensity of the larvae in the host can be determined using histology (Bower et al., 1992; Powell et al., 1999; Kim et al., 2006; Morgan et al., 2012). For example, in the NOAA’s Status and Trends “Mussel Watch” program, impacts of trematode infection on the health conditions of oysters and mussels were scored from 0 (none) to 4 (the highest level of infection) based on histology (Ellis et al., 1998; Kim and Powell 2007). Similarly, Powell et al. (1999) examined the impacts of trematode parasitism on the health and reproduction of petroleum seep mussels using histology in which the infection level was categorized into numerical scales from 0 (none) to 4 (the highest level). However, those semiquantitative scales developed based on the mussel–trematode system were inapplicable to the Manila clam population, as heavy and light infection could not be distinguished. This could be due possibly to different host–parasite systems, resulting in an erroneous estimation of the infection intensity.
To understand the effects of larval trematode infection on the reproduction of clams, we collected and analyzed a substantial number of clams quantitatively prior to the spawning period using histology and subsequent image analysis. This study first reports the negative impact of the larval trematode infection on the reproductive capacity of the Manila clam population and visually demonstrates a degree of infection.
Materials and methods
Sample collection and examination of the larval trematode infection
Previous studies (Park et al., 2010; Hong et al., 2016) reported a low prevalence of the larval trematodes, ranging from 4.2% to 6.7% in the Manila clam on the west coast. Therefore, a large number of samples were required to secure enough number of infected individuals. In addition, Uddin et al. (2012) reported that most Manila clams on the west coast of Korea are in the late developing or ripe stage in June. Accordingly, we collected 1,255 adult Manila clams with a mean shell length of 36.2 mm from an intertidal mudflat on the west coast of Korea (Figure 1) in June 2018. After measuring the whole tissue weight to the milligram, we screened for larval trematode-infected clams using a needle biopsy. A small portion of clam gonad tissues was excised using a fine syringe needle for the assay. The gonad tissue extracts were placed on a glass slide, and the presence of the larval trematode was examined using a light compound microscope. The needle biopsy revealed that out of 1,255 clams examined, 61 clams were identified to be infected by a larval trematode. Although histology alone would not be able to distinguish trematode species, we were able to identify the larval trematode to species level according to Shimura et al. (1982), as distinctive morphological characteristics of three different species were observed. After the needle biopsy, we randomly selected 428 clams identified as uninfected and 61 infected clams for histology. We also compared the observed multiple infection frequency with the expected frequency, according to Cort et al. (1937), to interpret the co-infection phenomenon.
Histology and condition index
For histology, a thin (2–3 mm) dorsoventral section was removed in the middle of the clam body, as previously described by Uddin et al. (2012). A total of 489 clams, including 61 larval trematode infections confirmed by the needle autopsy, were used in the histological examination. The body section was fixed in Davidson’s fixative solution, and then the tissues went through dehydration and embedding in paraffin. A 6-µm-thick paraffin section was sliced from the paraffin block, deparaffinized, and stained with Harris hematoxylin and eosin Y. Based on microscopic appearance in histology, the reproductive stages of the clams were categorized into six stages, including resting, early developing, late developing, ripe, partially spawning, and spent (Drummond et al., 2006; Uddin et al., 2012). Infection by the protozoan parasite Perkinsus olseni was also examined to determine whether the clam fecundity is further affected by co-infection. The condition index (CI) of each clam was calculated as CI = [dry tissue weight (g)/dry shell weight (g)] × 1,000 (Walne and Mann, 1975; de Montaudouin et al., 2016).
P. olseni infection intensity
The infection intensity of P. olseni was determined using Ray’s fluid thioglycollate medium (RFTM, Ray, 1966) assay. A piece of gill tissue was incubated in RFTM and dissolved in 2M of NaOH to quantify Perkinsus cells according to previously described methods (Choi et al., 1989; Park and Choi, 2001). The intensity was recorded as the number of P. olseni cells/gram of gill tissue.
Image analysis
The entire area of the histology section was first scanned using a digital slide scanner (Axio Scan.Z1, ZEISS) that captured the whole section image at a ×400 magnification from a compound light microscope. To determine the effect of the larval trematodes quantitatively, we then measured total surface area (TSA), larval trematode area (LTA), and gonad area (GA) in the digitized images using an image-contouring tool in Zen 2.3 software (ZEISS) (Figure 2). In addition, TSA and GA of 100 randomly selected clams that were not infected by the larval trematodes were also measured to compare the proportion of gonad area to the total surface area between the uninfected and castrated clams.
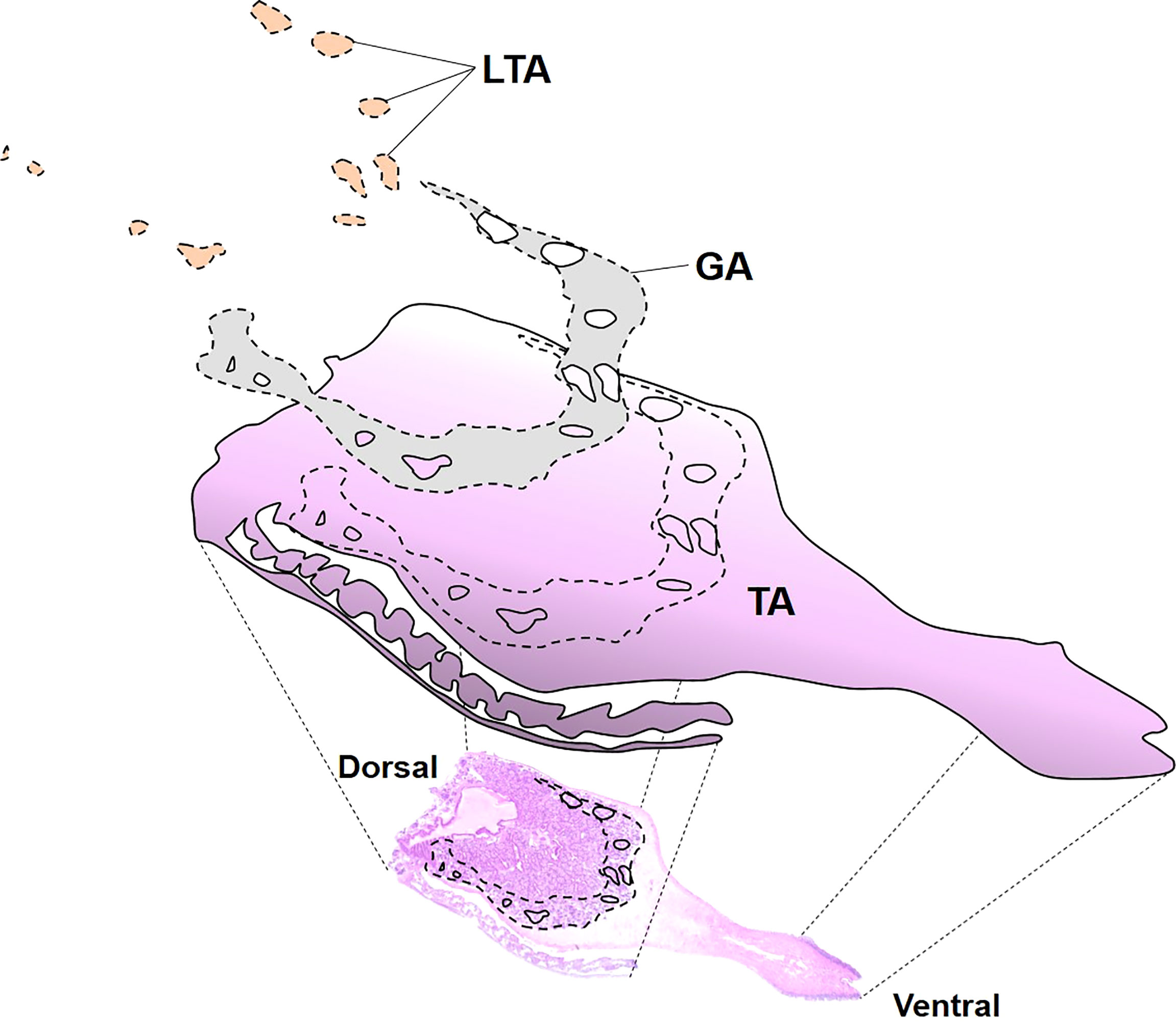
Figure 2 Digitized image of each histological slide. TA, the total area of the scanned slide, GA, the gonad area occupied by eggs or sperms, LTA, and the larval trematode area.
Semiquantitative scale
As different infection phases were observed in the clam from histology, we categorized larval trematode infections into five types, including none (0), light (1), moderate (2), heavy (3), and very heavy (4), based on the developmental stage of trematodes and their distribution in the host. The differences among the five infection categories were validated by comparing the mean proportion of parasite surface area to host using boxplots. In addition, the infection level of the larval trematodes in 68 infected clams was assessed using this semiquantitative scale.
Statistical analysis
The data were tested for normal distribution (Shapiro–Wilk test) and homogeneity of variances (Levene’s test). As the proportion of GA to TSA in the castrated clams was not normally distributed, a nonparametric Mann–Whitney U-test was used to compare those with the uninfected clams. The ratio of LTA to TSA of the four infection categories was compared using nonparametric Kruskal–Wallis’s one-way analysis of variance (ANOVA, Sokal and Rohlf, 1995) and Tukey’s post-hoc range test. We also tested the effects of infection on clam condition using a nonparametric Kruskal–Wallis’s one-way ANOVA and Tukey’s range test. In addition, the observed co-infection frequency and the expected frequency were compared using the Chi-squared test. The statistical analysis was performed using SPSS v.21.0 software (IBM Corporation, New York, USA), and p < 0.05 was considered statistically significant.
Results
Identification of larval trematode species and the prevalence
The needle biopsy analysis indicated that 61 out of 1,255 clams (4.9%) examined contained larval trematodes. However, histology identified seven more infected clams, suggesting that the needle autopsy is somewhat less sensitive than histology. Consequently, the larval trematode infection prevalence in adult clams was estimated to be 5.4% (68/1,255). It was noticeable that the seven clams contained only germinal balls in the sporocysts, which may have mislead those clams as uninfected in the needle autopsy.
Three different species of larval trematode could be extracted and identified from the sporocysts isolated from the visceral mass/gonad by the needle. Under a light microscope, C. tapidis exhibited distinct eyespots with a comparatively long tail (Figure 3A), while C. pectinata included numerous setae on the tail (Figure 3B). The cercaria stage of P. duboisi was characterized by a bifurcated tail (Figure 3C).
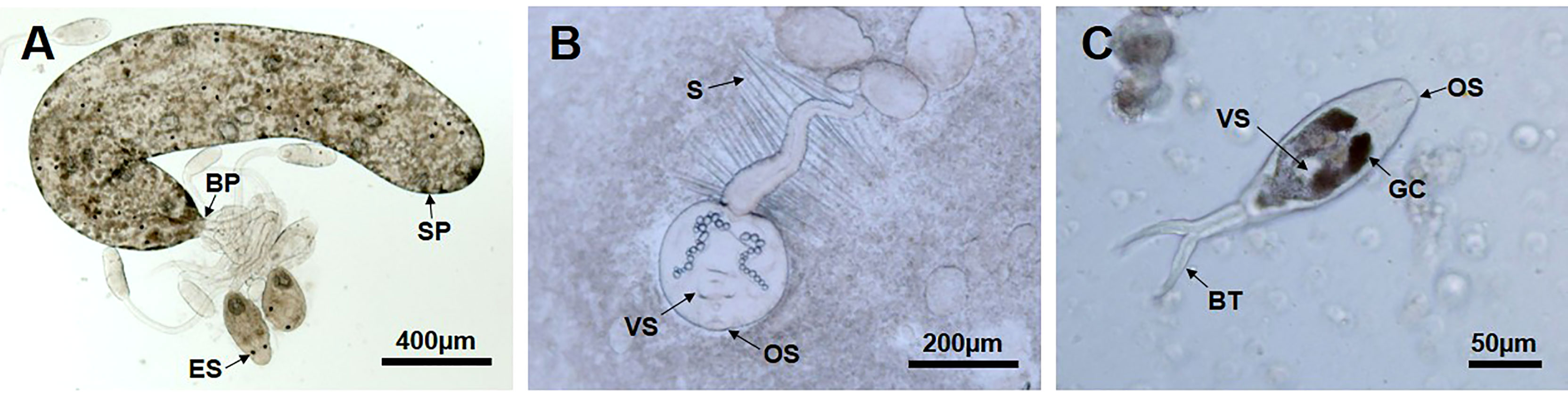
Figure 3 Three different types of larval trematodes were identified from Manila clam. (A) Sporocyst (SP) discharging the immature and mature Cercaria tapidis through the birth pore (BP). ES, eye spot. (B) Cercaria pectinata distinguished by a tail with setae (S). OS, oral sucker, VS, ventral sucker. (C) Parvatrema duboisi in cercaria stage with a bifurcated tail (BT). GC, gut caeca.
The three larval trematode species were distinguishable in histology based on the morphological characteristics (Figures 4B, C). Out of the 68 infected clams, infection by C. tapidis was most prevalent (40/68), followed by C. pectinata (18/68) and P. duboisi (4/68 clams). Histology also indicated that C. tapidis and C. pectinata co-infected a single clam (1/68). The observed co-infection frequency (1/1,255 = 0.0008) was significantly lower than the expected frequency (0.5737) (Chi-squared test, p < 0.05), suggesting possible interspecific competition. While most infected clams exhibited mature larval cercaria in the sporocysts, some clams (7/68) included only germinal cells or balls in the host tissues. Histology also revealed that the larval trematodes infect both female (28/68) and male clams (30/68). The female-to-male sex ratio was 0.93:1.00 in the cercaria-infected clam group and 0.85:1.00 in the cercaria-uninfected group (Supplementary Table S2).
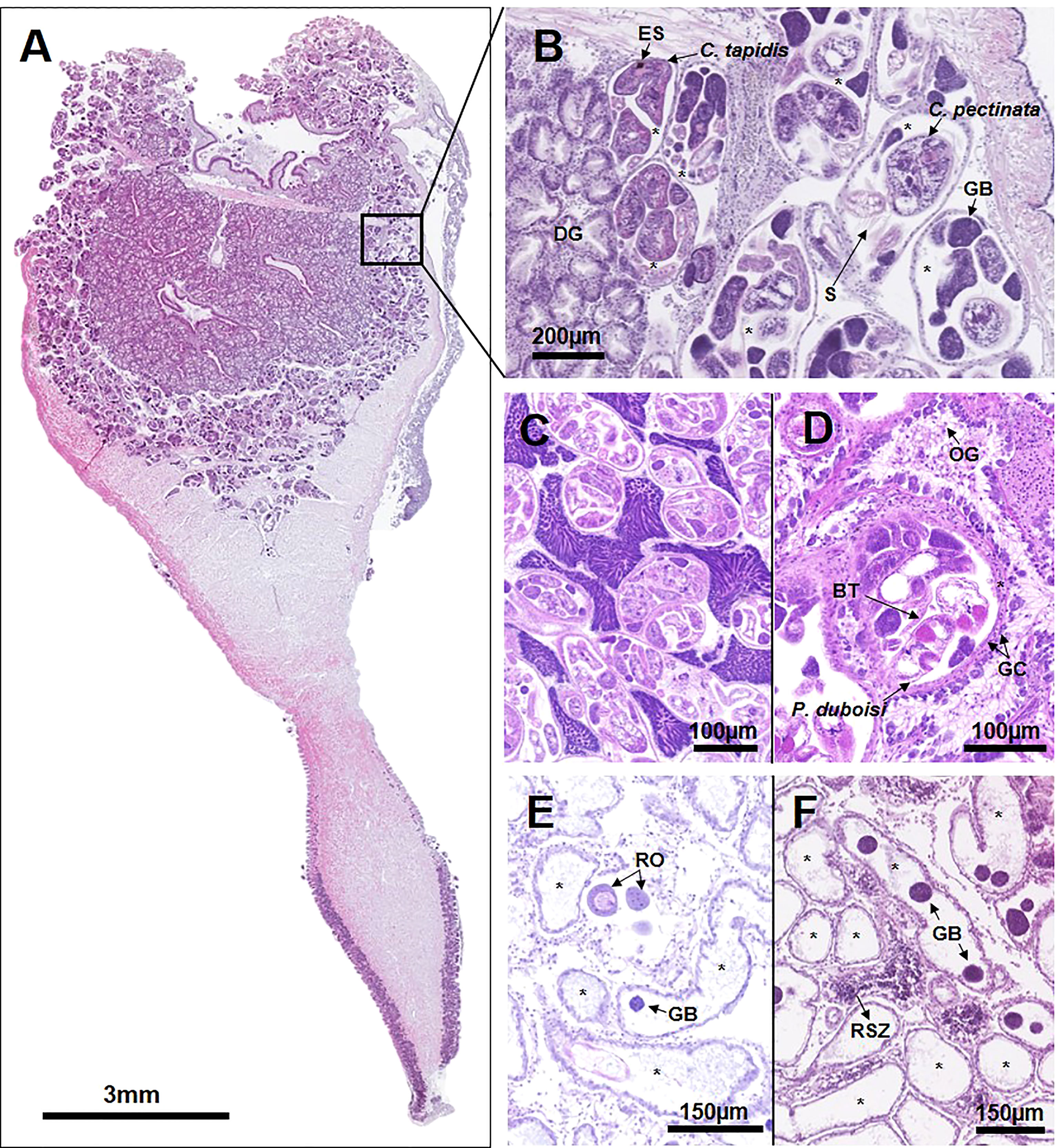
Figure 4 Microphotographs demonstrating the occupation of the Manila clam reproductive system by the larval trematodes. (A) Entire longitudinal clam section, in which the larval trematodes fully occupy the clam gonad. (B) Magnified view of the affected area near digestive gland (DG), exhibiting co-infection by Cercaria tapidis and Cercaria pectinata. Sporocysts (*) containing cercariae (C) and germinal balls (GB) are fully occupying the gonad. C. tapidis is distinguished by eye spots (ES), while C. pectinata is characterized by a tail with setae (S). (C) Partially castrated male clam with normal gametogenesis. (D) Partial castration with inhibited gametogenesis in the female clam. Oogonia (OG) are attached to the basal membrane of the follicular wall, indicating that the clam is in the early developing stage. Castration is caused by the intrusion of Parvatrema duboisi cercaria possessing the bifurcated tail (BT) and gut caeca (GC). (E, F) Postcastration demonstrating full occupation of the clam gonad by sporocysts (*) containing germ balls (GB) or empty sporocysts. Some follicles contain relic oocyte (RO) or relic spermatozoa (RSZ).
Distribution and pathology
In histology, the larval trematodes were found in the gonad, digestive gland, gills, and mantle (Figure 4A). As shown in Figure 4A, the gonad was the most common infection organ, while the larval trematodes were not observed in the foot muscular tissue. The developmental stages of the larval trematodes observed in this study included the early-stage sporocyst containing germinal cells or germinal balls and the growing or mature sporocysts containing germinal balls and mature cercariae (Figure 4B). The oval- to egg-shaped sporocysts containing developing cercaria and germinal balls were the most prevalent form observed in the clam (Figure 4B). The sporocysts were located in the interfollicular area and were separated clearly from the host tissue and hemocytes by the outer tegumental layer of the parasite (Figure 4C).
We identified four different types of larval trematode infection consequences: (1) complete castration with an absence of gonad follicles (Figure 3B), (2) partial castration with normal gonad development (Figure 4C), (3) partial castration with retarded gonad development (Figure 4D), and (4) postcastration with a few mature residual gametes (Figure 4E). The complete castration was characterized by the full occupation of the gonad by sporocysts containing mature cercariae and germ balls. The first type of partial castration was characterized by the larvae and an unaffected normal gonad, the same reproductive stage as an uninfected clam (Figure 4C). In contrast, some clams exhibited previtellogenic oocytes attached to the basal membrane of the follicular wall (Figure 4D) or spermatogonia on the periphery of the acinus (Figure 5), indicating that the gonad maturation was retarded, possibly due to the infection. Clams in the postcastration phase showed empty sporocysts or sporocysts containing a few germ balls in the follicles (Figures 4E, F). Residual mature oocytes or spermatozoa were found in clams in the postcastration phase.
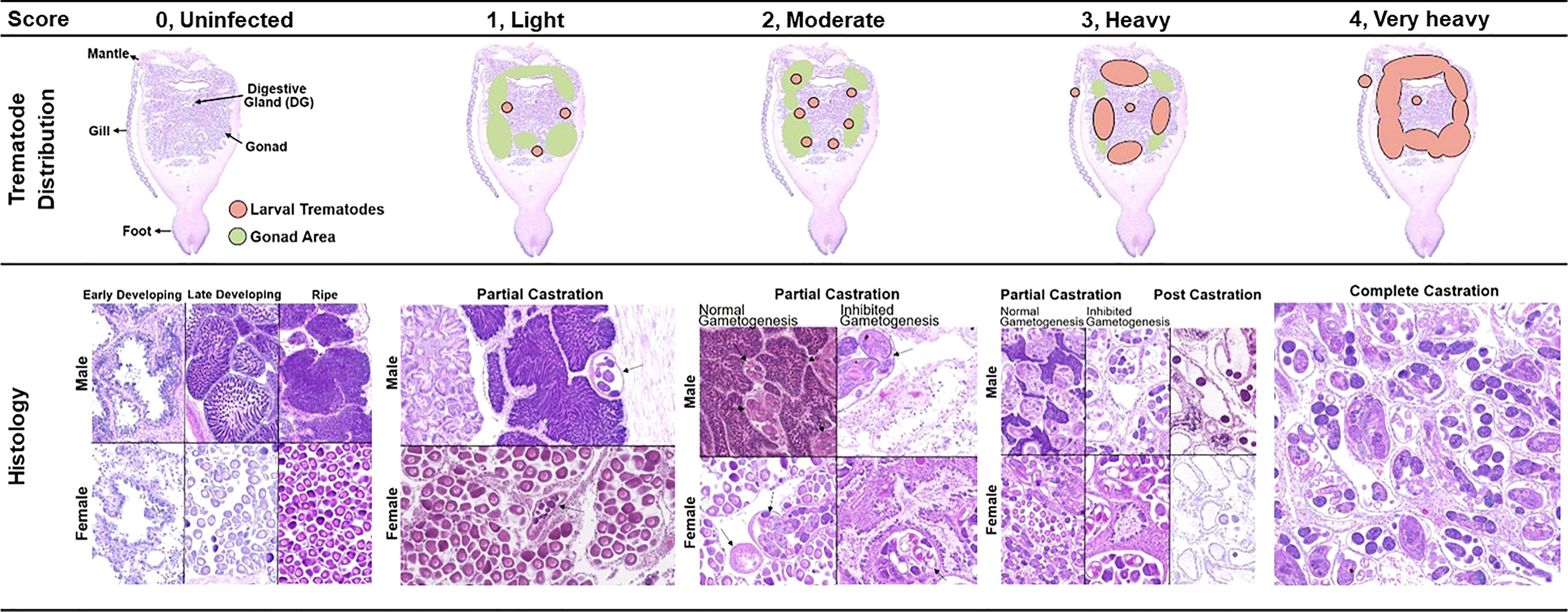
Figure 5 Frequency distribution of different reproductive stages of Manila clams in different infection statuses. Uninfected (0) clams exhibit well-developed gonads and no larval trematode in any tissue. Light (1), early-stage sporocysts contain germinal cells or germinal balls, and the sporocytes are found in the gonad. Moderate (2), the sporocysts contain germinal balls and a few mature cercaria.
Manila clam reproductive stages
As Manila clams in this study were collected in early June, during which the common reproductive stage of the clam includes the late-developing and ripe stage (Park and Choi, 2004; Uddin et al., 2012), we considered clams in the resting or early developing stage as abnormal. Most clams in this study were in the late developing or ripe stage (469/489, 94.5%), whereas 5.5% (27/489) of the clams were in the early developing (14/489) or the resting stage (13/489). Histology revealed that such reproductively retarded clams were severely infected by the larval trematodes. Nine out of 14 clams in the early developing stages were heavily infected by the larval trematodes (Figure 6).
Figure 6 represents the numbers and frequency distribution of the reproductive stages of the Manila clams in different infection categories. The reproductive condition of clams in “free of infection” and “exclusively infected by P. olseni” demonstrated a similar pattern, as more than 96% of clams in these categories were in the late-developing or ripe stage. In contrast, the normal reproductive stages only account for 61.5% and 69.1% of the clams “exclusively infected by Cercaria spp.” and “co-infected by Cercaria spp. and P. olseni,” respectively, indicating that the occurrence of abnormal reproductive stages (i.e., indifferent and early developing) is closely related to the larval trematode infection. The RFTM assay revealed that the mean P. olseni infection intensity of clams “exclusively infected by P. olseni” was 1,426,207 ± 1,608,685 cells/g gill tissue and 1,094,303 ± 1,559,695 cells/g gill tissue in the clams co-infected by P. olseni and Cercaria spp. (Supplementary Table S1).
GA in castrated and uninfected clams
The image analysis indicated that in June, GA occupied 28.0% of the total section area when clams were fully ripe and free from larval trematode infection (Table 1). In contrast, clams infected by the larvae and exhibiting a certain type of castration showed significantly smaller GA (14.4%, p < 0.05).
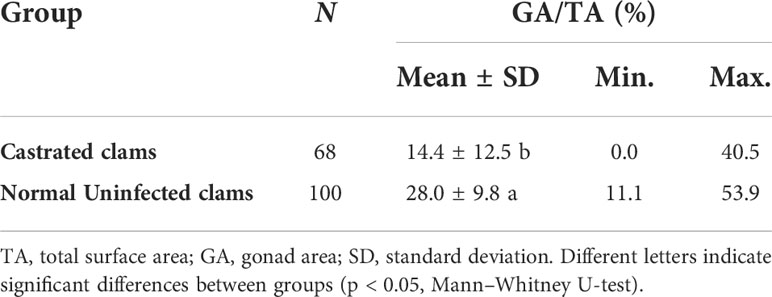
Table 1 The proportion of surface area occupied by larval trematodes in the total cross-section and gonad area of the Manila clam.
Categorization of the degree of infection
Figure 5 represents each category of the semiquantitative scale. The scale has a range of 0 (none) to 4 (very heavily infected). The early-stage sporocysts containing germinal cells are solely observed in the light infection (score 1), while the mature sporocysts containing germinal balls and cercariae are found in moderate, heavy, and very heavy infections. In moderate infection (score 2), the sporocysts containing mature cercariae and a few germ balls occupy the gonad and digestive gland of the clam. The heavy infection (score 3) can be differentiated from the moderate by the area occupied by the larval trematodes. In very heavy infection (score 4), sporocysts containing mature cercariae and a few germ balls occupy the gonad, digestive gland, mantle, and gill. Fully occupied follicles by the larval trematode are the characteristics of the very heavy infection.
Boxplots in Figure 7 demonstrate the distribution pattern of LTA/TSA (%) among the four infection categories. The percent area ranged from 0.3 ± 0.0 in light, 9.2 ± 5.7 is moderate, 16.7 ± 5.5 in heavy, and 26.9 ± 7.2% in very heavy infection, respectively. Nonparametric Kruskal–Wallis’s test and Tukey’s post-hoc range test revealed that the mean of LTA/TSA was significantly different among the groups (p < 0.05).
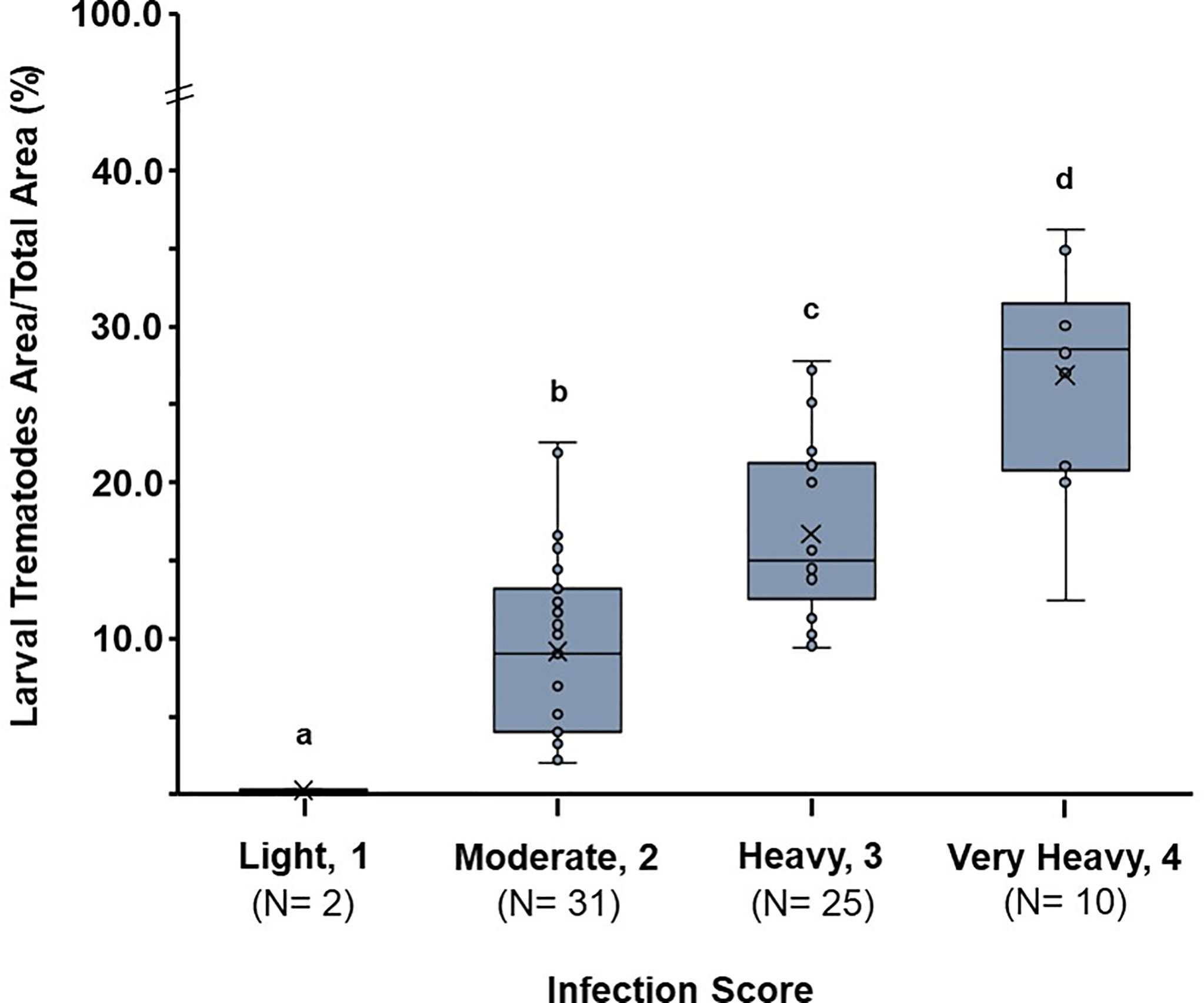
Figure 7 Boxplots demonstrating proportion of parasite surface area by infection score in this study. Median and mean values are indicated with a line within the box and “x,” respectively. LTA, larval trematode area; TA, total area; GA, gonad area. Different letters indicate significant differences between groups (p < 0.05, Kruskal–Wallis test).
Application of the semiquantitative score
When the semiquantitative infection score was applied to the 68 trematode-infected clams, 10 clams were in very heavy, 25 clams in heavy, 31 clams in moderate, and two clams in light infection (Table 2). Each infection category also corresponded to one or more castration types. While the very heavy infection (four) and light infection (one) solely corresponded to “complete castration” and “partial castration,” respectively, the moderate (two) and heavy infection (three) were a mixture of two or more castration types.
Histology also indicated that 46 individuals from 68 infected clams (67.6%) were in normal reproductive condition (i.e., late-developing or ripe), although the gonad is partially castrated. In contrast, the rest of the 22 clams (32.4%) failed to reproduce, as 10 clams were in “complete castration,” three clams in “postcastration,” and nine clams in “partial castration with inhibited gametogenesis.”
Correlation between the infection and CI of clam
Table 3 summarizes the CIs of Manila clams in different cercaria-infection categories. The nonparametric Kruskal–Wallis test and the post-hoc Tukey’s range test indicated that the mean CI of cercaria-free clams (192 ± 29, N = 421) is significantly higher than the CI of clams in heavy (171 ± 26, N = 25) or very heavy (167 ± 44, N = 10) infection (p < 0.05). However, the test also indicated that the mean CI of uninfected clams did not differ significantly from the light and moderate infections.
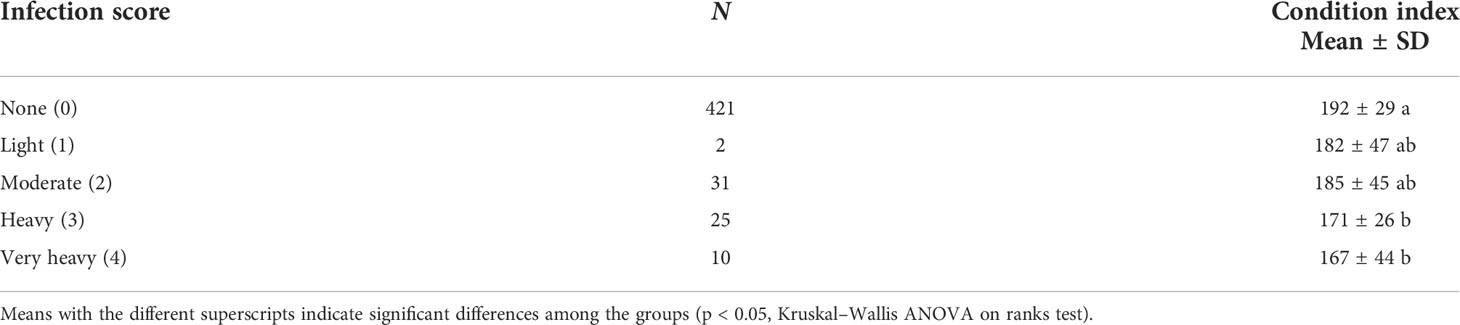
Table 3 Mean condition index of the Manila clams in different parasite infection profiles and infection scores.
Discussion
Larval trematodes infection in Manila clam
Infection prevalence of Cercaria spp. in the adult Manila clam (mean SL: 36.2 mm) in this study (5.4%) is comparable to that observed in the clams (SL range: 28.2–39.5 mm) on the west coast (4.2% to 6.7%) (Park et al., 2010; Hong et al., 2016), while the prevalence reported in other regions are somewhat variable. In a pathology survey of the adult Manila clam (mean SL range: 28.25–34.34 mm) carried out in three different clam beds on the coast of Ariake Bay, Japan (Park et al., 2008), the mean infection prevalence of Cercaria spp. was 12.2%, with the value ranging from 7.1% to 25.0%. Meanwhile, a substantially high infection prevalence of the larval trematode was reported in three clam beds (R. decussatus, mean SL range: 30.2–45.1 mm) in Egypt with an annual mean prevalence ranging from 17.9% to 24.2%, and the highest monthly prevalence reached 81.7% in one of the clam fisheries (El-Wazzan and Radwan, 2013). The variable prevalence observed throughout the regions could be addressed by two explanations (i.e., environmental heterogeneity and different trematode species). According to Byers et al. (2016), the infection prevalence of trematodes is closely related to habitat characteristics and therefore shows site-specific consistency. The prevalence of the larval trematodes depends on the species as well. As the intermediate host and the definitive host may vary depending specifically on the host-parasite system, the spatial heterogeneity among the regions is believed to be one explanation.
In this study, we first presented the infection prevalence of three different sporocyst species separately, whereas previous studies reporting sporocyst infection in Manila clams on the west coast of Korea provided the merged infection prevalence without distinguishing the species. It is somewhat surprising that two different larval trematode species (i.e., C. tapidis and C. pectinata) co-infect the clam individual, although the incidence rate was low (one out of 68 infected clams). The very low prevalence of co-infection and the fact that the observed co-infection frequency is lower than the expected value suggest that competition might occur among the larval trematodes in the clam population. This competition seems plausible as the three different larval trematodes share a similar way of food consumption in the same ecological community. Indeed, such double infections by larval trematodes generally occur at a low incidence rate (Kuris and Lafferty, 1994; Miura and Chiba, 2007; Magalhães et al., 2020), suggesting possible interspecific competition within the parasite population. Similarly, several trematode families in varying life stages seem to co-exist in the clam individual and population. Although not recorded in this study, the Manila clam not only harbors sporocysts but also metacercarial P. duboisi in the mantle (Le et al., 2015; Cho et al., 2020) and Echinostomatidae metacercariae in the foot (Kim et al., 2004). Furthermore, the prevalence of co-infection among these trematodes is much higher than the prevalence of co-infection among the competing larval trematodes in the sporocyst stage (unpublished data). This could be further explained as a result of niche partitioning among the larval trematode species. In other words, three larval trematode species in the sporocyst stage (i.e., C. tapidis, C. pectinata, P. duboisi) compete for limited resources in the clam visceral mass, while the other two trematode metacercariae co-exist with the trematode sporocysts by using different resources and forming different niches.
Effects of the larval trematode infection on the host reproduction
We found a substantial increase in gonad tissue atrophies when the clams are associated with the infection. Among the 68 infected clams, 35 individuals were in very heavy (four) or heavy (three) infection, indicating that more than half of the infected clams are severely impacted, demonstrating markedly reduced follicle area. Histology indicated that the severe infection eventually leads to 32.4% of the infected clams failing to reproduce during a spawning period. Several studies have also observed such atrophies mediated by gonadal castration (Shelley et al., 1988; Lasiak, 1992; Da Silva et al., 2002; Meng et al., 2019).
According to Sullivan et al. (1985), castration by the parasites could be the consequence of either mechanical (i.e., ingestion or pressure) or chemical (i.e., secretion of inhibition factors) activities of the parasites. The mechanical destruction of host tissue is closely related to the feeding activity of the parasite. Dubois et al. (2009) reported that Labratrema minimus sporocysts feed on the dissolved organic matter metabolized by the host cockle, Cerastoderma edule. Alternatively, Coustau et al. (1991) suggested that parasitic castration of the mussel Mytilus edulis is induced by antimitotic activity of the larval trematodes. Coustau et al. (1991) found a mitosis-inhibiting factor in the parasitized mussels, although it was not clearly understood whether the parasites directly affect the host gametes or stimulate the gametogenesis inhibitory system of the host. As we observed both reduced reproductive effort (i.e., the gonad area) and inhibited gametogenesis, it is believed that the larval trematode in Manila clams exerts both mechanical and chemical impacts, resulting in gonadal castration.
In this study, inhibition or retarded gametogenesis was also found in the clams not infected by the larval trematodes, suggesting that factors other than trematode infection, such as limited food supply and environmental contamination, could also be negatively associated with gonad development (Kluytmans et al., 1988; Siah et al., 2003; Dukeman et al., 2005; Uddin et al., 2013). Furthermore, along with the larval trematode, the protozoan parasite P. olseni may also deteriorate the gonad maturation of Manila clam, as reported by Park et al. (2006) and Uddin et al. (2010). In June 2018, clams in both “free of infection (i.e., not infected by P. olseni or larval trematode)” and “exclusively infected by P. olseni” reached late-developing (80%) or ripe (17 to 20%), although the P. olseni infection intensity was considerably high. This suggests a benign effect of Perkinsus infection on clam reproduction. However, as histology revealed, clams in reproductive rest in June, when most clams were ready to spawn, were 100% infected by the larval trematodes with the “heavy and very heavy” infections, suggesting that the larval trematodes mainly cause the observed abnormal gonad development. According to Park and Choi (2004), female Manila clams in the tidal flat in Gomso Bay, approximately 90 km to the south of this study site, produce 0.94 to 11.79 million eggs during a spawning period. Similarly, Uddin et al. (2012) estimated the fecundity of Manila clams in a tidal flat in Incheon Bay, about 80 km to the north of the study site, as 2.54 to 15.8 million eggs just prior to spawning. Therefore, we believe that the female Manila clams in Jugyo tidal flat may produce a similar quantity of eggs during the spawning as Park and Choi (2004) and Uddin et al. (2012) reported. Accordingly, it is estimated that the total castration by the larval trematode infection would result in a deficit of several million eggs during spawning, which may, in turn, affect the population by damaging the subsequent recruitment.
Semiquantitative larval trematode infection scale
Histology-based semiquantitative scores in previous studies include a 5-point scale by Kim et al. (2006), a 3-point scale by El-Wazzan and Radwan (2013), and a 4-point scale by Da Silva et al. (2002). Based on the qualitative distribution of the parasite within the host, Kim et al. (2006) categorized infection intensity as follows: “0 (uninfected)”, “1 (present in the gonads only)”, “2 (completely filling the gonads),” “3 (completely filling the gonads; extensive invasion of the digestive gland and/or the gills)”, and “4 (completely filling the gonads; substantially filling the digestive gland or gill; individuals appear to be a sac of sporocysts).” The other two studies deployed stereology to estimate the area occupied by the larval trematodes from the histology section. Da Silva et al. (2002) measured the parasite volume fraction from the gonad of brown mussel, Perna perna, and described four intensity categories (light, parasite volume fraction less than 5% of the gonad; moderate, 5%–25%; heavy, 25%–50%; very heavy, more than 50%). Similarly, El-Wazzan and Radwan (2013) measured the area of the parasite in the gonad of the carpet shell clam, Ruditapes decussatus, and classified them into “light (less than 25%),” “medium (26%–75%),” and “heavy (more than 75%).” Despite the introduction of these semiquantitative scores, no score was applicable to the Manila clam histology due probably to differences in infection patterns among host-parasite systems. According to Kim et al. (2006), the previous 5-point scale was developed based on mytilid mussels infected by sporocysts of the families Fellodistomidae and Bucephalidae. Although the stereology-based infection scales by El-Wazzan and Radwan (2013) and Da Silva et al. (2002) could be an alternative, these methods are applicable only when histology sections are stereologically analyzed. Accordingly, we re-categorized the degree of infection into five categories, among which the mean proportion of PA/TA was significantly different. The distribution within the host and the developmental stage of the trematode larvae were the most important considerations in classifying each category since those two factors were closely related to the infection intensity. According to several studies that addressed intramolluscan development of digenetic trematodes, numerous sporocysts containing cercariae are developed from a single miracidium that penetrates a host (Wilson et al., 1971; Ataev et al., 1998; Skála et al., 2014). Thus, the suggested semiquantitative score could be simply applicable in routine analysis of the Manila clam histology, as demonstrated in this study. Furthermore, we emphasize the importance of discriminating between light and heavy trematode infection, as an increased mass of the larval trematodes leads to decreased fecundity and CI of the clam.
Larval trematode infection and the clam condition index
According to previous studies, larval trematodes could weigh up to 34% of the total flesh mass of the cockle, Cerastoderma edule (Baudrimont and de Montaudouin, 2007), and 19% of the wedge clam, Donax trunculus (de Montaudouin et al., 2014). Taking this into account, the negative impact of larval trematode infection on a bivalve CI could be masked by the weight gain caused by parasites. Nevertheless, in this study, the CI of the Manila clam was significantly lower in the individuals with heavy (three) and very heavy (four) infections when compared to the noninfection group. This manifest difference was observable, as a gap between the CI of the normal clam and the heavily infected clam could be maximized when the normal clams are in the ripe stage. In previous studies conducted on the west coast of Korea, the CI of the Manila clam reaches its annual peak in April and June as the clams are ready to spawn (Park and Choi, 2004; Uddin et al., 2010; Uddin et al., 2012). If the CI of the normal clam in the resting stage is compared with that of the heavily infected clams, there would be no clear difference between the two groups. Indeed, de Montaudouin et al. (2014) also demonstrated that the mean CI of the larval trematode-infected wedge clams was lower than the uninfected clams during the gametogenesis period, while there was no significant difference during the postspawning season. In any case, our results suggest that a heavy larval trematode infection in the clam causes substantial damage to the reproductive capacity.
Conclusion
The evidence from this study demonstrated that infection by the three Cercaria spp. causes substantial damage to the reproductive capacity of the Manila clam. Reproductive abnormalities, including reduced gonad area and inhibition in gametogenesis, were closely associated with the larval trematodes infection. Such infection was observed in 5.4% of the clam population on the west coast, and more than half of those infected clams were severely affected, indicating that trematode infection might pose a substantial impact on the clam population. Based on the histology observation, we suggest a semiquantitative score that could be simply applied to the Manila clam histology.
Data availability statement
The raw data supporting the conclusions of this article will be made available by the authors, without undue reservation.
Author contributions
Y-GC and K-SC conceived, designed, and supervised the study. Y-GC, H-ML, J-HK, and J-SS performed the experiments. Y-GC, H-ML, and H-DJ analyzed the data. Y-GC and K-SC wrote the manuscript. All authors reviewed this manuscript. These authors contributed to the article and approved the submitted version.
Funding
This study was supported by the Basic Science Research Program through the National Research Foundation of Korea (NRF) funded by the Ministry of Education (grant number 2019R1A6A1A03033553). This study was also partly supported by the project “Shift of Marine Ecosystem in Response to Climate Change in the Tsushima Warm Current System of Korean Waters,” funded by the Korean Ministry of Oceans and Fisheries (grant number 20220559).
Conflict of interest
The authors declare that the research was conducted in the absence of any commercial or financial relationships that could be construed as a potential conflict of interest.
Publisher’s note
All claims expressed in this article are solely those of the authors and do not necessarily represent those of their affiliated organizations, or those of the publisher, the editors and the reviewers. Any product that may be evaluated in this article, or claim that may be made by its manufacturer, is not guaranteed or endorsed by the publisher.
Acknowledgments
The authors wish to thank the staff of the Shellfish Research and Aquaculture Laboratory at Jeju National University for their valuable help with all experimental work.
Supplementary material
The Supplementary Material for this article can be found online at: https://www.frontiersin.org/articles/10.3389/fmars.2022.936520/full#supplementary-material
References
Ataev G. L., Fournier A., Coustau C. (1998). Comparison of Echinostoma caproni mother sporocyst development in vivo and in vitro using Biomphalaria glabrata snails and a b. glabrata embryonic cell line. J. Parasitol. 84 (2), 227–235. doi: 10.2307/3284475
Baudoin M. (1975). Host castration as a parasitic strategy. Evolution 29 (2), 335. doi: 10.2307/2407221
Baudrimont M., de Montaudouin X. (2007). Evidence of an altered protective effect of metallothioneins after cadmium exposure in the digenean parasite-infected cockle (Cerastoderma edule). Parasitology 134 (2), 237. doi: 10.1017/S0031182006001375
Bower S. M. (2006). “Parasitic diseases of shellfish,” in Fish diseases and disorders (Vol. 1). Eds. Woo P. T., Bruno D. W. (Wallingford: CABI publishing), 629–677.
Bower S. M., Blackbourn J., Meyer R. (1992). Parasite and symbiont fauna of Japanese littlenecks, Tapes philippinarum (Adams and reev), in British Columbia. J. Shellfish Res. 11, 13–19.
Bower S. M., McGladdery S. E., Price I. M. (1994). Synopsis of infectious diseases and parasites of commercially exploited shellfish. Annu. Rev. Fish Dis. 4, 1–199. doi: 10.1016/0959-8030(94)90028-0
Byers J. E., Holmes Z. C., Blakeslee A. M. H. (2016). Consistency of trematode infection prevalence in host populations across large spatial and temporal scales. Ecology 97 (7), 1643–1649. doi: 10.1002/ecy.1440
Choi K.-S., Wilson E. A., Lewis D. H., Powell E. N., Ray S. M. (1989). The energetic cost of Perkinsus marinus parasitism in oysters: quantification of the thioglycollate method. J. Shellfish Res. 8, 125–131.
Cho Y.-G., Kang H.-S., Le C. T., Kwon M. G., Jang M.-S., Choi K.-S. (2020). Molecular characterization of urosporidium tapetis sp. nov., a haplosporidian hyperparasite infecting metacercariae of Parvatrema duboisi (Dollfus 1923), a trematode parasite of Manila clam Ruditapes philippinarum on the west coast of Korea. J. Invertebr. Pathol. 175, 107454. doi: 10.1016/j.jip.2020.107454
Cort W. W., McMullen D. B., Brackett S. (1937). Ecological studies on the cercariae in Stagnicola emarginata angulata (Sowerby) in the Douglas lake region, Michigan. J. Parasitol. 23 (5), 504–532. doi: 10.2307/3272399
Coustau C., Renaud F., Delay B., Robbins I., Mathieu M. (1991). Mechanisms involved in parasitic castration: in vitro effects of the trematode Prosorhynchus squamatus on the gametogenesis and the nutrient storage metabolism of the marine bivalve mollusc Mytilus edulis. Exp. Parasitol. 73 (1), 36–43. doi: 10.1016/0014-4894(91)90005-H
Dang C., de Montaudouin X., Bald J., Jude F., Raymond N., Lanceleur L., et al (2009). Testing the enemy release hypothesis: trematode parasites in the non-indigenous Manila clam Ruditapes philippinarum. Hydrobiologia 630 (1), 139–148. doi: 10.1007/s10750-009-9786
Da Silva P. M., Magalhães A. R. M., Barracco M. A. (2002). Effects of bucephalus sp. (Trematoda: Bucephalidae) on Perna perna mussels from a culture station in ratones grande island, Brazil. J. Invertebr. Pathol. 79 (3), 154–162. doi: 10.1016/S0022-2011(02)00026-5
de Montaudouin X., Kisielewski I., Bachelet G., Desclaux C (2000). A census of macroparasites in an intertidal bivalve community, Arcachon Bay, France. Oceanol. Acta 23 (4), 453–468. doi: 10.1016/S0399-1784(00)00138-9
de Montaudouin X., Bazairi H., Mlik K. A., Gonzalez P. (2014). Bacciger bacciger (Trematoda: Fellodistomidae) infection effects on wedge clam Donax trunculus condition. Dis. Aquat. Organ. 111 (3), 259–267. doi: 10.3354/dao02769
de Montaudouin X., Lucia M., Binias C., Lassudrie M., Baudrimont M., Legeay A., et al. (2016). Why is asari (= Manila) clam Ruditapes philippinarum fitness poor in arcachon bay: a meta-analysis to answer? Estuar. Coast. Shelf Sci. 179, 226–235. doi: 10.1016/j.ecss.2015.09.009
Drummond L., Mulcahy M., Culloty S. (2006). The reproductive biology of Manila clam, Ruditapes philippinarum, from the north-West of Ireland. Aquaculture 254, 326–340. doi: 10.1016/j.aquaculture.2005.10.052
Dubois S. Y., Savoye N., Sauriau P. G., Billy I., Martinez P., de Montaudouin X. (2009). Digenean trematodes–marine mollusc relationships: a stable isotope study. Dis. Aquat. Org. 84 (1), 65–77. doi: 10.3354/dao02022
Dukeman A. K., Blake N. J., Arnold W. S. (2005). The reproductive cycle of the flame scallop, Ctenoides scaber (Born 1778), from the lower Florida keys and its relationship with environmental conditions. J. Shellfish Res. 24 (2), 341–351. doi: 10.2983/0730-8000(2005)24[341:TRCOTF]2.0.CO;2
Eliuk L. K., Brown S., Wyeth R. C., Detwiler J. T. (2020). Parasite-modified behaviour in non-trophic transmission: trematode parasitism increases the attraction between snail intermediate hosts. Can. J. Zool. 98 (7), 417–424. doi: 10.1139/cjz-2019-0251
Ellis M. S., Barber R. D., Hillman R. E., Kim Y., Powell E. N. (1998). “Histopathology analysis,” in Sampling and analytical methods of the national status and trends program mussel watch projects: 1993, 1996 updated,. Eds. Lauenstein G. G., Cantillo A. (Silver Spring, Maryland: NOAA Tech. Mem. NOS. ORCA), 130, 198–215.
El-Wazzan E., Radwan E. H. (2013). Histopathological study for evaluation of trematode larval infection in the carpet shell clam, Tapes decussatus, from three Egyptian clam fisheries. Egypt. J. Aquat. Res. 39 (4), 291–301. doi: 10.1016/j.ejar.2013.12.010
Fretas R., Martins R., Campino B., Figueira E., Soares A. M. V. M., Montaudoin X. (2014). Trematode communities in cockles (Crastoderma edule) of the ria de aveiro (Portugal): influence of inorganic contamination. Mar. pollut. Bull. 82, 117–126. doi: 10.1016/j.marpolbul.2014.03.012
Galaktionov K. V., Bustnes J. O., Bardsen B.-J., Wilson J. G., Nikolaev K. E., Sukhotin A. A., et al. (2015). Factors influencing the distribution of trematode larvae in blue mussels Mytilus edulis in the north Atlantic and Arctic oceans. Mar. Biol. 162, 193–206. doi: 10.1007/s00227-014-2586-4
Galaktionov K. V., Irwin S. W. B., Saville D. H. (2006). One of the most complex life-cycles among trematodes: a description of Parvatrema margaritense (Chin) n. comb.(Gymnophallidae) possessing parthenogenetic metacercariae. Parasitology 132 (5), 733. doi: 10.1017/S0031182005009765
Gordon D. M., Rau M. E. (1982). Possible evidence for mortality induced by the parasite Apatemon gracilis in a population of brook sticklebacks (Culaea inconstans). Parasitology 84 (1), 41–47. doi: 10.1017/S0031182000051647
Heasman M. P., O’Connor W. A., Frazer A. W. J. (1996). Digenean (Bucephalidae) infections in commercial scallops, Pecten fumatus reeve, and doughboy scallops, Chlamys (Mimachlamys) asperrima (Lamarck), in Jervis bay, new south Wales. J. Fish. Dis. 19 (5), 333–339. doi: 10.1111/j.1365-2761.1996.tb00371.x
Hong H.-K., Donaghy L., Kang C.-K., Kang H.-S., Lee H.-J., Park H.-S., et al. (2016). Substantial changes in hemocyte parameters of Manila clam Ruditapes philippinarum two years after the hebei spirit oil spill off the west coast of Korea. Mar. pollut. Bull. 108 (1-2), 171–179. doi: 10.1016/j.marpolbul.2016.04.033
Jacobson K. C., Teel D., Van Doornik D. M., Casillas E. (2008). Parasite-associated mortality of juvenile pacific salmon caused by the trematode Nanophyetus salmincola during early marine residence. Mar. Ecol. Prog. Ser. 354, 235–244. doi: 10.3354/meps07232
Jung B.-K., Chang T., Shin H., Ryoo S., Hong S., Lee J., et al. (2021). Parvatrema duboisi (Digenea: Gymnophallidae) lif cycle stages in Manila clams, Ruditapes philippinarum, from aphae-do (Island), shinan-gun, Korea. Korean J. Parasitol. 59, 83–88. doi: 10.3347/kjp.2021.59.1.83
Karatayev A. Y., Mastitsky S. E., Burlakova L. E., Karatayev V. A., Hajduk M. M., Comm D. B. (2012). Exotic molluscs in the great lakes host epizootically important trematodes. J. Shellfish Res. 31, 885–894. doi: 10.2983/035.031.0337
Kim Y.-G., Chun S.-K. (1981). A trematode, cercaria tapidis parasitic in the nautral stock of Tapes philippinarum. bull. Korean Fish. Soc 14 (4), 217–220.
Kim Y., Powell E. N. (2007). Distribution of parasites and pathologies in sentinel bivalves: NOAA status and trends “Mussel watch” program. J. Shellfish Res. 26 (4), 1115–1151. doi: 10.2983/0730-8000(2007)26[1115:DOPAPI]2.0.CO;2
Kim Y., Powell E. N., Ashton-Alcox K. A. (2006). Histopathology analysis. histological techniques for marine bivalve molluscs: Update. NOAA Tech. Mem. NOS NCCOS 27, 19–52.
Kim Y.-G., Yu J.-E., Chung E.-Y., Chung P.-R. (2004). Acanthoparyphium tyosenense (Digenea: Echinostomatidae): experimental confirmation of the cercaria and its complete life history in Korea. J. Parasitol. 90 (1), 97–102. doi: 10.1645/GE-3145
Kluytmans J. H., Brands F., Zandee D. I. (1988). Interactions of cadmium with the reproductive cycle of Mytilus edulis l. Mar. Environ. Res. 24 (1–4), 189–192. doi: 10.1016/0141-1136(88)90296-6
Kuris A. M., Lafferty K. D. (1994). Community structure: larval trematodes in snail hosts. Annu. Rev. Ecol. Syst. 25, 189–217. doi: 10.1146/annurev.es.25.110194.001201
Lafferty K. D., Morris A. K. (1996). Altered behavior of parasitized killifish increases susceptibility to predation by bird final hosts. Ecology 77 (5), 1390–1397. doi: 10.2307/2265536
Lassalle G., de Montaudouin X., Soudant P., Paillard C. (2007). Parasite co-infection of two sympatric bivalves, the Manila clam (Ruditapes philippinarum) and the cockle (Cerastoderma edule) along a latitudinal gradient. Aquat. Living Resour. 20 (1), 33–42. doi: 10.1051/alr:2007013
Lasiak T. (1992). Bucephalid trematode infections in mytilid bivalves from the rocky intertidal of southern Chile. J. Molluscan Stud. 58 (1), 29–36. doi: 10.1093/mollus/58.1.29
Le T. C., Kang H.-S., Hong H.-K., Park K.-J., Choi K.-S. (2015). First report of urosporidium sp., a haplosporidian hyperparasite infecting digenean trematode Parvatrema duboisi in Manila clam, Ruditapes philippinarum on the west coast of Korea. J. Invertebr. Pathol. 130, 141–146. doi: 10.1016/j.jip.2015.08.004
Littlewood D. T. J. (2006). “The evolution of parasitism in flatworms,” in Parasitic flatworms: molecular biology, biochemistry, immunology and physiology. Eds. Maule A. G., Marks N. J. (Wallingford: CABI Publishing), 1–36.
Magalhães L., Daffe G., Freitas R., de Montaudouin X. (2020). Monorchis parvus and Gymnophallus choledochus: two trematode species infecting cockles as first and second intermediate host. Parasitology 147 (6), 643–658. doi: 10.1017/S0031182020000402
Magalhães L., de Montaudouin X., Correia S., Daffe G., Gonzalez P., Figueira E., et al. (2019). Seasonal variation of transcriptomic and biochemical parameters of Donax trunculus related to its infection by Bacciger bacciger (trematode parasite). Estuar. Coast. Shelf Sci. 219, 291–299. doi: 10.1016/j.ecss.2019.02.012
Magalhães L., Freitas R., de Montaudouin X. (2015). Bucephalus minimus, a deleterious trematode parasite of cockles cerastoderma spp. Parasitol. Res. 114 (4), 1263–1278. doi: 10.1007/s00436-015-4374-6
Markowitz K. N., Williams J. D., Krause M. K. (2016). Development of quantitative PCR assay for detection of the trematode parasite Proctoeces maculatus in the blue mussel Mytilus edulis. dis. Aquat. Org. 122 (2), 125–136. doi: 10.3354/dao03072
Meng X., Tan Y., Yang W., Rbbani G., Yan X., Fang L., et al. (2019). Gonad status and gene expression of the Manila clam Ruditapes philippinarum infected by a digenetic trematode. J. Shellfish Res. 38 (2), 271–278. doi: 10.2983/035.038.0207
Miura O., Chiba S. (2007). Effects of trematode double infection on the shell size and distribution of snail hosts. Parasitol. Int. 56 (1), 19–22. doi: 10.1016/j.parint.2006.10.002
Morgan E., O’Riordan R. M., Kelly T. C., Culloty S. C. (2012). Influence of disseminated neoplasia, trematode infections and gametogenesis on surfacing and mortality in the cockle Cerastoderma edule. Dis. Aquat. Org. 98, 73–84. doi: 10.3354/dao02428
Navas J. I., Castillo M. C., Vera P., Ruiz-Rico M. (1992). Principal parasites observed in clams, Ruditapes decussatus (L.), Ruditapes philippinarum (Adams et Reeve), Venerupis pullastra (Montagu) and Venerupis aureus (Gmelin), from the Huelva coast (SW Spain). Aquaculture 107 (2-3), 193–199. doi: 10.1016/0044-8486(92)90067-U
Ngo T. T. T., Choi K.-S. (2004). Seasonal changes of Perkinsus and Cercaria infections in the Manila clam Ruditapes philippinarum from jeju, Korea. Aquaculture 239 (1-4), 57–68. doi: 10.1016/j.aquaculture.2004.06.026
Noble E. R., Noble G. A. (1971). Parasitology, the biology of animal parasites (Philadelphia: Lea and Febiger).
Paladini G., Longshaw M., Gustinelli A., Shinn A. P. (2017). “Parasitic diseases in aquaculture: their biology, diagnosis and control,” in Diagnosis and control of diseases of fish and shellfish. Eds. Austin B., Newaj-Fyzul A. (Chichester: John Wiley & Sons, Ltd), pp.37–pp107.
Park K.-I., Choi K.-S. (2001). Spatial distribution of the protozoan parasite perkinsus sp. found in the Manila clams, Ruditapes philippinarum, in Korea. Aquaculture 203 (1-2), 9–22. doi: 10.1016/S0044-8486(01)00619-6
Park K.-l., Choi K.-S. (2004). Application of enzyme-linked immunosorbent assay for studying of reproduction in the Manila clam Ruditapes philippinarum (Mollusca: Bivalvia): I. quantifying eggs. Aquaculture 241 (1–4), 667–687. doi: 10.1016/j.aquaculture.2004.08.017
Park K.-I., Figueras A., Choi K.-S. (2006). Application of enzyme-linked immunosorbent assay (ELISA) for the study of reproduction in the Manila clam Ruditapes philippinarum (Mollusca: Bivalvia): II. Impacts of Perkinsus olseni on clam reproduction. Aquaculture 251 (2-4), 182–191. doi: 10.1016/j.aquaculture.2005.06.003
Park K.-l., Tsutsumi H., Hong J.-S., Choi K.-S. (2008). Pathology survey of the short-neck clam Ruditapes philippinarum occurring on sandy tidal flats along the coast of ariake bay, Kyushu, Japan. J. Invertebr. Pathol. 99 (2), 212–219. doi: 10.1016/j.jip.2008.06.004
Park K.-I., Yang H.-S., Kang D.-H. (2010). Density dependent growth and mortality of Manila clam Ruditapes philippinarum reared in cages in gomso-bay, Korea. Korean J. Malacol. 26 (1), 91–95.
Ponurovsky S. K., Yakovlev Y. M. (1992). The reproductive biology of the Japanese littleneck, Tapes philippinarum. J. Shellfish Res. 11, 265–277.
Powell E. N., Barber R. D., Kennicutt M. II, Ford S. E. (1999). Influence of parasitism in controlling the health, reproduction and PAH body burden of petroleum seep mussels. Deep Sea Res. I 46, 2053–2078. doi: 10.1016/S0967-0637(99)00035-7
Ray S. M. (1966). A review of the culture method for detecting Dermocystidium marinum with suggested modifications and precautions. In Proc. Natl. Shellfish Assoc. 54, 55–69.
Shelley C., Glazebrook J., Turak E., Winsor L., Denton G. (1988). Trematode (Digenea: Bucephalidae) infection in the burrowing clam Tridacna crocea from the great barrier reef. Dis. Aquat. Organ. 4, 143–147. doi: 10.3354/dao004143
Shimura S., Yoshinaga T., Wakabayashi H. (1982). Three marine cercariae in the clam Tapes philippinarum from lake hamana, Japan: Morphology and level of infection. Fish Pathol. 17 (2), 129–137. doi: 10.3147/jsfp.17.129
Shinn A., Pratoomyot J., Bron J., Paladini G., Brooker E., Brooker A. (2015). Economic impacts of aquatic parasites on global finfish production. Global Aquacult. Advocate 2015, 58–61.
Siah A., Pellerin J., Amiard J. C., Pelletier E., Viglino L. (2003). Delayed gametogenesis and progesterone levels in soft-shell clams (Mya arenaria) in relation to in situ contamination to organotins and heavy metals in the st. Lawrence river (Canada). Comp. Biochem. Physiol. - C Toxicol. Pharmacol. 135 (2), 145–156. doi: 10.1016/S1532-0456(03)00085-1
Skála V., Bulantová J., Walker A. J., Horák P. (2014). Insights into the development of Notocotylus attenuatus (Digenea: Notocotylidae) in Lymnaea stagnalis: from mother sporocyst to cercariae. Parasitol. Int. 63 (1), 94–99. doi: 10.1016/j.parint.2013.09.009
Sohn W.-M., Na B.-K., Cho S.-H., Lee W.-J. (2017). Prevalence and density of digenetic trematode metacercariae in clams and oysters from western coastal regions of the republic of Korea. Korean J. Parasitol. 55, 399–408. doi: 10.3347/kjp.2017.55.4.399
Sullivan J. T., Cheng T. C., Howland K. H. (1985). Studies on parasitic castration: castration of Ilyanassa obsoleta (Mollusca: Gastropoda) by several marine trematodes. Trans. Am. Microsc. Soc, 154–171. doi: 10.2307/3226423
Thieltges D. W. (2006a). Effect of infection by the metacercarial trematode Renicola roscovita on growth in intertidal blue mussel Mytilus edulis. Mar. Ecol. Prog. Ser. 319, 129–134. doi: 10.3354/meps319129
Uddin M. J., Jeung H.-D., Yang H.-S., Kim B.-K., Ju S.-J., Choi K.-S. (2013). Quantitative assessment of reproductive effort of the Manila clam Ruditapes philippinarum in a lagoon on jeju island (Korea) using enzyme-linked immunosorbent assay. Invertebr. Reprod. Dev. 57 (4), 316–324. doi: 10.1080/07924259.2013.793219
Uddin M. J., Yang H.-S., Choi K.-S., Kim H.-J., Hong J.-S., Cho M. (2010). Seasonal changes in Perkinsus olseni infection and gametogenesis in Manila clam, Ruditapes philippinarum, from Seonjaedo Island in Incheon, off the west coast of Korea. J. World Aquac Socc 41, 93–101. doi: 10.1111/j.1749-7345.2009.00337.x
Uddin M. J., Yang H.-S., Park K.-J., Kang C.-K., Kang H.-S., Choi K.-S. (2012). Annual reproductive cycle and reproductive efforts of the Manila clam Ruditapes philippinarum in incheon bay off the west coast of Korea using a histology-ELISA combined assay. Aquaculture 364–365, 25–32. doi: 10.1016/j.aquaculture.2012.07.006
Walne P. R., Mann R. (1975). “Growth and biochemical composition in ostrea edulis and crassostrea gigas,” in Ninth european marine biology symposium (Scotland, United Kingdom: Aberdeen University Press), 587–607.
Wegeberg A. M., Jensen K. T. (2003). In situ growth of juvenile cockles, Cerastoderma edule, experimentally infected with larval trematodes (Himasthla interrupta). J. Sea Res. 50 (1), 37–43. doi: 10.1016/S1385-1101(03)00042-X
Wilson R. A., Pullin P., Denison J. (1971). An investigation of the mechanism of infection by digenetic trematodes: the penetration of the miracidium of Fasciola hepatica into its snail host Lymnaea truncatula. Parasitology 63 (3), 491–506. doi: 10.1017/S003118200008001X
Keywords: Digenea, larval trematodes, Manila clam, gonad castration, histology, semiquantitative scale
Citation: Cho Y-G, Lee H-M, Kim J-H, Shin J-S, Jeung H-D and Choi K-S (2022) Effects of larval trematode parasitism on the reproductive capacity of Manila clam Ruditapes philippinarum in a sandy-mud tidal flat on the west coast of Korea. Front. Mar. Sci. 9:936520. doi: 10.3389/fmars.2022.936520
Received: 05 May 2022; Accepted: 29 June 2022;
Published: 22 July 2022.
Edited by:
Alex Ford, University of Portsmouth, United KingdomReviewed by:
Teresa Maria Coelho Baptista, Polytechnic Institute of Leiria, PortugalXavier De Montaudouin, Université de Bordeaux, France
Luísa Magalhães, University of Aveiro, Portugal
Copyright © 2022 Cho, Lee, Kim, Shin, Jeung and Choi. This is an open-access article distributed under the terms of the Creative Commons Attribution License (CC BY). The use, distribution or reproduction in other forums is permitted, provided the original author(s) and the copyright owner(s) are credited and that the original publication in this journal is cited, in accordance with accepted academic practice. No use, distribution or reproduction is permitted which does not comply with these terms.
*Correspondence: Kwang-Sik Choi, skchoi@jejunu.ac.kr