Long-term changes in zooplankton in the Changjiang estuary from the 1960s to 2020
- 1Key Laboratory of Marine Ecosystem Dynamics & Second Institute of Oceanography, Ministry of Natural Resources (MNR), Hangzhou, China
- 2Key Laboratory of Marine Ecological Monitoring and Restoration Technology, Ministry of Natural Resources (MNR), East China Sea Environmental Monitoring Center, State Oceanic Administration, Shanghai, China
- 3Marine Space Resources Management Technology Key Laboratory, Ministry of Natural Resources (MNR), Marine Academy of Zhejiang Province, Hangzhou, China
- 4Observation and Research Station of Yangtze River Delta Marine Ecosystems, Ministry of Natural Resources (MNR), Zhoushan, China
The Changjiang estuary (CJE) is a large estuary that is affected by multiple anthropogenic stressors and climate change. The long-term trend of zooplankton in the CJE is an important indicator of the ecological response to stressors. We applied the Mann–Kendall trend analysis and Pettitt test to detect the trend and breakpoints of the biomass of the large mesozooplankton (LMZ; 505–20 000 μm) in four seasons, abundance of main LMZ taxa in summer from the 1960s to 2020, and abundance of dominant species in summer from 2000 to 2020 in the CJE. Results showed that LMZ biomass increased significantly during spring and summer, and the breakpoints both occurred in the 1980s. After the breakpoint, the mean biomass increased from 142.88 to 429.42 mg/m3 in spring and from 296.28 to 723.92 mg/m3 in summer. After 2000, the abundance of Copepoda in summer increased by more than 10 times compared to the 1960s. Under the conditions of warming and increased dinoflagellate abundance in the CJE, the abundance of the warm-water and omnivorous small calanoid copepod Paracalanus aculeatus increased significantly. Meanwhile, the significant decrease in the abundance of the temperate brackish species Labidocera euchaeta was probably mainly related to warming. The rapid changes in LMZ biomass during the late 1980s and mid-1990s is probably the result of a combination of enhanced bottom–up support, reduced top–down pressure, and promotion of temperature. This study provides scientific evidence and insights into the adaptive management of the Changjiang Basin.
Introduction
Estuaries are located at the transition zone between a river and shelf, which are characterized by strong coastal nonlinear physical processes (current, wind, and tide), and receive terrestrial materials from mega-river systems, including abundant dissolved or particulate organic and inorganic matter (Ge et al., 2020a). Additionally, estuaries are affected by climate change (Giosan et al., 2014). Anthropogenic activities in river basins, especially agricultural and industrial drainage and damming, have resulted in reduced sediment and increased nutrient transport into estuaries in recent decades, such as in the Mississippi, Indus, Nile, and Changjiang estuaries (Giosan et al., 2014; Chen et al., 2021). Nitrogen (N), phosphorus (P), and silicon (Si) are the three major nutrients. Among these, N and P concentrations have increased in river systems and associated estuaries, mainly because of fertilization. The Si concentration remained relatively stable, but exceptions have been observed following hydrological perturbations in upper river catchments, such as damming and water diversion for irrigation (Ge et al., 2020a). Increasing nutrient inputs, changing relative nutrient ratios, decreasing sediment loads, and global warming affect the biogeochemical dynamics of estuarine ecosystems. Phytoplankton abundance increases, harmful algal blooms, and seasonal hypoxia have been detected in regions with large riverine inputs in summer when vertical stratification is the strongest over a year, for example, the northern Gulf of Mexico, Chesapeake Bay, Baltic Sea, and East China Sea (ECS; Zhang et al., 2021). The responses of zooplankton are inconsistent across estuaries and may be determined by different trophic interactions in the food web. For example, the abundance of the ctenophore Mnemiopsis leidyi increased and that of the copepod Acartia tonsa decreased in the Chesapeake Bay (Kimmel et al., 2012; Stone et al., 2018), and the zooplankton standing crop reduced in the Nile delta (Chen et al., 2021), while the abundance ratio of copepods to cladocerans changed in the San Francisco estuary (Lehman et al., 2010).
The Changjiang (Yangtze) River is one of the largest rivers in the world, delivering approximately 9.28 × 1011 m3/yr of freshwater and 3.51 × 108 tons/yr of sediments into the estuary (multi-year mean between 1951 and 2020 at the Datong hydrological gauging station) (CWRCMWR, 1951–2020). As the longest river in China, over 30% of its population lives within the Changjiang drainage basin (Zhang et al., 2021). Since the 1960s, population growth and economic development in the basin have resulted in a rapid increase in nutrient fluxes into the Changjiang estuary (CJE), particularly dissolved inorganic N (Chai et al., 2006; Wang et al., 2018). In addition, over the past 60 years, more than 50,000 dams have been built in drainage basins; the sediment load of the Changjiang River has decreased significantly in recent decades, particularly after the construction of the world’s largest dam, the Three Gorges Dam (TGD) in 2003 (Zhao et al., 2021). The ECS, where the CJE is located, is one of the most rapidly warming large marine ecosystems (Belkin, 2009). Similar to other typical estuaries that are severely impacted by anthropogenic activities and warming, the CJE and its adjacent waters have been suffering from serious eutrophication, nutrient ratio shifts, phytoplankton and harmful algal blooms, seasonal hypoxia (Li et al., 2018; Li et al., 2019), and deterioration of fishery resources and jellyfish blooms exacerbated by overfishing (Xian et al., 2005).
Mesozooplankton play an important role in linking primary production to fish production and are excellent sentinels for the response of oceanic biota to climate change (Dam, 2013; Rice et al., 2015; Steinberg and Landry, 2017). Warm-water species generally refer to marine organisms whose growth and reproductive temperature range is higher than 20°C, and whose monthly average water temperature in their natural distribution area is higher than 15°C, and includes subtropical and tropical species. Temperate species generally refer to marine species with a wide range of suitable temperatures for growth and reproduction (4–20°C) and a wide range of monthly average water temperatures (0–25 °C) in their natural distribution areas, and includes cold-temperate species and warm-temperate species. Several previous studies comparing historical data from the 1960s, 1980s, and early 2000s in the CJE indicated that under the impact of warming, the abundance of warm-temperate species [Euphausia pacifica (Euphausiacea), Euchaeta plana (Copepoda)] decreased, whereas that of warm-water species [Pseudeuphausia sinica (Euphausiacea), Lucifer intermedius (Decapoda), and Lucifer hanseni (Decapoda), Euchaeta concinna (Copepoda), Temora turbinata (Copepoda)] increased (Ma et al., 2009; Zhang et al., 2010; Gao and Xu, 2011; Xu et al., 2013; Xu and Zhang, 2014). In addition, based on data in the CJE from 1996 to 2005, Li et al. (2010) revealed that the dominance of copepods decreased, while that of medusae increased. However, overall changes in the zooplankton community (including community biomass, main taxa, and dominant species) have not been reported in the CJE, especially when combined with data from the last two decades. We speculated that zooplankton biomass may increase under the background of warming, food increase, and overfishing; meanwhile, the abundance of warm-water and hypoxic-tolerant species may increase under warming and hypoxic stress.
Based on the above hypothesis and available data, we examined (1) the trend of zooplankton biomass in the four seasons from the 1960s to 2020, (2) the trend of abundance of zooplankton taxa in summer from the 1960s to 2020, and (3) the trend of abundance of dominant species of zooplankton in summer from 2000 to 2020. These results will provide an understanding of the response of mesozooplankton communities to the combined impacts of global warming and human perturbations in a large subtropical estuary and identify the factors that influence the changes in zooplankton characteristics.
Materials and methods
Study area
The CJE and inner shelf of the ECS are typical estuarine-shelf coastal zones with complicated hydrological systems, which are mainly affected by Changjiang Diluted Water (CDW), coastal currents, and the Taiwan Warm Current. CDW brings a large input of freshwater and sediment from the upstream. The mixing of freshwater with oceanic water forms a low-salinity plume. This plume changes seasonally in terms of its spreading areas and pathways, flowing either into the shelf of ECS during summer or farther away along the ECS coast during winter. A large quantity of suspended sediments from the river experience the dynamics of local vertical resuspension and settle in the estuary to form a sediment front (SF), which is jointly determined by the low-salinity plume and tidal mixing (Ge et al., 2020b; Li et al., 2021). A sediment plume usually co-occurs with a dissolved nutrient plume and a low-salinity plume. Sediment and dissolved nutrient fronts are located near the river mouth, whereas the low-salinity front (LSF) extends offshore over the shelf (Li et al., 2021; Figure 1A).
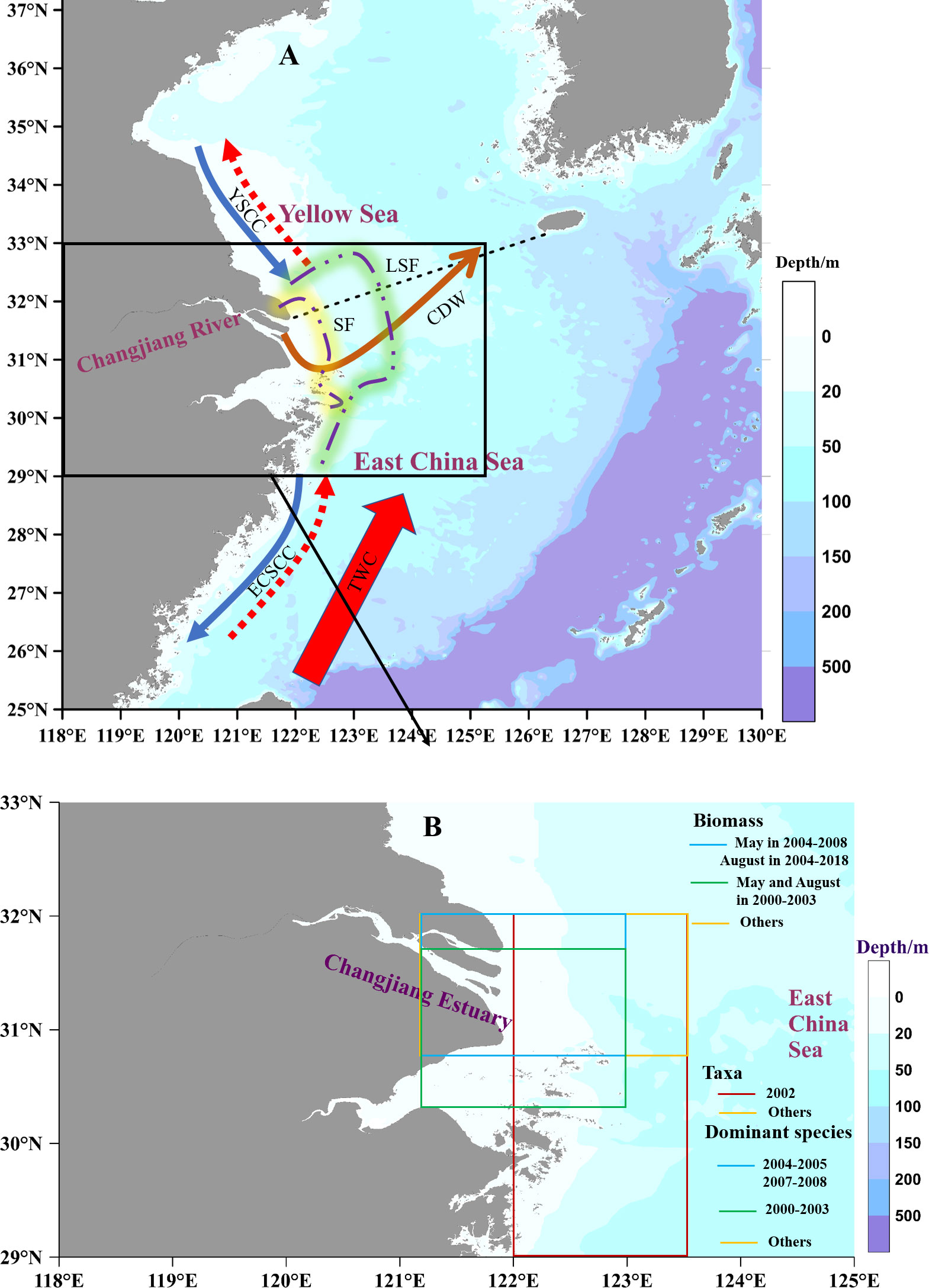
Figure 1 Hydrological system around the Changjiang estuary (A) and sampling areas of historical zooplankton data in Table 1 (B). CDW: Changjiang Diluted Water; YSCC: Yellow Sea Coastal Currents, ECSCC: East China Sea Coastal Currents, blue solid and red dotted arrows indicate the different seasonal flow directions of Coastal Currents; TWC: Taiwan Warm Current; SF: Sediment Front; LSF: Low-Salinity Front.
Estuarine fronts are important mesoscale physical processes that shape spatial patterns of different water masses. The water masses on either side of the front are characterized by different water properties, generating different spatial patterns of primary productivity and habitats for plankton assemblages. In the CJE and its adjacent waters, the shoreward water mass of the SF was characterized by the highest nutrients and total suspended matter (TSM). Consequently, the extensive light limitation led to the lowest chlorophyll-a (Chl-a). The water mass between the SF and LSF displayed the highest Chl-a, which benefited from the increased light availability and higher nutrients contributed by the plume and coastal upwelling. The shelf water mass beyond the LSF showed the lowest TSM and nutrients, and hence, the distinct reduction in nutrient supply resulted in relatively low Chl-a (Li et al., 2021).
Zooplankton near the CJE are usually divided into three or four groups, depending on the preference of species for water temperature and salinity. Freshwater species (e.g., Sinocalanus sinensis) can only be found inside the river mouth and are usually scarce. Estuarine species (e.g., Pseudodiaptomus sp. and Tortanus sp.) live near the river mouth, where salinity varies from 6 to 20. Oceanic species from the Yellow and East China Seas (e.g., Calanus sinicus, Euchaeta spp., Paracalanus spp., and Sagitta enflata) usually appear offshore. Shelf species (Acartia pacifica, Centropages dorsispinatus, Labidocera euchaeta, Sagitta nagae, and Diphyes chamissonis) live between estuarine and oceanic species, and those from neritic areas of both the Yellow and East China Seas can be observed in this area (Gao and Zhang, 1992).
On the other hand, the Changjiang River is affected by the East Asian monsoon, which results in higher rainfall in summer and lower rainfall in winter. The maximum runoff occurs from May to October, contributing 71.8% of the annual flow recorded at the Datong station, the last station before the river enters the coast, approximately 600 km upstream of the river mouth. The operation mode of the TGD changed the seasonal discharge at the river mouth, and reduced peak flooding in the summer, while increased the flow in winter.
Description of datasets
A survey of the zooplankton community in the CJE began in 1959, during the China National Oceanic Census. Since then, surveys, including on zooplankton, have been conducted every decade, except in the 1990s. In addition, zooplankton monitoring observations in the summer have been conducted almost every year since 2000. All zooplankton samples were collected using a plankton net with a mesh size of 505 μm (diameter: 50 cm and length: 145 cm when the water depth was < 30 m; diameter: 80 cm and length: 280 cm when the water depth was > 30 m). Therefore, in this study, we analyzed the long-term changes of the designated “large mesozooplankton” which range in size from 505 to 20,000 μm (generally, mesozooplankton are zooplankton ranging in size from 200 to 20,000 μm).
Zooplankton data were derived from the literatures covering a similar region and our studies (Figure 1B). Historical data included zooplankton biomass from 1959 to 2017 in spring and winter, 1959 to 2020 in summer, and 1959 to 2010 in autumn. Historical data also included the abundance of zooplankton taxa in summer from 1961 to 2020, and the abundance of dominant species in summer from 2000 to 2020, although there were several missing values (Table 1). Eleven zooplankton taxa were collected; unfortunately, with many missing values. Data on Copepoda and Euphausiacea covered the 1960s, 1980s, 2000s, and the 2010s. Data on Mysidacea, Amphipoda, and Decapoda covered the 1960s, 2000s, and 2010s. Data on Chaetognatha, Tunicata, Diplostraca, Medusa (including Hydrozoa and Ctenophora), Polychaeta, and planktonic larvae only covered the 2000s and the 2010s. Zooplankton data representing spring, summer, autumn, and winter were collected in May, August, November, and January, respectively.
Zooplankton samples were collected using plankton nets via vertical tows from 2 m above the bottom to the surface. The collected samples were stored in 5% formalin in 1-L plastic bottles. The volume of the filtered water was measured using a digital flow meter. In the laboratory, mesozooplankton samples were filtered through a silk sieve with a mesh size of 160 μm and then weighed with a 0.1-mg electronic balance after picking out sundries. Taxonomic identification and enumeration were performed using a stereoscope and microscope. Zooplankton biomass was determined as the ratio of the zooplankton wet weight to the filtered water volume, and abundance was determined as the ratio of the number of individuals to the filtered water volume. Dominant species were defined as those with a dominance index (Y) greater than 0.02. Y= (ni/N) ×fi , where ni represents the abundance of the species i, N is the total abundance of the community, and fi is the appearance frequency at all of the stations (Dufrene and Legendre, 1997).
The seasonal mean sea surface temperature (SST) from 1982 to 2020 was derived from level-4 daily products from multiple sensors including Advanced Very High Resolution Radiometers (AVHRRs), the series of Along Track Scanning Radiometers (ATSRs), and the Sea and Land Surface Temperature Radiometer (SLSTR) (2.1 version with 0.05° × 0.05° resolution; https://cds.climate.copernicus.eu/cdsapp#!/dataset/satellite-sea-surface-temperature?tab=form).
Statistical analyses
Trend analyses of annual zooplankton biomass in four seasons and abundance of taxa and dominant species in summer were performed using the nonparametric Mann–Kendall test (M–K test), which is widely used to detect monotonic increasing or decreasing trends of time series. Test S was exported to indicate a monotonic trend when the number of years n ≤ 10, whereas Test Z was exported to indicate a monotonic trend when n > 10. Sen’s nonparametric method was used to estimate the true slope of the existing trend (Salmi et al., 2002). Differences were considered statistically significant at p< 0.05. The Pettitt test was employed to determine whether at least one “breakdown-type” discontinuity existed in the time series. The time series were treated as nonstationary when the Pettitt test detected a change point in Pettitt test at p< 0.05. The nonstationary time series was further divided into subsets using the change points as breakpoints, and the nonparametric Kruskal–Wallis test (K–W test) was performed to confirm a shift in the mean value. K–W tests were also used to detect the differences in abundance of zooplankton taxa among decades. Spearman nonparametric tests were used to analyze the correlation between zooplankton biomass and SST. The M–K test, Sen’s slope estimation, and Pettitt test were executed in R using “zyp” and “trend” packages (R Development Core Team, 2014) (http://cran.r-project.org). The K–W test and Spearman test were calculated in SPSS 20.0.
Results
Trends of zooplankton biomass in four seasons
The annual mean zooplankton biomass for the years 1959, 1960, 1961, 1973, 1982/1983, 1985/1986, and 2006/2007 showed a gradual upward trend, from 109.59 mg/m3 around 1960 to 150.25 mg/m3 in the early 1970s and from 183.90 mg/m3 in the mid-1980s to 298.04 mg/m3 in the mid-2000s (Figure 2A; Table 2).
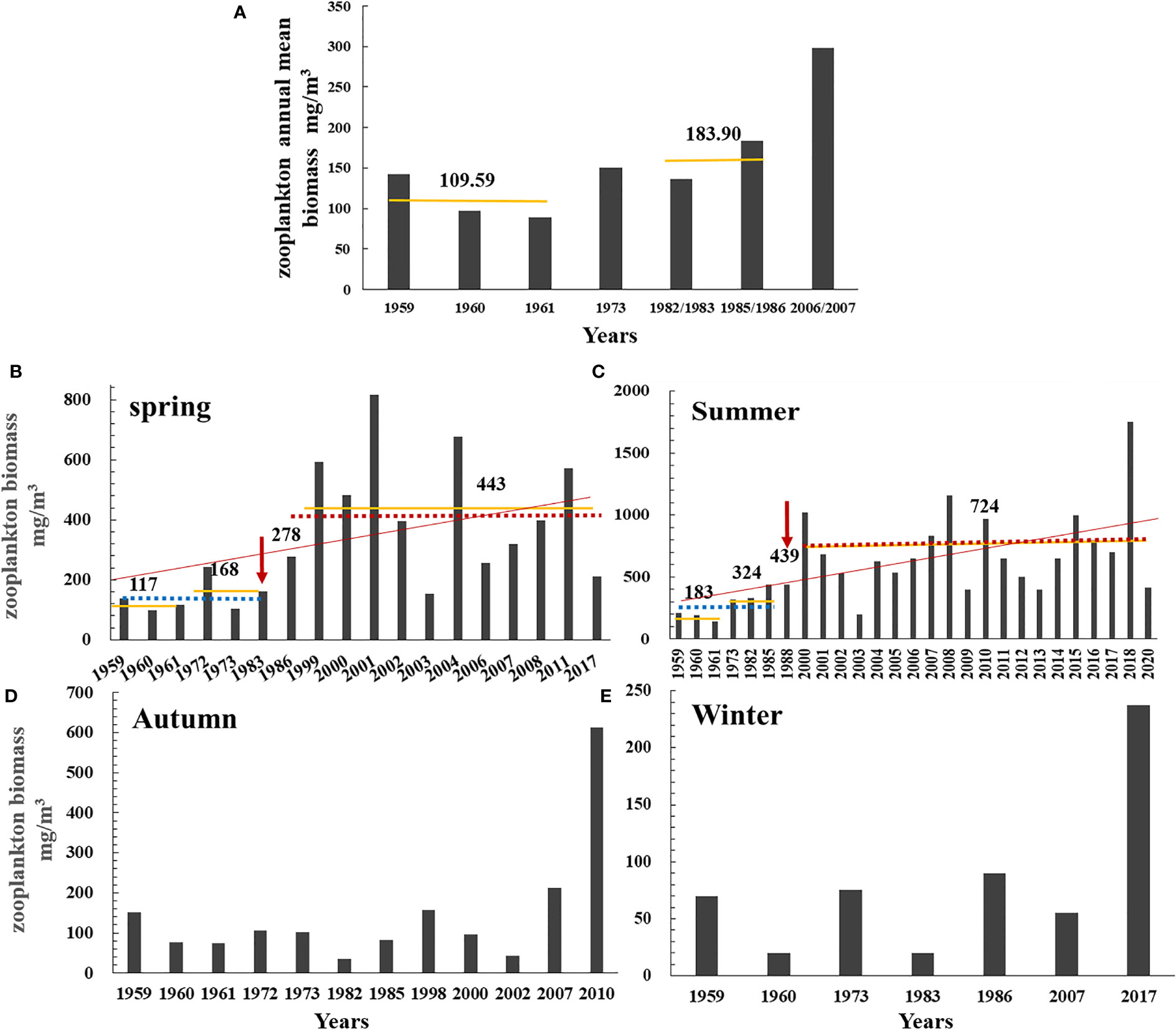
Figure 2 The annual mean biomass of zooplankton from 1959 to 2007 (A) and zooplankton biomass in spring from 1959 to 2017 (B), in summer from 1959 to 2020 (C), in autumn from 1959 to 2010 (D), and in winter from 1959 to 2017 (E) in the Changjiang estuary. Red line: trend line. Yellow line and the value above it: the mean biomass over a period. Red arrow: change point (breakpoint). Blue dotted line: mean biomass before breakpoint; red dotted line: mean biomass after breakpoint.
Long-term data on zooplankton biomass for the four seasons showed significant increasing trends during spring and summer at rates of 15.55 and 23.32 mg/m3/yr, respectively, according to Sen’s slope of the time series (pspring< 0.05; psummer< 0.01). The biomass in spring increased from 117 mg/m3 around 1960 to 168 mg/m3 from the early 1970s to early 1980s, and from 278 mg/m3 in the late 1980s to 443 mg/m3 from the end of 1990s to the present. The biomass in summer increased from 183 mg/m3 around 1960 to 324 mg/m3 from the early 1970s to early 1980s, and from 439 mg/m3 in the late 1980s to 724 mg/m3 from the end of 1990s to the present. The Pettitt test revealed 1983 and 1988 as the breakpoints of biomass during spring for the period 1959–2017 (p = 0.013) and during summer for the period 1959–2020 (p = 0.001), respectively (Figures 2B, C). After the breakpoint, the mean biomass increased from 142.88 to 429.42 mg/m3 in spring (p = 0.002 in the K–W test) and from 296.28 to 723.92 mg/m3 in summer (p = 0.001 in the K–W test), or approximately 200% and 144% increased after the breakpoint in spring and summer, respectively (Table 2). However, no obvious monotonic long-term trend in biomass for autumn and winter was detected (Figures 2D, E; Table 2).
Trends of abundance of zooplankton groups in summer
Long-term changes in the abundance of 11 zooplankton taxa in summer were analyzed. Based on the time span of data, the monotonic trends of abundance were examined for Copepoda and Euphausiacea, while the differences in abundance between decades were examined for the other taxa. Results showed significant increasing trends in the abundances of Copepoda and Euphausiacea, according to Sen’s slope of the time series (pCopepoda< 0.01; pEuphausiacea< 0.05). The Pettitt test revealed 1984 as the breakpoint of the abundance of Copepoda (p = 0.012) and Euphausiacea (p = 0.050) (Figures 3A, B). After the breakpoint, the mean abundance of Copepoda and Euphausiacea increased from 46.55 to 493.12 ind/m3 and from 4.45 to 15.51 ind/m3, respectively. However, the K–W test showed that the differences in the abundance of Copepoda (p = 0.064) and Euphausiacea (p = 0.077) were not significant before and after the breakpoint (Table 2). In addition, there was no obvious interdecadal variation in the abundances of Mysidacea (p = 0.888), Amphipoda (p = 0.117), Decapoda (p = 0.304), Medusa (p = 0.180), Chaetognatha (p = 0.083), Tunicata (p = 0.180), Diplostraca (p = 0.655), Polychaeta (p = 0.180), and planktonic larvae (p = 0.655; Figures 3C–K; Table 3).
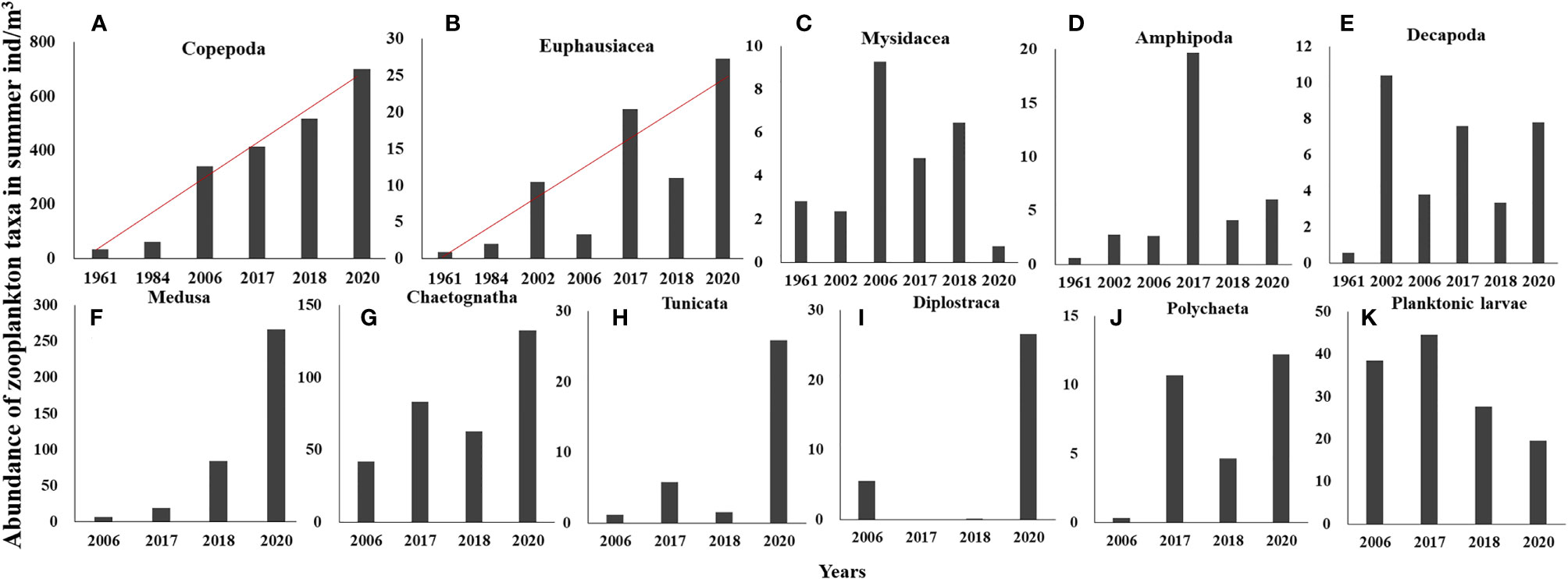
Figure 3 Abundance of Copepoda (A) and Euphausiacea (B) in summer in the 1960s, 1980s, 2000s, and 2010s; abundance of Mysidacea (C), Amphipoda (D), and Decapoda (E) in summer in the 1960s, 2000s, and 2010s; abundance of Medusa (including Hydrozoa and Ctenophora) (F), Chaetognatha (G), Tunicata (H), Diplostraca (I), Polychaeta (J), Planktonic larvae (K) in summer in the 2000s and 2010s. Red line: trend line. The different scales of the Y-axis are adjusted for different taxa abundances.
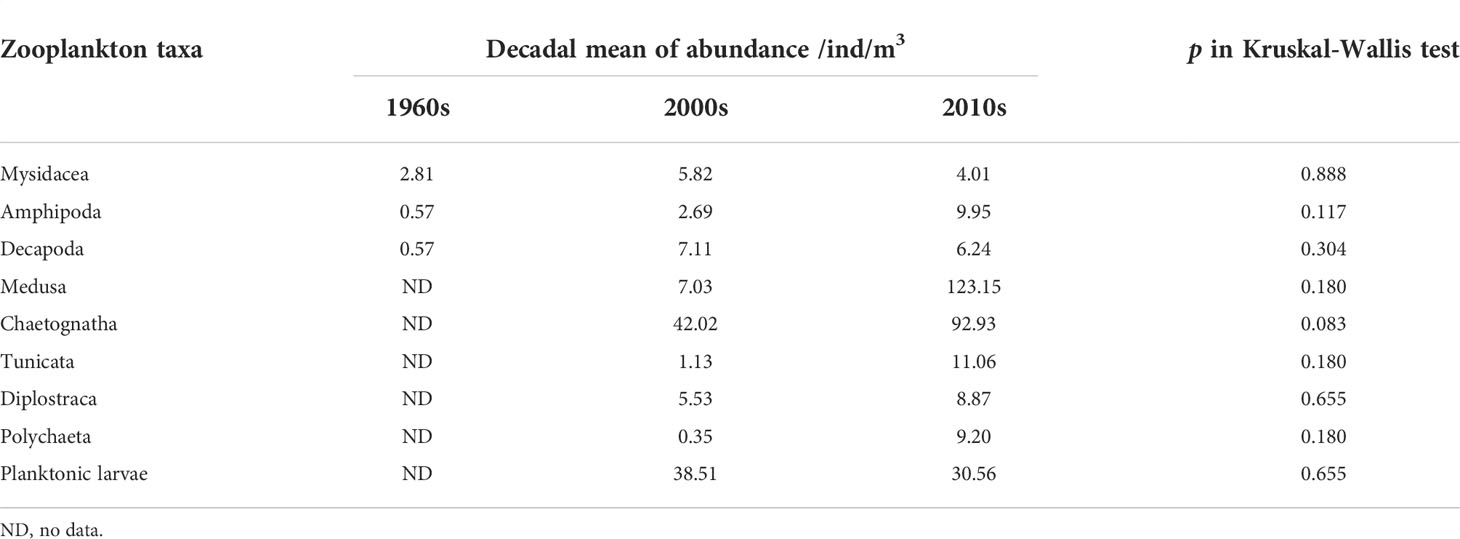
Table 3 Interdecadal differences in average abundance of zooplankton taxa in the Changjiang estuary.
Trends of abundance of dominant species in summer
In the CJE, in summer, the dominant species of Copepoda were A. pacifica, C. dorsispinatus, C. sinicus, L. euchaeta, P. aculeatus, and Euchaeta spp. (Euchaeta concinna and large numbers of Euchaeta larvae). During 2000–2020, the monotonic trend of the abundance of the dominant species P. aculeatus was significantly increasing and it was significantly decreasing for L. euchaeta based on the Sen’s slope of the time series (pP. aculeatus< 0.05; pL. euchaeta< 0.01). The Pettitt test revealed 2008 and 2005 as the breakpoints of abundance of P. aculeatus (p = 0.005) and L. euchaeta (p = 0.006), respectively (Figures 4D, E). After the breakpoint, the mean abundance of P. aculeatus increased from 9.10 to 75.07 ind/m3 (p = 0.009 in the K–W test), while the mean abundance of L. euchaeta decreased from 59.72 to 9.25 ind/m3 (p = 0.004 in the K–W test) (Table 2). In addition, the abundance of A. pacifica increased slightly at a non-significant level with 2006 as the breakpoint (p = 0.038) (Figure 4A; Table 2). The mean abundance of A. pacifica before and after the breakpoint was 63.10 and 177.82 ind/m3 respectively, (p = 0.032 in the K–W test). However, there was no obvious long-term trend in the abundance of the other dominant species of Copepoda (Figures 4B, C, F; Table 2).
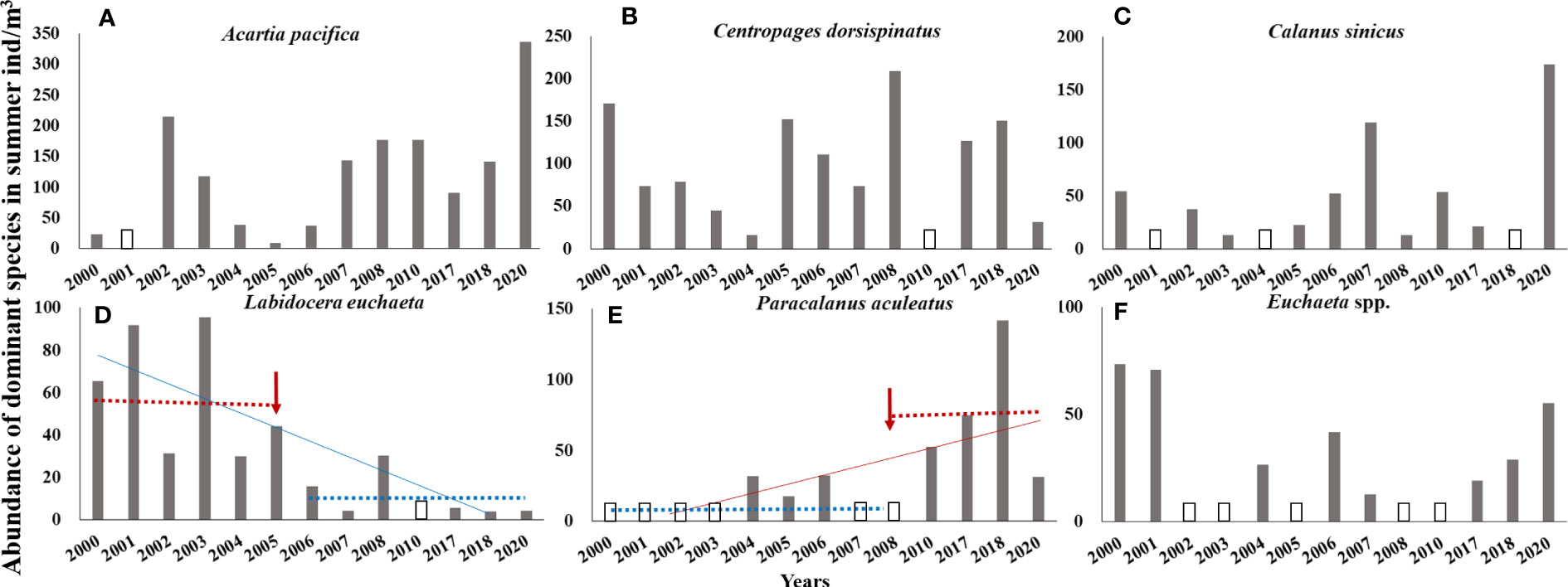
Figure 4 Abundance of Acartia pacifica (A), Centropages dorsispinatus (B), Calanus sinicus (C), Labidocera euchaeta (D), Paracalanus aculeatus (E), and Euchaeta spp. (F) in summer from 2000 to 2020. Red and blue lines: trend lines. Red arrow: change point (breakpoint). Blue dotted line: mean abundance before/after breakpoint; red dotted line: mean abundance before/after breakpoint. An empty column indicates that the species is not the dominant species in a given year. Euchaeta spp. includes Euchaeta concinna and large numbers of Euchaeta larvae.
Discussion
Significant increase in biomass
The annual average biomass of zooplankton in the CJE increased by 1.68 times from around 1960 to mid-1980s, and by 2.72 times from around 1960 to the mid-2000s. In terms of seasons, we found that zooplankton biomass from the 1960s to 2020 increased significantly during spring and summer, and breakpoints occurred in the 1980s. Biomass in spring increased by 1.67, 3.87, and 3.33 times from around 1960 to 1970s–1980s, 2000s, and 2010s, respectively. Similarly, biomass in summer increased by 2.08, 3.63, and 4.28 times from around 1960 to 1970s–1980s, 2000s, and 2010s, respectively. Owing to the lack of historical data for the early to mid-1990s, we can only identify the shift in zooplankton biomass occurring between the late 1980s and the mid-1990s. This period coincided with the rapid increase in N and P in the Changjiang Basin caused by the extensive application of fertilizers, rapid development of industry and urbanization after 1980, and the rapid rise in water temperature since 1982 in the ECS (Belkin, 2009; Wang et al., 2021).
N- and P-fertilizer application in the Changjiang Basin increased gradually from 1–2 × 106 t/yr and 0.2–0.5 × 106 t/yr during the 1960s–1970s to 7–8 × 106 t/yr and 2.5 × 106 t/yr at the end of the 1990s, respectively, and both were stable after 2000 because of changes in national policies (Wang et al., 2021). The rapid increase in N and P in the Changjiang Basin led directly to an increase in dissolved inorganic nitrogen (DIN) and dissolved inorganic phosphorus (DIP) in the CJE. DIN concentrations in the Datong station increased from 20 μmol/L in the 1960s to 65.1, 89.4, and 150 μmol/L in the 1980s, 2000s, and 2010, respectively, and then decreased slightly in the last decade. DIP concentrations increased from 0.5 μmol/L in the 1960s to 0.6, 1.1, and 1.4 μmol/L in the 1980s, 2000, and 2005, respectively, and then reduced to 1.0 µm/L after 2010 (Jiang et al., 2010; Wang et al., 2021). Instead, the dissolved silicate concentration continued to decline during this period, from 320 μmol/L in the early 1960s to 100 μmol/L after 2000, and it has been almost stable in the last two decades (Wang et al., 2021). Belkin (2009) found that the SST in the ECS was relatively stable during 1957–1981, but accelerated warming was observed from 1982 to 2003 at a rate of up to 1.0°C/decade. A significant warming trend was also detected in our study area during 1982–2020 in spring (p< 0.001), summer (p< 0.05), and autumn (p< 0.001), with warming rates of 0.52, 0.21, and 0.20 °C/decade, and the Pettitt test revealed breakpoints at 1996, 1993, and 1995 during spring (p< 0.01), summer (p< 0.05), and autumn (p< 0.01), respectively (Figure 5; Table 2).
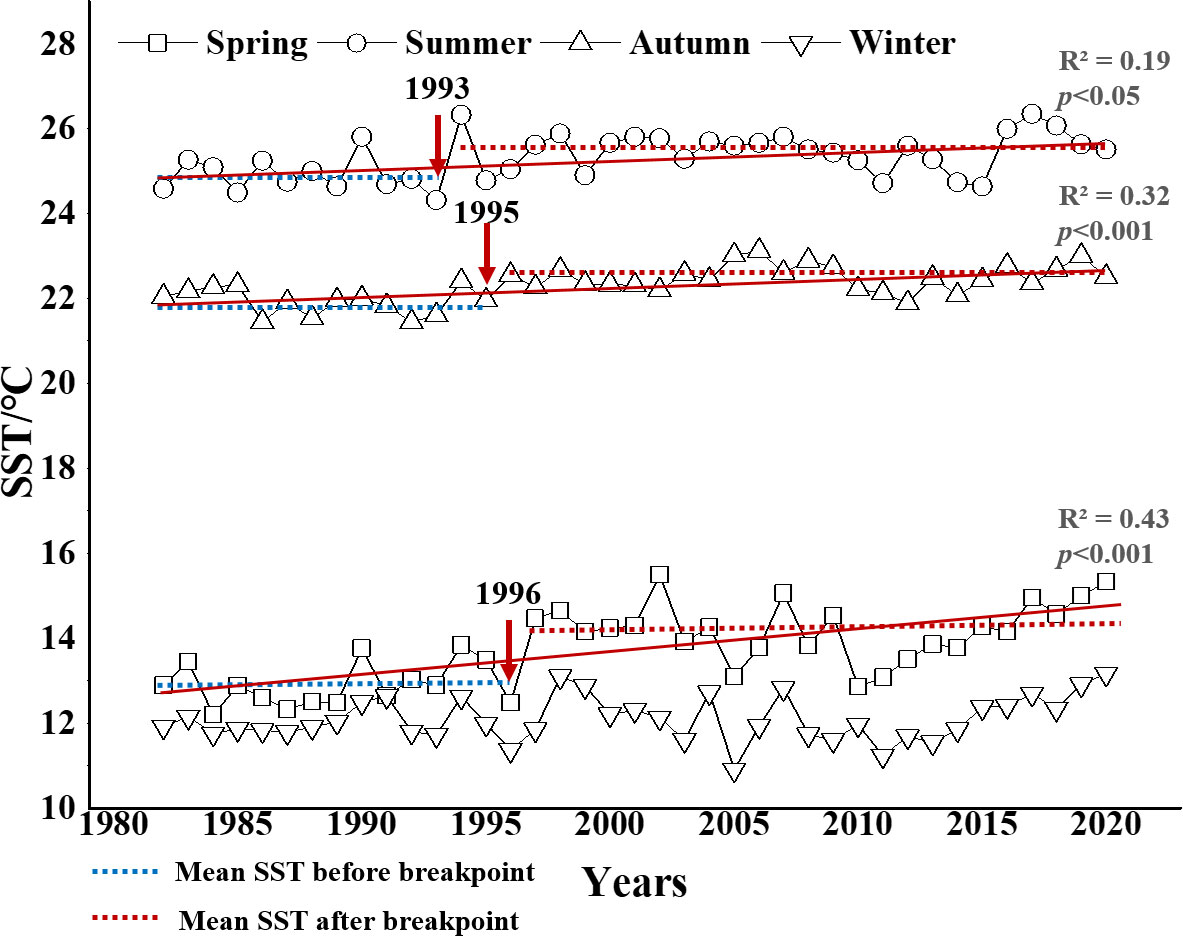
Figure 5 Sea surface temperature (SST) in the Changjiang estuary in four seasons from 1982 to 2020. Red line: trend line. Red arrow: change point (breakpoint). Blue dotted line: mean SST before breakpoint; red dotted line: mean SST after breakpoint.
Jiang et al. (2010) revealed that the year-round average phytoplankton abundance decreased from 4.7 × 104 cell/L in 1958–1959 to 2.6 × 104 cell/L in 1985–1986, and then increased significantly to 9.9 × 104 cell/L in 2004–2005 in the area that is consistent with this study (approximately twice from the end of the 1950s to the mid-2000s), due to the increase in DIN concentration. This upward trend seems to be consistent with that of the annual average zooplankton biomass from the early 1960s to the mid-2000s. Furthermore, phytoplankton abundance increased in all seasons during this period, with the largest increase in spring (from 3 × 102 cell/L to 2 × 105 cell/L), followed by winter (from 5 × 101 cell/L to 1 × 104 cell/L) and autumn (from 2 × 101 cell/L to 3 × 103 cell/L), while an increase of more than two orders of magnitude in summer occurred beyond the LSF (Jiang et al., 2010). Conversely, fishery resource density decreased significantly between 1960 and the early 2000s. The catch per fishing effort decreased from 636.26, 1130.55, and 659.26 kg/h in 1960 to 16.26, 26.23, and 222.01 kg/h in 2004 during spring, summer, and autumn, respectively (Li et al., 2007), and then to 4.44 kg/h in 2011 during spring (Ding, 2013). In terms of temperature effects, the Spearman nonparametric test showed that zooplankton biomass was positively correlated with SST in spring (R = 0.214, p = 0.482, and n = 13), summer (R = 0.416*, p = 0.049, and n = 23), and autumn (R = 0.607, p = 0.148, n = 7), and the correlation was significant in summer. Therefore, the increase in zooplankton biomass in the CJE is a combined effect of enhanced bottom–up support, reduced top–down pressure, and promotion of temperature. The increase in zooplankton biomass was gradual, but a rapid rise occurred between the late 1980s and the 1990s, when all these three effects changed significantly. Coincidentally, Rebstock and Kang (2003) revealed that the zooplankton biomass in the ECS increased gradually from the 1980s to the early 1990s and sharply in the 1990s as a response to climate change. The significant positive correlation between zooplankton biomass and SST in summer observed in this study combined with Rebstock and Kang (2003) results suggests that climate change probably plays an important role in the rapid change in zooplankton biomass in the CJE.
Additionally, the increases in zooplankton biomass were significant in spring and summer, but not in autumn and winter, which could not be ascertained clearly because of numerous missing data. The concentrations of DIN, DIP, and phytoplankton abundance in the four seasons increased to almost the same extent (Jiang et al., 2010), and the degree of warming in autumn was similar to that in summer (Table 2); however, the decrease in fishery resources in autumn was smaller than that in spring and summer (Li et al., 2007). Therefore, it is difficult to determine whether the non-significant increases in zooplankton biomass in autumn and winter were only due to the small sample size. More data are required for causal analysis.
Specific to the changing taxa in summer, significant increases were detected in the abundances of Copepoda and Euphausiacea, which continued to increase from the 1960s to 2020, but they were considerably higher after 2000 than before 2000. Furthermore, the degree of increase in the abundance of Copepoda was greater than that of Euphausiacea. The former was more than 10 times higher after 2000 than it was in the 1960s. Water temperature and food availability are the most important factors that affect the population dynamics of copepods (Ban, 1994; Devreker et al., 2005). The Chl-a concentration increased by only a factor of four (from 2 to 8 μg/L) from the 1980s to the 2000s (Wang, 2006; Jiang et al., 2014). We speculate that ocean warming was also an important cause of the increase in copepod abundance in the summer. Rebstock and Kang (2003) showed that the increase in the abundance of Copepoda, Chaetognatha, and Euphausiacea (both copepod predators) was synchronous with the increase in zooplankton biomass in the ECS due to warming. Based on these facts, we propose that the increase in zooplankton abundance was mainly due to the increase in crustaceans such as copepods in the CJE and ECS, and the effect of temperature cannot be ignored.
The increase in abundance of small copepods had limited contribution to the increase in biomass, due to their small size of individuals. However, small copepods are important food source for gelatinous zooplankton, e.g., small-jellyfish (Medusa in our study) and Chaetognatha (Wang et al., 2020a). The areas with high abundance of small copepods and Medusa overlapped near the CJE in summer (Gao et al., 2015). Therefore, the long-term increases in copepod abundance and SST might provide more abundant food and suitable temperature for gelatinous zooplankton. A study on trends in Large Marine Ecosystems showed that jellyfish populations increased obviously in the ECS and Yellow Sea ecosystems (Brotz et al., 2012). In addition, the abundance of small-jellyfish in Jiaozhou Bay (located in the Yellow Sea) increased by almost 4 times from the 1990s to 2000s (Sun et al., 2012). Our study also found that the abundance of Medusa and Chaetognatha in summer was higher in the 2010s than in the 2000s (Table 3), although the difference was not significant. Therefore, we speculated that the increase of zooplankton biomass in the CJE in summer might be related to the increase of gelatinous zooplankton.
Decrease in temperate species and increase in warm-water species
The effects of climate change on zooplankton have been discussed over several years. The trend in zooplankton biomass with warming was not consistent in different trophic ecosystems, but the expansion of warm-water species was almost uniform (Rebstock and Kang, 2003; Batten and Welch, 2004; Beaugrand, 2004; Sheridan and Landry, 2004; Hooff and Peterson, 2006; Wiafe et al., 2008; Piontkovski and Castellani, 2009; Keister et al., 2011; Rice et al., 2015). The impacts of climate change on China’s marine ecosystems have also been reviewed (Kang et al., 2021). Changes in zooplankton include a decrease in abundance of large-sized copepods (e.g., L. euchaeta and C. sinicus), northward expansion in distribution and an increase in abundance of small-sized copepods (e.g., A. pacifica and C. dorsispinatus) in the eutrophic temperate semi-enclosed Bohai Sea (Yang et al., 2018), northward migration of temperate species (e.g., C. sinicus, E. pacifica, and Sagitta crassa) and ctenophore Pleurobrachia globosa, blooms of Jellyfish and Thaliacea in the temperate continental shelf of Yellow Sea and subtropic continental shelf of the ECS (Xu et al., 2013; Shi et al., 2015; Xu and Zhang, 2014; Shi et al., 2016; Wang et al., 2020b), an increase in the abundance of small-sized copepods (e.g., Oncaea conifer), and species diversity in the tropic South China Sea (Wang et al., 2014; Gong et al., 2017).
In the present study, we found that the abundance of L. euchaeta and P. aculeatus during 2000–2020 significantly decreased and increased, respectively. L. euchaeta is a temperate brackish species with an optimum distribution temperature of approximately 16 °C and an optimum salinity of 12–20, whose abundance was higher in winter and spring, but lower in summer and autumn in the CJE (Xu and Gao, 2009). It has been reported that the abundance of L. euchaeta in spring, summer, and winter decreased significantly in 2002 compared to 1959 and this phenomenon was linked to warming (Xu and Gao, 2009). Our study reconfirmed the negative effects of warming on L. euchaeta based on data from the last two decades. This is the first report to show that the abundance of P. aculeatus increased significantly in the CJE. Paracalanus spp. are neritic, warm-water, small calanoid copepods, and could be found in the CJE in four seasons, with higher abundances in summer and spring (data from 2006 in this study). P. aculeatus was not a dominant species before 2004 in this area in summer, but it was the dominant species in 2004 and 2006, indicating that its abundance and relative abundance increased; however, the significance of this increase could not be determined (Zhang et al., 2010; data from 2006 in this study). The significant increase in the abundance of P. aculeatus was confirmed by data from the last two decades in this study. We propose that the increase in the abundance of P. aculeatus is related to its small size, warm-water preference, and feeding habits. Rice et al. (2015) found that an increase in temperature can benefit smaller-sized copepods in eutrophic systems where food was not limited, probably due to the allometric growth law and food availability. Measurements of the in situ egg production rate (EPR) of Paracalanus parvus revealed that EPR and weight-specific female growth were both strongly positively related to water temperature (Jang et al., 2013). On the other hand, Paracalanus spp. often ingest ciliates, dinoflagellates, and nanoflagellates in large quantities in addition to phytoplankton (Suzuki et al., 1999). This was also confirmed by the weak correlation between EPR and female growth of Paracalanus spp. and Chl-a concentration (Jang et al., 2013). Wang et al. (2021) showed the change in phytoplankton from siliceous to non-siliceous-dominated communities around 2000 along with the increase in species number and abundance of dinoflagellates due to excessive N and lowering of dissolved silicate. Therefore, the increase in abundance of dinoflagellates in the CJE can also be one of the reasons for the increase in P. aculeatus (Wang et al., 2021).
Hypoxia in the CJE started in late spring and early summer, became the most serious in August, decreased in autumn, and finally, disappeared in winter. Hypoxia is primarily associated with marine-sourced organic carbon production, which is stimulated by coastal eutrophication, resulting from excessive terrestrial nutrient runoff. The intensity, duration, and frequency of hypoxia has increased significantly in recent decades (Li et al., 2018). It has been documented that jellyfish can survive in low-oxygen water at the bottom, which allows them to avoid predators (Purcell et al., 2007; Purcell, 2012); and Acartia tonsa appears to be quite tolerant to low oxygen relative to the co-occurring copepod species Labidocera aestiva and Centropages hamatus (Stalder and Marcus, 1997). Our results show a continuous increase in Medusa abundance from 2006 to 2020 (Figure 3F), but without a significant trend owing to the short time span of data. A slight increase in abundance of A. pacifica was also detected in this study (Figure 4A). However, jellyfish also benefit from warming (Purcell, 2005; Wang et al., 2012); therefore, it is difficult to determine the effect of hypoxia on zooplankton composition by comparing historical data. A number of studies in the northern Gulf of Mexico and Chesapeake Bay showed that hypoxia can drive the size structure of the zooplankton community and the vertical distribution in the water column, but few studies have describe detailed taxonomic composition in relation to hypoxia (Elliott et al., 2012).
Conclusions
Long-term changes in large mesozooplankton (505–20,000 μm) in the CJE from the 1960s to 2020 were revealed in this study. Zooplankton biomass increased, and the regime shift occurred between the late 1980s and the mid-1990s, when nutrient concentrations, phytoplankton abundance, and SST increased synchronously, while fishery resource density decreased significantly. The abundance of Copepoda in summer increased significantly from the 1960s to 2020, and it was more than 10 times after 2000 compared to the 1960s. In the context of warming and increase in dinoflagellate abundance, the abundance of the warm-water and omnivorous small calanoid copepod P. aculeatus increased significantly, whereas that of the temperate brackish species L. euchaeta decreased mainly due to warming. However, it is difficult to determine the effects of hypoxia on long-term zooplankton changes. Moreover, some increasing or decreasing trends were not significant, such as zooplankton biomass in autumn and winter, and abundance of Medusa and Chaetognatha in summer, probably because of the small sample size. Hence, long-term monitoring is necessary for the adaptive management of the Changjiang Basin.
Data availability statement
The raw data supporting the conclusions of this article will be made available by the authors, without undue reservation.
Author contributions
PD wrote the draft. W-JY acquired zooplankton data during 2017–2020 and participated in zooplankton data collecting. B-PD collected zooplankton data during 2003–2018 in summer. MM acquired SST data. Y-LZ participated in data analysis in R software package. F-PC participated in zooplankton data collecting. Z-BJ revised the manuscript LS participated in the manuscript discussion. Q-ZC funded the work and composed the idea. All authors contributed to the article and approved the submitted version.
Funding
This work is supported by the National Key Research and Development Program of China (2018YFD0900901, 2018YFD0900905), the Open Research Fund of Marine Ecological Monitoring and Restoration Technology (MEMRT202106), the Open Research Fund of Key Laboratory of Marine Ecosystem Dynamics (MED202005), the Long-term Observation and Research Plan in the Changjiang Estuary and the Adjacent East China Sea Project (LORCE, No. 14282), Zhejiang Provincial Project (330000210130313013006), Key Research and Development Program of Zhejiang Province (2021C03186). and the Yangtze Delta Estuarine Wetland Ecosystem Observation and Research Station, Ministry of Education & Shanghai Science and Technology Committee (ECNU-YDEWS-2020).
Acknowledgments
We are grateful to Cheng-Gang Liu from second institute of oceanography, MNR for Figure 1 modification, and Wen-Wen Chen from Shanghai Ocean University for her help in collecting historical data.
Conflict of interest
The authors declare that the research was conducted in the absence of any commercial or financial relationships that could be construed as a potential conflict of interest.
Publisher’s note
All claims expressed in this article are solely those of the authors and do not necessarily represent those of their affiliated organizations, or those of the publisher, the editors and the reviewers. Any product that may be evaluated in this article, or claim that may be made by its manufacturer, is not guaranteed or endorsed by the publisher.
References
Ban S. (1994). Effect of temperature and food concentration on post-embryonic development, egg production and adult body size of calanoid copepod Eurytemora affinis. J. Plankton Res. 16 (6), 721–735. doi: 10.1093/plankt/16.6.721
Batten S., Welch D. (2004). Changes in oceanic zooplankton populations in the north-east pacific associated with the possible climatic regime shift of 1998/1999. Deep Sea Res. Part II: Topical Stud. Oceanogr. 51, 863–873. doi: 10.1016/j.dsr2.2004.05.009
Beaugrand G. (2004). The north Sea regime shift: Evidence, causes, mechanisms and consequences. Prog. Oceanogr. 60 (2), 245–262. doi: 10.1016/j.pocean.2004.02.018
Belkin I. M. (2009). Rapid warming of Large marine ecosystems. Prog. Oceanogr. 81 (1), 207–213. doi: 10.1016/j.pocean.2009.04.011
Brotz L., Cheung W. W. L., Kleisner K., Pakhomov E., Pauly D. (2012). Increasing jellyfish populations: trends in Large marine ecosystems. Hydrobiologia 690 (1), 3–20. doi: 10.1007/s10750-012-1039-7
Cai M., Xu Z. L., Zhu D. D. (2008). Distribution characteristics of pelagic amphipoda in the Changjiang estuary and adjacent waters. Acta Oceanolog. Sin. 30 (5), 81–87. doi: 10.3321/j.issn:0253-4193.2008.05.011
Chai C., Yu Z., Song X., Cao X. (2006). The status and characteristics of eutrophication in the Yangtze river (Changjiang) estuary and the adjacent East China Sea, China. Hydrobiologia 563, 313–328. doi: 10.1007/s10750-006-0021-7
Chen H., Xu Z. L. (2009). Seasonal distribution of pelagic ostracoda in the Changjiang estuary and its adjacent waters. Chin. J. Appl. Environ. Biol. 15 (1), 072–077. doi: 10.3724/SP.J.1145.2009.00072
Chen Z., Xu H., Wang Y. (2021). Ecological degradation of the Yangtze and Nile delta-estuaries in response to dam construction with special reference to monsoonal and arid climate settings. Water 13 (9): 1145. doi: 10.3390/w13091145
Chen J. J., Xu Z. L., Zhu D. D. (2008). Seasonal abundance and distribution of pelagic euphausiids in the Changjiang estuary, China. Acta Ecolog. Sin. 28 (11), 5279–5285. doi: 10.3321/j.issn:1000-0933.2008.11.009
Chen Y. Q., Zheng G. X., Zhu Q. Q. (1985). A preliminary study of the zoo plankton in the Changjiang estuary area. Donghai Mar. Sci. 3 (3), 53–61. doi: CNKI:SUN:DHHY.0.1985-03-008
CWRCMWR (Changjiang Water Resource Commission of the Minstry of Water Resource) (1951-2020). Internal report of Changjiang water and sediment bulltion (Wuhan, China: Yangtze Press).
Dam H. G. (2013). Evolutionary adaptation of marine zooplankton to global change. (Beijing, China: Science Press), 289.
Devreker D., Souissi S., Seuront L. (2005). Effects of chlorophyll concentration and temperature variation on the reproduction and survival of temora longicornis (Copepoda, calanoida) in the Eastern English channel. J. Exp. Mar. Biol. Ecol. 318, 145–162. doi: 10.1016/j.jembe.2004.12.011
Ding P. X. (2013). Evolution and cause analysis of typical coastal zones in China during the last 50 years. (Beijing, China: Science Press) ,289.
Dufrene M., Legendre P. (1997). Species assemblages and indicator species: the need for a flexible asymmetrical approach. Ecol. Monogr. 67, 345–366. doi: 10.1890/0012-9615(1997)067
Editorial board for marine atlas (EBMA) (1991). Marine atlas of Bohai Sea, Yellow Sea, East China Sea: Biology (Beijing, China: China Ocean Press), 91–187.
Elliott D., Pierson J., Roman M. (2012). Relationship between environmental conditions and zooplankton community structure during summer hypoxia in the northern gulf of Mexico. J. Plankton Res. 34, 602–613. doi: 10.1093/plankt/fbs029
Gao Q., Chen J. J., Xu Z. L., Zhu D. D. (2015). Abundance distribution and seasonal variation of medusae, siphonophores, and ctenophores in the Changjiang (Yangtze river) estuary and the adjacent East China Sea. Acta Ecolog. Sin. 35 (22), 7328–7337. doi: 10.5846/stxb201403210505
Gao Q., Xu Z. (2011). Effect of regional warming on the abundance of Pseudeuphausia sinica Wang et Chen (Euphausiacea) off the Changjiang river (Yangtze river) estuary. Acta Oceanolog. Sin. 30, 122–128. doi: 10.1007/s13131-011-0169-5
Gao S. W., Zhang H. Q. (1992). On the ecology of zooplankton in the Changjiang river estuary. Stud. Mar. Sin. 33, 201–216.
Ge J., Shi S., Jie L., Xu Y., Chen C., Bellerby R., et al. (2020a). Interannual variabilities of nutrients and phytoplankton off the Changjiang estuary in response to changing river inputs. J. Geophys. Res.: Oceans 125 (3), e2019JC015595. doi: 10.1029/2019JC015595
Ge J., Torres R., Chen C., Jie L., Xu Y., Bellerby R., et al. (2020b). Influence of suspended sediment front on nutrients and phytoplankton dynamics off the Changjiang estuary: A FVCOM-ERSEM coupled model experiment. J. Mar. Syst. 204, 103292. doi: 10.1016/j.jmarsys.2019.103292
Giosan L., Syvitski J., Constantinescu S., Day J. (2014). Climate change: Protect the world’s deltas. Nature 516, 31–33. doi: 10.1038/516031a
Gong Y. Y., Yang Y. T., Fang J. T., Cai Y. C., Xu S. N., Chen Z. Z. (2017). Spatial distribution of zooplankton in continental slope of northern South China Sea in summer. South China Fish. Sci. 13 (5), 8–15. doi: 10.3969/j.issn.2095-0780.2017.05.002
Gu X. L., Xu Z. L. (2008). Ecological character of pelagic mysids in Yangtze estuary of China. Chin. J. Appl. Ecol. 19 (9), 2042–2048. doi: CNKI:SUN:YYSB.0.2008-09-029
Hooff R. C., Peterson W. T. (2006). Copepod biodiversity as an indicator of changes in ocean and climate conditions of the northern California Current ecosystem. Limnol. Oceanogr. 51, 2607–2620. doi: 10.4319/lo.2006.51.6.2607
Hu J., Xu Z. L., Zhu D. D. (2008). Seasonal changes of ecological characteristics of pelagic mollusc in Yangtze river estuary. J. Fish. Sci. China 15 (6), 111–123. doi: 10.3321/j.issn:1005-8737.2008.06.011
Jang M. C., Shin K., Hyun B., Lee T., Choi K. H. (2013). Temperature-regulated egg production rate, and seasonal and interannual variations in Paracalanus parvus. J. Plankton Res. 35 (5), 1035–1045. doi: 10.1093/plankt/fbt050
Jiang Z., Liu J., Chen J., Chen Q., Xuan J., Zeng J. (2014). Responses of summer phytoplankton community to drastic environmental changes in the Changjiang (Yangtze river) estuary during the past 50 years. Water Res. 54, 1–11. doi: 10.1016/j.watres.2014.01.032
Jiang T., Yu Z., Song X., Cao X., Yuan Y. (2010). Long-term ecological interactions between nutrient and phytoplankton community in the Changjiang estuary. Chin. J. Oceanol. Limnol. 28, 887–898. doi: 10.1007/s00343-010-9059-5
Kang B., Pecl G., Lin L., Sun P., Zhang P., Li Y., et al. (2021). Climate change impacts on China’s marine ecosystems. Rev. Fish. Biol. Fish. 31, 599–629. doi: 10.1007/s11160-021-09668-6
Keister J., Di Lorenzo E., Morgan C., Combes V., Peterson W. (2011). Zooplankton species composition is linked to ocean transport in the northern California Current. Glob. Change Biol. 17, 2498–2511. doi: 10.1111/j.1365-2486.2010.02383.x
Kimmel D., Boynton W., Roman M. (2012). Long-term decline in the calanoid copepod Acartia tonsa in central Chesapeake bay, USA: An indirect effect of eutrophication? Estuarine Coast. Shelf Sci. 101, 76–85. doi: 10.1016/j.ecss.2012.02.019
Lehman P., Teh S. J., Boyer G., Nobriga M., Bass E., Hogle C. (2010). Initial impacts of microcystis aeruginosa blooms on the aquatic food web in the San Francisco estuary. Hydrobiologia 637, 229–248. doi: 10.1007/s10750-009-9999-y
Li L., Cen J., Cui L., Lu S. (2019). Response of size-fractionated phytoplankton to environmental factors near the Changjiang estuary. Acta Oceanol. Sin. 38, 151–159. doi: 10.1007/s13131-018-1259-4
Li W., Ge J., Ding P., Ma J., Glibert P., Liu D. (2021). Effects of dual fronts on the spatial pattern of chlorophyll-a concentrations in and off the Changjiang river estuary. Estuaries Coasts 44 (5), 1408–1418. doi: 10.1007/s12237-020-00893-z
Li J. S., Li S. F., Ding F. Y. (2007). Analysis on annual change of fish diversity in Yangtze estuary offshore water area. J. Fish. Sci. China 14, 637–643.
Li Y., Li D., Tang J., Wang Y., Liu Z., He S. (2010). Long-term changes in the Changjiang estuary plankton community related to anthropogenic eutrophication. Aquat. Ecosyst. Health Manage. 13, 66–72. doi: 10.1080/14634980903579942
Li Z., Song S., Li C., Yu Z. (2018). The sinking of the phytoplankton community and its contribution to seasonal hypoxia in the Changjiang (Yangtze river) estuary and its adjacent waters. Estuarine Coast. Shelf Sci. 208, 170–179. doi: 10.1016/j.ecss.2018.05.007
Liu S. H., Xiang L. Y., Liu C. C., Wang J. H. (2013). Ecological distribution characteristics of zooplankton in Yangtze river estuary in spring and summer during 2007-2008. Mar. Sci. Bull. 32 (2), 184–190. doi: 10.11840/j.issn.1001-6392.2013.02.010
Luo B. Z., Shen H. T. (1994). Three gorges project and estuarine ecological environment (Beijing, China: Science Press), 212–214.
Ma Z., Xu Z., Zhou J. (2009). Effect of global warming on the distribution of Lucifer intermedius and L. hanseni (Decapoda) in the Changjiang estuary. Prog. Nat. Sci. 19, 1389–1395. doi: 10.1016/j.pnsc.2008.12.008
Piontkovski S., Castellani C. (2009). Long-term declining trend of zooplankton biomass in the tropical Atlantic. Hydrobiologia 632, 365–370. doi: 10.1007/s10750-009-9854-1
Purcell J. E. (2005). Climate effects on formation of jellyfish and ctenophore blooms: a review. J. Mar. Biol. Assoc. U. K. 85 (3), 461–476. doi: 10.1017/S0025315405011409
Purcell J. (2007). Environmental effects on asexual reproduction rates of the scyphozoan, Aurelia labiata. Mar. Ecol.: Prog. Ser. 348, 183–196. doi: 10.3354/meps07056
Purcell J. E. (2012). Jellyfish and ctenophore blooms coincide with human proliferations and environmental perturbations. Ann. Rev. Mar. Sci. 4 (1), 209–235. doi: 10.1146/annurev-marine-120709-142751
Purcell J. E., Uye S., Lo W. (2007). Anthropogenic causes of jellyfish blooms and their direct consequences for humans: a review. Mar. Ecol.: Prog. Ser. 350, 153–174. doi: 10.3354/meps07093
R Development Core Team (2014). R: A language and environment for statistical computing (Vienna, Austria: R Foundation for Statistical Computing). Available at: http://www.R-project.org/, ISBN: ISBN 3–900051–07–0.
Rebstock G. A., Kang Y. S. (2003). A comparison of three marine ecosystems surrounding the Korean peninsula: Responses to climate change. Prog. Oceanogr. 59, 357–379. doi: 10.1016/j.pocean.2003.10.002
Rice E. (2015). Impact of climate change on estuarine zooplankton: Surface water warming in long island sound is associated with changes in copepod size and community structure. Estuaries Coasts 38, 13–23. doi: 10.1007/s12237-014-9770-0
Salmi T., Määttä A., Anttila P., Ruoho-Airola T., Amnell T. (2002). Detecting trends of annual values of atmospheric pollutants by the Mann-Kendall test and sen’s slope estimates-the excel template application MAKESENS (Helsinki: Finnish Meteorological Institute).
Sheridan C. C., Landry M. R. (2004). A 9-year increasing trend in mesozooplankton biomass at the Hawaii ocean time-series station ALOHA. J. Mar. Sci. 61 (4), 457–463. doi: 10.1016/j.icesjms.2004.03.023
Shi Y., Song S., Zhang G. T., Wang S., Li C. (2015). Distribution pattern of zooplankton functional groups in the Yellow Sea in June: A possible cause for geographical separation of giant jellyfish species. Hydrobiologia 754, 43–58. doi: 10.1007/s10750-014-2070-7
Shi Y., Sun S., Li C., Zhang G. T. (2016). Interannual changes in the abundance of zooplankton functional groups in the southern Yellow Sea in early summer. Oceanolog. Limnolog. Sin. 47, 1–8. doi: 10.11693/hyhz20150300095
Stalder L., Marcus N. (1997). Zooplankton responses to hypoxia: Behavioral patterns and survival of three species of calanoid copepods. Mar. Biol. 127, 599–607. doi: 10.1007/s002270050050
Steinberg D., Landry M. (2017). Zooplankton and the ocean carbon cycle. Ann. Rev. Mar. Sci. 9, 413–444. doi: 10.1146/annurev-marine-010814-015924
Stone J., Steinberg D., Fabrizio M. (2018). Long-term changes in gelatinous zooplankton in Chesapeake bay, USA: Environmental controls and interspecific interactions. Estuaries Coasts 42 (2), 513–527. doi: 10.1007/s12237-018-0459-7
Sun S., Li Y., Sun X. (2012). Changes in the small-jellyfish community in recent decades in Jiaozhou bay, China. Chin. J. Oceanol. Limnol. 30 (4), 507–518. doi: 10.1007/s00343-012-1179-7
Suzuki K., Nakamura Y., Hiromi J. (1999). Feeding by the small calanoid copepod Paracalanus sp. on heterotrophic dinoflagellates and ciliates. Aquat. Microb. Ecol. 17 (1), 99–103. doi: 10.3354/ame017099
Wang B. (2006). Cultural eutrophication in the Changjiang (Yangtze river) plume: History and perspective. Estuarine Coast. Shelf Sci. 69, 471–477. doi: 10.1016/j.ecss.2006.05.010
Wang Y., Chen X. Q., Lin M., Xiang P., Wang C. G., Wang Y. G. (2014). Composition distribution and interannual variation of zooplankton communities in the northern South China Sea. in The 14th annual proceedings of Fujian Marine Society, Xiamen, China.
Wang B., Mming X., Wei Q., Xie L. (2018). A historical overview of coastal eutrophication in the China seas. Mar. pollut. Bull. 136, 394–400. doi: 10.1016/j.marpolbul.2018.09.044
Wang Y. T., Sun S., Li C. L., Zhang F. (2012). Effects of temperature and food on asexual reproduction of the scyphozoan, Aurelia sp.1. Oceanolog. Limnolog. Sin. 43 (5), 900–904. doi: 10.11693/hyhz201205004004
Wang L., Wang B. D., Chen Q. W., Tang X. W., Han R. (2016). Characteristics of the zooplankton community and impact factors in the Yangtze estuary coastal erea after third stage impoundment of the Three Gorges Dam. Acta Ecolog. Sin. 36 (9), 2505–2512. doi: 10.5846/stxb201412062423
Wang Y., Xu H., Li M. (2021). Long-term changes in phytoplankton communities in China’s Yangtze estuary driven by altered riverine fluxes and rising sea surface temperature. Geomorphology 376, 107566. doi: 10.1016/j.geomorph.2020.107566
Wang P., Zhang F., Liu M., Sun S., Xian H. (2020). Isotopic evidence for size-based dietary shifts in the jellyfish cyanea nozakii in the northern East China Sea. J. Plankton. Res. 42(6), 689–701. doi: 10.1093/plankt/fbaa042
Wang S., Zhang G.T., Zhou K., Song S. (2020b). Long-term population variability and reproductive strategy of a northward expanded ctenophore pleurobrachia globosa mose In a temperate bay, China. J. Exp. Mar. Biol. Ecol. 533, 151457. doi: 10.1016/j.jembe.2020.151457
Wiafe G., Yaqub H., Mensah M., Frid C. (2008). Impact of climate change on long-term zooplankton biomass in the upwelling region of the gulf of Guinea. J. Mar. Sci. 65, 318–324. doi: 10.1093/icesjms/fsn042
Xian W. W., Kang B., Liu R. Y. (2005). Jellyfish blooms in the Yangtze estuary. Science 307, 41. doi: 10.1126/science.307.5706.41c
Xian W. W., Luo B. Z. (2015). Estuarine ecology and environment before impoundment of three gorges project (Beijing, China: China Ocean Press), 176–177.
Xu Z. L., Gao Q. (2009). Labidocera euchaeta: its distribution in Yangtze river estuary and responses to global warming. Chin. J. Appl. Ecol. 20 (5), 1196–1201.
Xu Z. L., Gao Q., Kang W., Zhou J. (2013). Regional warming and decline in abundance of Euchaeta plana (Copepoda, calanoida) in the nearshore waters of the East China Sea. J. Crustacean Biol. 33, 323–331. doi: 10.2307/23446134
Xu R., Li Y. H., Li Z. E., Wang J. H. (2009). Quantitative comparison of zooplankton in different habitats of the Changjiang estuary. Acta Ecolog. Sin. 29 (4), 1688–1696. doi: 10.3321/j.issn
Xu Z. L., Shen X. Q. (2005). Zooplankton biomass and its variation in water near Changjiang estuary. Resourcis Environ. Yangtze basin 14 (3), 282–286. doi: 10.3969/j.issn.1004-8227.2005.03.004
Xu Z. L., Shen X. Q., Ma S. W. (2005). Ecological characters of zooplankton dominant species in the waters near the Changjiang estuary in spring and summer. Mar. Sci. 29 (12), 13–19. doi: 10.1360/biodiv.050121
Xu Z. L., Zhang D. (2014). Dramatic declines in Euphausia pacifica abundance in the East China Sea: Response to recent regional climate change. Zool. Sci. 31, 135–142. doi: 10.2108/zsj.31.135
Yang L., Liu J., Wang X. L., Xu Y., Li X., He L. (2018). Zooplankton community variation and its relationship with environmental variables in Bohai bay. J. Mar. Sci. 36 (1), 93–101.
Yang Y., Liu P. X., Zhou H. H., Xia L. H. (2020). Evaluation of the biodiversity variation and ecosystem health assessment in Changjiang estuary during the past 15 years. Acta Ecolog. Sin. 40 (24), 8892–8904. doi: 10.5846/stxb201912272811
Zhang W., Moriarty J., Wu H., Feng Y. (2021). Response of bottom hypoxia off the Changjiang river estuary to multiple factors: A numerical study. Ocean. Modelling. 159, 101751. doi: 10.1016/j.ocemod.2021.101751
Zhang G. T., Song S., Xu Z., Zhang Q. (2010). Unexpected dominance of the subtropical copepod temora turbinata in the temperate Changjiang river estuary and its possible causes. Zool. Stud. 49, 492–503. doi: 10.1111/j.1365-2141.1995.tb05244.x
Zhang D. J., Yan Q. L., Wang Z. L. (2008). Variation in species number and biomass of zooplankton in typical estuaries of China. Oceanolog. Et Limnolog. Sin. 39 (5), 536–540. doi: 10.3321/j.issn:0029-814X.2008.05.017
Zhao B., Yao P., Li D., Yu Z. (2021). Effects of river damming and delta erosion on organic carbon burial in the Changjiang estuary and adjacent East China Sea inner shelf. Sci. Total Environ. 793, 148610. doi: 10.1016/j.scitotenv.2021.148610
Zhou X. D., Xu Z. L. (2009). Ecological characteristics of the pelagic decapods in the Changjiang estuary. J. Fish. China 12 (2), 111–123. doi: 10.3321/j.issn:1000-0615.2009.01.005
Keywords: zooplankton, multidecadal trend, anthropogenic stressors, global warming, Changjiang estuary
Citation: Du P, Ye W-J, Deng B-P, Mao M, Zhu Y-L, Cheng F-P, Jiang Z-B, Shou L and Chen Q-Z (2022) Long-term changes in zooplankton in the Changjiang estuary from the 1960s to 2020. Front. Mar. Sci. 9:961591. doi: 10.3389/fmars.2022.961591
Received: 04 June 2022; Accepted: 28 June 2022;
Published: 15 August 2022.
Edited by:
Jun Xu, Institute of Hydrobiology, Chinese Academy of Sciences (CAS), ChinaReviewed by:
Kaizhi Li, South China Sea Institute of Oceanology, Chinese Academy of Sciences, ChinaWuchang Zhang, Institute of Oceanology, Chinese Academy of Sciences (CAS), China
Copyright © 2022 Du, Ye, Deng, Mao, Zhu, Cheng, Jiang, Shou and Chen. This is an open-access article distributed under the terms of the Creative Commons Attribution License (CC BY). The use, distribution or reproduction in other forums is permitted, provided the original author(s) and the copyright owner(s) are credited and that the original publication in this journal is cited, in accordance with accepted academic practice. No use, distribution or reproduction is permitted which does not comply with these terms.
*Correspondence: Quan-Zhen Chen, chenquanzhen@sio.org.cn