Azole Fungicides and Their Endocrine Disrupting Properties: Perspectives on Sex Hormone-Dependent Reproductive Development
- National Food Institute, Technical University of Denmark, Lyngby, Denmark
Azoles are antifungal agents used in both agriculture and medicine. They typically target the CYP51 enzyme in fungi and, by so doing, disrupt cell membrane integrity. However, azoles can also target various CYP enzymes in mammals, including humans, which can disrupt hormone synthesis and signaling. For instance, several azoles can inhibit enzymes of the steroidogenic pathway and disrupt steroid hormone biosynthesis. This is of particular concern during pregnancy, since sex hormones are integral to reproductive development. In other words, exposure to azole fungicides during fetal life can potentially lead to reproductive disease in the offspring. In addition, some azoles can act as androgen receptor antagonists, which can further add to the disrupting potential following exposure. When used as pharmaceuticals, systemic concentrations of the azole compounds can become significant as combatting fungal infections can be very challenging and require prolonged exposure to high doses. Although most medicinal azoles are tightly regulated and used as prescription drugs after consultations with medical professionals, some are sold as over-the-counter drugs. In this review, we discuss various azole fungicides known to disrupt steroid sex hormone biosynthesis or action with a focus on what potential consequences exposure during pregnancy can have on the life-long reproductive health of the offspring.
Introduction
Over-the-counter (OTC) drugs are widely accessible. The global pharmaceutical OTC drugs market is estimated to be around US $130 billion per annum and steadily increasing. These drugs are deemed safe for the consumer as long as they are used appropriately. However, used inappropriately even OTC drugs pose potential health risks and should be used with caution. Worryingly, a high proportion of consumers consider OTC drugs completely harmless, thereby running the risk of adverse health effects by underestimating the risks associated with self-medication (Roumie and Griffin, 2004; Westerlund et al., 2017; Ylä-Rautio et al., 2020; Bauer et al., 2021). For instance, a Swedish survey from 2017 reported that 7% of participants agreed completely or to a large extent on the statement that OTC drugs are “completely harmless regardless of how they are being used” and 54% that they are “harmless as long as you follow the directions on the package” (Westerlund et al., 2017).
OTC drugs comprise a diverse group of pharmaceuticals counting more than 300,000 registered products according to the United States Food and Drug Administration (U.S. FDA, 2020). They have widely different applications and effects, also with varied drug-related problems (Ylä-Rautio et al., 2020). Amongst these effects are endocrine disrupting properties and consequences for reproductive development and function. In particular mild analgesics have received much focus in recent years because of suspected endocrine disrupting properties and associated reproductive disorders (Kristensen et al., 2016; Bauer et al., 2021). A major concern with such endocrine disrupting OTC drugs is inappropriate use by pregnant women, as fetal sexual development is reliant on sex hormones. If these hormones are disrupted during early life, especially in male fetuses, it can lead to a range of reproductive disorders in humans including genital malformations, germ cell cancers, and infertility (Skakkebaek et al., 2016; Jorgensen et al., 2021).
Besides analgesics, OTC antifungal drugs containing azoles are widely used, also by pregnant women who frequently develop vaginal yeast infections (Sobel, 2007). Many azole fungicides are potent endocrine disruptors, but these also include both prescription drugs and agricultural pesticides. With regard to OTC drugs containing azoles as the active ingredient, they are considered safe for the user; however, recent studies have questioned this, at least for certain vulnerable individuals such as the unborn child (Mogensen et al., 2017; Draskau et al., 2021). Herein, we will briefly discuss the endocrine disrupting potential of azoles and possible detrimental effects on sexual development.
Azole Fungicides
The first azole with antifungal activity was reported in the 1940s and by the late 1950s antifungal drugs with azoles as the active ingredient was introduced to the market for clinical use (Sheehan et al., 1999). Today, more than 60 years later, the number of azole compounds has increased and they are used extensively to combat yeast infections in a clinical setting; often a first-choice treatment for mycotic infections including vaginal mycosis in pregnant women (Sobel, 2007). Notably, azoles are also widely used for agricultural crop protection. These agricultural azoles are not a focus of this review, but rather mentioned for two important reasons. Firstly, because the broad use in both agriculture and pharmacology leads to emerging fungal resistance to azoles (Bastos et al., 2021). Secondly, because the endocrine disrupting modes of action often are shared between many agricultural and medicinal azoles, thus studies across domains can inform on their potential to cause endocrine disrupting effects.
Azole antifungal agents, being it agricultural or medicinal, are classically designed to interfere with the heme-group of the fungal cytochrome P450 (CYP) enzyme CYP51, also known as sterol 14α-demethylase (Podust et al., 2001; De Petris et al., 2015). In this way, azoles block the production of the essential fungal membrane component ergosterol, disrupting the cell membrane and ultimately inhibiting fungal growth (Hitchcock et al., 1990; Georgopapadakou, 1998). A challenge, however, is that CYP enzymes are found in all animal kingdoms. For instance, humans and other animals also possess a CYP51 enzyme that is structurally closely related to fungal CYP51. But perhaps more problematically, many azoles can also interfere with other members of the CYP superfamily of enzymes, of which there are many.
With respect to reproductive development, which is the primary focus herein, the CYP enzymes of the steroidogenic pathway are obvious and include important regulators of sex hormone synthesis such as CYP17 and CYP19. In fact, CYP19 (aromatase) has been shown to be a key target of many azole fungicides, both agricultural and medicinal (Mason et al., 1985; Vinggaard et al., 2000; Kragie et al., 2002; Zarn et al., 2003; Trösken et al., 2006). In humans and other mammals, CYP19 is responsible for converting androgens to estrogens and as such play a critical role in sex hormone homeostasis throughout development and in adulthood (Simpson et al., 2002; Blakemore and Naftolin, 2016). Hence, perturbing CYP19 activity can impede on sexual development and function, as discussed further below.
Hormone-Dependent Male Sexual Development
A detailed description of male sexual development is beyond the scope of this article, yet we will outline some key points that are essential with respect to the potential adverse effects azoles can cause if exposure occurs during fetal life. In mammals, sex is initially determined by the pairing of sex chromosomes at fertilization, which is followed by gonadal sex determination approximately 6 weeks after conception in humans (Jorgensen et al., 2021), resulting in testes forming in XY fetuses and ovaries in XX fetuses. Following gonadal sex determination, the testes of male fetuses will rapidly differentiate and start to produce testosterone by the fetal Leydig cells (Svingen and Koopman, 2013). In turn, testosterone will be secreted by the testes into the circulation and, in simple terms, drive masculinization of the male fetus. In rats, a masculinization programming window (MPW) has been established (Welsh et al., 2008), which corresponds to a window of fetal development where most of the masculine phenotypes are determined in response to a surge in testosterone synthesis; a sensitive window also found in other mammals, including humans (Sharpe, 2020). Importantly, however, differentiation of external genitalia and several other androgen-sensitive tissues relies on the presence of dihydrotestosterone (DHT) rather than testosterone.
DHT is synthesized from testosterone by the enzyme 5α-reductase in target tissues. In mice and rats, this relationship appears relatively linear, with testosterone mainly deriving from the testicular Leydig cells. However, in several other mammals, including humans, DHT can also be synthesized from the intermediate steroid hormone androsterone during development and this intermediate steroid is largely derived from the placenta, and to a lesser extent the fetal liver and adrenals (O’Shaughnessy et al., 2019). This alternative ‘backdoor pathway’ to androgen signaling during development is significantly different between species such as rats and humans, and must be considered when evaluating how perturbation to androgen synthesis or signaling can result in incomplete masculinization (Sharpe, 2020).
Nevertheless, exposure to chemical substances with anti-androgenic activities during the MPW can impede on masculinization processes and lead to reproductive disorders. They include hypospadias or cryptorchidism at birth, or reduced fertility or testis cancers later in life (Skakkebaek et al., 2016; Jorgensen et al., 2021). Impaired masculinization can also be assessed by the anogenital distance (AGD), which is considered a broad biomarker of fetal androgen action; although not a disorder in itself, a short AGD in male offspring is associated with male reproductive disorders (Thankamony et al., 2016; Schwartz et al., 2019; Fischer et al., 2020). As will be discussed in the following, exposure to certain azoles can lead to these adverse effect outcomes.
Adverse Reproductive Effects of Azole Fungicides
Prenatal exposure to antifungal azole drugs has been associated with short male AGD in humans (Mogensen et al., 2017). This preliminary study reported that exposure to a single oral dose of 150 mg fluconazole inside gestational weeks 8–14 of pregnancy (assumed to be the human MPW) was associated with a significantly shorter AGD in newborn boys. Interestingly, use of OTC vaginal tablets containing miconazole or clotrimazole was also associated with a nominally shorter AGD, albeit not statistically significant, and shortest in boys that had been exposed during the MPW compared to outside this developmental window (Mogensen et al., 2017).
It should be noted that the aforementioned study, as well as other relevant epidemiological studies of azole fungicides and potential reproductive effects in humans, has relied on a small number of cases. As such, our knowledge is still limited when it comes to associations between developmental azole exposure and adverse reproductive outcomes in humans, including hypospadias or cryptorchidism at birth, or reduced fertility or testis cancers later in life. But several epidemiological studies, again with small case numbers, have found no overall increased risk of hypospadias or cryptorchidism following azole treatment during pregnancy (Mastroiacovo et al., 1996; Czeizel et al., 2004; Carter et al., 2008; Nørgaard et al., 2008; Howley et al., 2016; Liu et al., 2020). Based on these seemingly disparate studies of low statistical power, larger prospective studies investigating the potential adverse reproductive effects of azole medications are needed to be able to form any solid conclusions.
Numerous studies using rats or mice have investigated the endocrine disrupting potentials of azole fungicides from both agriculture and medicine, and the observed adverse reproductive outcomes are many and diverse (Figure 1). Several azoles induce anti-androgenic effects such as short male AGD following developmental exposure (Vinggaard et al., 2005; Laier et al., 2006; Taxvig et al., 2007; Taxvig et al., 2008; Hass et al., 2012; Johansson et al., 2021a), but surprisingly also longer AGDs in both male and female offspring with certain azole fungicides (Noriega et al., 2005; Laier et al., 2006; Goetz et al., 2007; Taxvig et al., 2007; Christiansen et al., 2009; Hass et al., 2012; Melching-Kollmuss et al., 2017). Developmental exposure to azoles have also been shown to cause nipple retention in male rat offspring (Noriega et al., 2005; Vinggaard et al., 2005; Laier et al., 2006; Taxvig et al., 2007; Hass et al., 2012), which is a biomarker for compromised androgen action (Schwartz et al., 2021). Azoles can also cause increased incidents of malformed external genitalia and hypospadias (Noriega et al., 2005; Laier et al., 2006), as well as delayed pubertal onset (Melching-Kollmuss et al., 2017; Johansson et al., 2021a), and fewer motile sperm (Taxvig et al., 2007; Melching-Kollmuss et al., 2017).
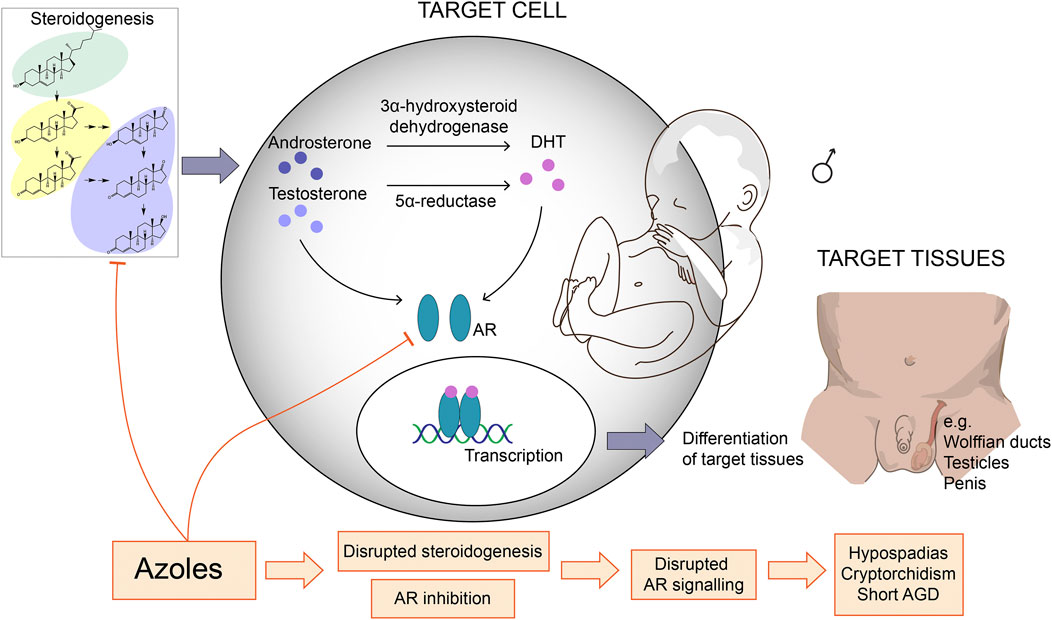
FIGURE 1. Azole fungicides with anti-androgenic effects and their potential mechanisms of action. Many azole fungicides can inhibit CYP enzyme activity and thereby disrupt steroidogenesis and, ultimately, steroid hormone profiles. This may lead to reduced androgen synthesis and signaling in androgen-sensitive target cells. Certain azoles can also antagonize the androgen receptor (AR) thereby interfere with AR activation and gene regulation in target cells. Since androgen signaling is crucial for masculinization during development, these molecular effects can impede on male development leading to reproductive disorders. AGD = anogenital distance, DHT = dihydrotestosterone.
Another frequently observed effect in rats is changes to steroid hormone levels in both serum and reproductive organs, specifically the testes. Common observations include decreased testosterone levels and increased progesterone (Vinggaard et al., 2005; Vinggaard et al., 2006; Laier et al., 2006; Taxvig et al., 2007; Taxvig et al., 2008; Draskau et al., 2021), but also decreased estrogen in plasma (Taxvig et al., 2008; Draskau et al., 2019; Draskau et al., 2021). These in vivo effects fit with what we know about the affinity towards CYP enzymes for many azoles, as revealed by numerous in vitro studies (Loose et al., 1983; Mason et al., 1985; Mason et al., 1987; Ayub and Levell, 1989a; Kragie et al., 2002; Zarn et al., 2003; Trösken et al., 2006; Kjærstad et al., 2010; Ohlsson et al., 2010; Van der Pas et al., 2012; Gal and Orly, 2014; Munkboel et al., 2019). This also extends to human fetal testis explant studies with ketoconazole, imazalil, prochloraz, and propiconazole, where exposure significantly lowered testosterone production (up to 90%) compared to vehicle controls (Mazaud-Guittot et al., 2013; Gaudriault et al., 2017). As for elevated progesterone, this is of potential importance for two reasons. Firstly, progesterone does not only act as a ligand for the progesterone receptor (PR), but has also been shown to have off-target effects on other nuclear receptors and give rise to various effects in vivo, including masculinization effects in female offspring arising from exposure to the progestin medroxyprogesterone (Wilkins et al., 1958; Money and Mathews, 1982; Kuhl, 2005). Secondly, because elevated progesterone may also lead to elevated androgen levels (Auchus and Chang, 2010), which help explain masculinization effects in female rat offspring exposed to prochloraz (Laier et al., 2006; Melching-Kollmuss et al., 2017). Finally, disrupted progesterone levels is also of importance in that it is intimately involved in regulating parturition. In fact, many azoles are known to disrupt parturition or induce dystocia in rats, likely by maintained high progesterone levels when a significant drop is required to induce parturition (Wolf et al., 1999; Noriega et al., 2005; Taxvig et al., 2007; Melching-Kollmuss et al., 2017; Johansson et al., 2021a).
Certain azoles are potent AR antagonists in vitro (Kjærstad et al., 2010; Roelofs et al., 2014; Draskau et al., 2019). Many of these are agricultural azoles, but they also include some pharmaceuticals, albeit they appear to be less potent AR antagonists. For instance, itraconazole (Williams et al., 2017; U.S. Environmental Protection Agency, 2021) and ketoconazole (Eil, 1992) (with some opposing evidence (Ayub and Levell, 1989b; Kjærstad et al., 2010)) seem able to interfere with the AR, whereas clotrimazole, miconazole, and fluconazole do not (Ayub and Levell, 1989b; Eil, 1992; Kjærstad et al., 2010; Roelofs et al., 2014). This is important since, as discussed above, fetal male reproductive development in humans is less dependent on testosterone as DHT can be synthesized from the intermediate androsterone coming from the placenta, adrenal, or liver. Notably, however, steroidogenesis disruptors can also be envisioned to perturb synthesis in other tissues and organs, as well as other biosynthesis pathways, including the backdoor pathway. Nevertheless, humans may not be as susceptible to disruption by chemicals perturbing testosterone synthesis by the testes as mice and rats are. The AR, on the other hand, is downstream of DHT action and consequently chemicals blocking DHT-dependent activation of the AR can have detrimental effects in the developing fetus irrespective of androgen synthesis. Thus, despite significant differences in androgen synthesis between mice/rats and humans, potential direct effects on nuclear receptors such as the AR raise further concern about the human health-related effects of exposure to azole fungicides.
In vitro and in vivo studies clearly demonstrate the endocrine disrupting potential of azole fungicides. Then, despite the fact that many azole fungicides are not readily absorbed (for instance from vaginal applicators) and are often rapidly metabolized, concerns still remain regarding indiscriminate use of azole fungicides, especially during pregnancy. For instance, an OTC azole fungicide such as clotrimazole has shown strong endocrine disrupting effects in vitro and in vivo at very low concentrations, even below human therapeutically used concentrations (Munkboel et al., 2019; Draskau et al., 2021). A second example, fluconazole, also displays endocrine disrupting potential (Mogensen et al., 2017; Munkboel et al., 2019), almost completely avoids metabolization (Bury et al., 2021), and can rapidly penetrate from plasma into female genital organs including uterus, ovary, and oviduct (Mikamo et al., 1999). These examples are cause for some concern regarding azole use during pregnancy and concomitant exposure of the unborn child.
Non-Classical Mechanisms of Azole Fungicides
When assessing the endocrine disrupting potential of chemicals it is common to primarily consider the classic estrogenic, androgenic, thyroid, and steroidogenic modalities. This does not exclude that other modalities could be relevant for endocrine disruption, however, and many other signaling pathways have been highlighted as important to consider in this context (Martyniuk et al., 2022). Our knowledge remains limited when considering the extent to which other mechanisms relevant for endocrine disruption can cause adverse health effects, but with respect to azole fungicides several alternative signaling pathways may be relevant.
Evidence exists that many azoles are capable of disrupting important evolutionary conserved pathways. For instance, the antifungal drug itraconazole can interfere with Hedgehog (HH) signaling (Tiboni et al., 2006; Johansson et al., 2021b), whereas ketoconazole can, for instance, affect sex-specific retinoic acid (RA) signaling in developing mouse testes (Bowles et al., 2006; Koubova et al., 2006). However, interference with these important morphogenic pathways also raises the question of teratogenicity.
Potent HH and RA disruptors, including specific azoles, are known to induce malformations and severe developmental toxicity in both animal models (Menegola et al., 2006; Tiboni et al., 2006, Tiboni et al., 2008; Dimopoulou et al., 2017) and humans (Mastroiacovo et al., 1996; Kazy et al., 2005; Carter et al., 2008; Nørgaard et al., 2008; Giavini and Menegola, 2010; Mølgaard-Nielsen et al., 2013; Howley et al., 2016). As such, chemicals acting by these modes of action would likely cause teratogenic effects at doses below or equal to those that would disrupt reproductive development. Still, considering the fact that human exposure often consists of a larger mixture of chemicals each at low concentrations, likely below concentrations that will induce teratogenicity, it can be speculated that several azoles or other chemicals interfering with these signaling pathways simultaneously can actually disrupt reproductive development. On the other hand, the importance of these modalities for medicinal drugs, which are often used at high concentrations, in inducing reproductive disorders may thus be to a lesser extent.
Concluding Remarks
Azoles are important agents used to combat fungal infections. Their importance for these applications should not be underestimated, not least considering that fungal infections can be very difficult to control or overcome. This short review is not intended to question the value of azole-containing drugs in treating these conditions. Rather, it is intended to highlight the fact that azole compounds can be very potent endocrine disruptors and act on several different signaling pathways that are crucial for normal development, herein with focus on reproductive development. This review has also emphasized a potential concern about OTC azole fungicides in particular, since indiscriminate use during pregnancy could impact reproductive development; perhaps not in isolation, but could lead to problematic outcomes if used in combination with other endocrine disrupting compounds such as analgesics and general exposure to environmental chemicals. Perhaps we would be better served by applying a cautionary principle.
Author Contributions
MKD and TS conceptualized the review. MKD made literature searches. MKD and TS wrote the first draft of the manuscript. MKD designed figure. MKD and TS prepared final figure. MKD and TS contributed to manuscript revision, read, and approved the submitted version.
Funding
This work was funded by the Danish Environmental Protection Agency (EPA) as a project under the Centre on Endocrine Disruptors (CeHoS). The funding body (Danish EPA) had no involvement in the writing, defining of the content, or in the decision to submit the article for publication.
Conflict of Interest
The authors declare that the research was conducted in the absence of any commercial or financial relationships that could be construed as a potential conflict of interest.
Publisher’s Note
All claims expressed in this article are solely those of the authors and do not necessarily represent those of their affiliated organizations, or those of the publisher, the editors and the reviewers. Any product that may be evaluated in this article, or claim that may be made by its manufacturer, is not guaranteed or endorsed by the publisher.
References
Auchus, R. J., and Chang, A. Y. (2010). 46,XX DSD: the Masculinised Female. Best Pract. Res. Clin. Endocrinol. Metab. 24, 219–242. doi:10.1016/J.BEEM.2009.11.001
Ayub, M., and Levell, M. J. (1989a). Inhibition of Human Adrenal Steroidogenic Enzymes In Vitro by Imidazole Drugs Including Ketoconazole. J. Steroid Biochem. 32, 515–524. doi:10.1016/0022-4731(89)90384-1
Ayub, M., and Levell, M. J. (1989b). The Effect of Ketoconazole Related Imidazole Drugs and Antiandrogens on [3H] R1881 Binding to the Prostatic Androgen Receptor and [3H]5α-Dihydrotestosterone and [3H]cortisol Binding to Plasma Proteins. J. Steroid Biochem. 33, 251–255. doi:10.1016/0022-4731(89)90301-4
Bastos, R. W., Rossato, L., Goldman, G. H., and Santos, D. A. (2021). Fungicide Effects on Human Fungal Pathogens: Cross-Resistance to Medical Drugs and beyond. Plos Pathog. 17, e1010073. doi:10.1371/journal.ppat.1010073
Bauer, A. Z., Swan, S. H., Kriebel, D., Liew, Z., Taylor, H. S., Bornehag, C. G., et al. (2021). Paracetamol Use during Pregnancy - a Call for Precautionary Action. Nat. Rev. Endocrinol. 17, 757–766. doi:10.1038/S41574-021-00553-7
Blakemore, J., and Naftolin, F. (2016). Aromatase: Contributions to Physiology and Disease in Women and Men. Physiology 31, 258–269. doi:10.1152/physiol.00054.2015
Bowles, J., Knight, D., Smith, C., Wilhelm, D., Richman, J., Mamiya, S., et al. (2006). Retinoid Signaling Determines Germ Cell Fate in Mice. Science 312, 596–600. doi:10.1126/science.1125691
Bury, D., Tissing, W. J. E., Muilwijk, E. W., Wolfs, T. F. W., and Brüggemann, R. J. (2021). Clinical Pharmacokinetics of Triazoles in Pediatric Patients. Clin. Pharmacokinet. 60, 1103–1147. doi:10.1007/S40262-021-00994-3
Carter, T. C., Druschel, C. M., Romitti, P. A., Bell, E. M., Werler, M. M., and Mitchell, A. A. (2008). Antifungal Drugs and the Risk of Selected Birth Defects. Am. J. Obstet. Gynecol. 198, 191e1–191e7. doi:10.1016/j.ajog.2007.08.044
Christiansen, S., Scholze, M., Dalgaard, M., Vinggaard, A. M., Axelstad, M., Kortenkamp, A., et al. (2009). Synergistic Disruption of External Male Sex Organ Development by a Mixture of Four Antiandrogens. Environ. Health Perspect. 117, 1839–1846. doi:10.1289/ehp.0900689
Czeizel, A. E., Kazy, Z., and Puho, E. (2004). Population-based Case-Control Teratologic Study of Topical Miconazole. Congenit. Anom. 44, 41–45. doi:10.1111/J.1741-4520.2004.00007.x
De Petris, A., Crestoni, M. E., Pirolli, A., Rovira, C., Iglesias-Fernández, J., Chiavarino, B., et al. (2015). Binding of Azole Drugs to Heme: A Combined MS/MS and Computational Approach. Polyhedron 90, 245–251. doi:10.1016/j.poly.2015.02.011
Dimopoulou, M., Verhoef, A., Pennings, J. L. A., van Ravenzwaay, B., Rietjens, I. M. C. M., and Piersma, A. H. (2017). Embryotoxic and Pharmacologic Potency Ranking of Six Azoles in the Rat Whole Embryo Culture by Morphological and Transcriptomic Analysis. Toxicol. Appl. Pharmacol. 322, 15–26. doi:10.1016/j.taap.2017.03.001
Draskau, M. K., Boberg, J., Taxvig, C., Pedersen, M., Frandsen, H. L., Christiansen, S., et al. (2019). In Vitro and In Vivo Endocrine Disrupting Effects of the Azole Fungicides Triticonazole and Flusilazole. Environ. Pollut. 255, 113309. doi:10.1016/j.envpol.2019.113309
Draskau, M. K., Rosenmai, A. K., Scholze, M., Pedersen, M., Boberg, J., Christiansen, S., et al. (2021). Human-relevant Concentrations of the Antifungal Drug Clotrimazole Disrupt Maternal and Fetal Steroid Hormone Profiles in Rats. Toxicol. Appl. Pharmacol. 422, 115554. doi:10.1016/j.taap.2021.115554
Eil, C. (1992). Ketoconazole Binds to the Human Androgen Receptor. Horm. Metab. Res. 24, 367–370. doi:10.1055/S-2007-1003337
Fischer, M. B., Ljubicic, M. L., Hagen, C. P., Thankamony, A., Ong, K., Hughes, I., et al. (2020). Anogenital Distance in Healthy Infants: Method-, Age- and Sex-Related Reference Ranges. J. Clin. Endocrinol. Metab. 105, 2996–3004. doi:10.1210/clinem/dgaa393
Gal, M., and Orly, J. (2014). Selective Inhibition of Steroidogenic Enzymes by Ketoconazole in Rat Ovary Cells. Clin. Med. Insights: Reprod. Health 8, CMRH.S14036–22. doi:10.4137/cmrh.s14036
Gaudriault, P., Mazaud-Guittot, S., Lavoué, V., Coiffec, I., Lesné, L., Dejucq-Rainsford, N., et al. (2017). Endocrine Disruption in Human Fetal Testis Explants by Individual and Combined Exposures to Selected Pharmaceuticals, Pesticides, and Environmental Pollutants. Environ. Health Perspect. 125, 087004. doi:10.1289/EHP1014
Georgopapadakou, N. H. (1998). Antifungals: Mechanism of Action and Resistance, Established and Novel Drugs. Curr. Opin. Microbiol. 1, 547–557. doi:10.1016/S1369-5274(98)80087-8
Giavini, E., and Menegola, E. (2010). Are Azole Fungicides a Teratogenic Risk for Human Conceptus? Toxicol. Lett. 198, 106–111. doi:10.1016/j.toxlet.2010.07.005
Goetz, A. K., Ren, H., Schmid, J. E., Blystone, C. R., Thillainadarajah, I., Best, D. S., et al. (2007). Disruption of Testosterone Homeostasis as a Mode of Action for the Reproductive Toxicity of Triazole Fungicides in the Male Rat. Toxicol. Sci. 95, 227–239. doi:10.1093/toxsci/kfl124
Hass, U., Boberg, J., Christiansen, S., Jacobsen, P. R., Vinggaard, A. M., Taxvig, C., et al. (2012). Adverse Effects on Sexual Development in Rat Offspring after Low Dose Exposure to a Mixture of Endocrine Disrupting Pesticides. Reprod. Toxicol. 34, 261–274. doi:10.1016/j.reprotox.2012.05.090
Hitchcock, C. A., Dickinson, K., Brown, S. B., Evans, E. G. V., and Adams, D. J. (1990). Interaction of Azole Antifungal Antibiotics with Cytochrome P-450-dependent 14α-Sterol Demethylase Purified from Candida Albicans. Biochem. J. 266, 475–480. doi:10.1042/bj2660475
Howley, M. M., Carter, T. C., Browne, M. L., Romitti, P. A., Cunniff, C. M., and Druschel, C. M. (2016). Fluconazole Use and Birth Defects in the National Birth Defects Prevention Study. Am. J. Obstet. Gynecol. 214, e1–657. e1–9. doi:10.1016/j.ajog.2015.11.022
Johansson, H. K. L., Christiansen, S., Draskau, M. K., Svingen, T., and Boberg, J. (2021a). Classical Toxicity Endpoints in Female Rats Are Insensitive to the Human Endocrine Disruptors Diethylstilbestrol and Ketoconazole. Reprod. Toxicol. 101, 9–17. doi:10.1016/j.reprotox.2021.01.003
Johansson, H. K. L., Taxvig, C., Olsen, G. P. M., and Svingen, T. (2021b). Effects of the Hedgehog Signaling Inhibitor Itraconazole on Developing Rat Ovaries. Toxicol. Sci. 182, 60–69. doi:10.1093/toxsci/kfab048
Jorgensen, A., Svingen, T., Miles, H., Chetty, T., Stukenborg, J.-B., and Mitchell, R. T. (2021). Environmental Impacts on Male Reproductive Development: Lessons from Experimental Models. Horm. Res. Paediatr., 1–17. doi:10.1159/000519964
Kazy, Z., Puhó, E., and Czeizel, A. E. (2005). Teratogenic Potential of Vaginal Metronidazole Treatment during Pregnancy. Eur. J. Obstet. Gynecol. Reprod. Biol. 123, 174–178. doi:10.1016/j.ejogrb.2005.03.016
Kjærstad, M. B., Taxvig, C., Nellemann, C., Vinggaard, A. M., and Andersen, H. R. (2010). Endocrine Disrupting Effects In Vitro of Conazole Antifungals Used as Pesticides and Pharmaceuticals. Reprod. Toxicol. 30, 573–582. doi:10.1016/j.reprotox.2010.07.009
Koubova, J., Menke, D. B., Zhou, Q., Capel, B., Griswold, M. D., and Page, D. C. (2006). Retinoic Acid Regulates Sex-specific Timing of Meiotic Initiation in Mice. Proc. Natl. Acad. Sci. U.S.A. 103, 2474–2479. doi:10.1073/pnas.0510813103
Kragie, L., Turner, S. D., Patten, C. J., Crespi, C. L., and Stresser, D. M. (2002). Assessing Pregnancy Risks of Azole Antifungals Using a High Throughput Aromatase Inhibition Assay. Endocr. Res. 28, 129–140. doi:10.1081/erc-120015045
Kristensen, D. M., Mazaud-Guittot, S., Gaudriault, P., Lesné, L., Serrano, T., Main, K. M., et al. (2016). Analgesic Use - Prevalence, Biomonitoring and Endocrine and Reproductive Effects. Nat. Rev. Endocrinol. 12, 381–393. doi:10.1038/nrendo.2016.55
Kuhl, H. (2005). Pharmacology of Estrogens and Progestogens: Influence of Different Routes of Administration. Climacteric 8, 3–63. doi:10.1080/13697130500148875
Laier, P., Metzdorff, S. B., Borch, J., Hagen, M. L., Hass, U., Christiansen, S., et al. (2006). Mechanisms of Action Underlying the Antiandrogenic Effects of the Fungicide Prochloraz. Toxicol. Appl. Pharmacol. 213, 160–171. doi:10.1016/j.taap.2005.10.013
Liu, D., Zhang, C., Wu, L., Zhang, L., and Zhang, L. (2020). Fetal Outcomes after Maternal Exposure to Oral Antifungal Agents during Pregnancy: A Systematic Review and Meta‐analysis. Int. J. Gynecol. Obstet. 148, 6–13. doi:10.1002/ijgo.12993
Loose, D. S., Kan, P. B., Hirst, M. A., Marcus, R. A., and Feldman, D. (1983). Ketoconazole Blocks Adrenal Steroidogenesis by Inhibiting Cytochrome P450-dependent Enzymes. J. Clin. Invest. 71, 1495–1499. doi:10.1172/jci110903
Martyniuk, C. J., Martínez, R., Navarro-Martín, L., Kamstra, J. H., Schwendt, A., Reynaud, S., et al. (2022). Emerging Concepts and Opportunities for Endocrine Disruptor Screening of the Non-EATS Modalities. Environ. Res. 204, 111904. doi:10.1016/j.envres.2021.111904
Mason, J. I., Murry, B. A., Olcott, M., and Sheets, J. J. (1985). Imidazole Antimycotics: Inhibitors of Steroid Aromatase. Biochem. Pharmacol. 34, 1087–1092. doi:10.1016/0006-2952(85)90613-6
Mason, J. I., Carr, B. R., and Murry, B. A. (1987). Imidazole Antimycotics: Selective Inhibitors of Steroid Aromatization and Progesterone Hydroxylation. Steroids 50, 179–189. doi:10.1016/0039-128X(83)90070-3
Mastroiacovo, P., Mazzone, T., Botto, L. D., Serafini, M. A., Finardi, A., Caramelli, L., et al. (1996). Prospective Assessment of Pregnancy Outcomes after First-Trimester Exposure to Fluconazole. Am. J. Obstet. Gynecol. 175, 1645–1650. doi:10.1016/s0002-9378(96)70119-9
Mazaud-Guittot, S., Nicolaz, C. N., Desdoits-Lethimonier, C., Coiffec, I., Maamar, M. B., Balaguer, P., et al. (2013). Paracetamol, Aspirin, and Indomethacin Induce Endocrine Disturbances in the Human Fetal Testis Capable of Interfering with Testicular Descent. J. Clin. Endocrinol. Metab. 98, E1757–E1767. doi:10.1210/jc.2013-2531
Melching-Kollmuss, S., Fussell, K. C., Schneider, S., Buesen, R., Groeters, S., Strauss, V., et al. (2017). Comparing Effect Levels of Regulatory Studies with Endpoints Derived in Targeted Anti-androgenic Studies: Example Prochloraz. Arch. Toxicol. 91, 143–162. doi:10.1007/s00204-016-1678-y
Menegola, E., Broccia, M. L., Di Renzo, F., and Giavini, E. (2006). Postulated Pathogenic Pathway in Triazole Fungicide Induced Dysmorphogenic Effects. Reprod. Toxicol. 22, 186–195. doi:10.1016/j.reprotox.2006.04.008
Mikamo, H., Kawazoe, K., Sato, Y., Izumi, K., Ito, T., Ito, K., et al. (1999). Penetration of Oral Fluconazole into Gynecological Tissues. Antimicrob. Agents Chemother. 43, 148–151. doi:10.1128/aac.43.1.148
Mogensen, D. M., Pihl, M. B., Skakkebæk, N. E., Andersen, H. R., Juul, A., Kyhl, H. B., et al. (2017). Prenatal Exposure to Antifungal Medication May Change Anogenital Distance in Male Offspring: a Preliminary Study. Environ. Health 16, 68. doi:10.1186/s12940-017-0263-z
Mølgaard-Nielsen, D., Pasternak, B., Hviid, A., and Sci, M. (2013). Use of Oral Fluconazole during Pregnancy and the Risk of Birth Defects. N. Engl. J. Med. 369, 830–839. doi:10.1056/NEJMoa1301066
Money, J., and Mathews, D. (1982). Prenatal Exposure to Virilizing Progestins: An Adult Follow-Up Study of Twelve Women. Arch. Sex. Behav. 11, 73–83. doi:10.1007/BF01541367
Munkboel, C. H., Rasmussen, T. B., Elgaard, C., Olesen, M.-L. K., Kretschmann, A. C., and Styrishave, B. (2019). The Classic Azole Antifungal Drugs Are Highly Potent Endocrine Disruptors In Vitro Inhibiting Steroidogenic CYP Enzymes at Concentrations Lower Than Therapeutic Cmax. Toxicology 425, 152247. doi:10.1016/j.tox.2019.152247
Nørgaard, M., Pedersen, L., Gislum, M., Erichsen, R., Sogaard, K. K., Schonheyder, H. C., et al. (2008). Maternal Use of Fluconazole and Risk of Congenital Malformations: a Danish Population-Based Cohort Study. J. Antimicrob. Chemother. 62, 172–176. doi:10.1093/jac/dkn157
Noriega, N. C., Ostby, J., Lambright, C., Wilson, V. S., and Gray, L. E. (2005). Late Gestational Exposure to the Fungicide Prochloraz Delays the Onset of Parturition and Causes Reproductive Malformations in Male but Not Female Rat Offspring1. Biol. Reprod. 72, 1324–1335. doi:10.1095/biolreprod.104.031385
Ohlsson, Å., Cedergreen, N., Oskarsson, A., and Ullerås, E. (2010). Mixture Effects of Imidazole Fungicides on Cortisol and Aldosterone Secretion in Human Adrenocortical H295R Cells. Toxicology 275, 21–28. doi:10.1016/j.tox.2010.05.013
O’Shaughnessy, P. J., Antignac, J. P., Le Bizec, B., Morvan, M.-L., Svechnikov, K., Söder, O., et al. (2019). Alternative (Backdoor) Androgen Production and Masculinization in the Human Fetus. Plos Biol. 17, e3000002. doi:10.1371/journal.pbio.3000002
Podust, L. M., Poulos, T. L., and Waterman, M. R. (2001). Crystal Structure of Cytochrome P450 14α-Sterol Demethylase (CYP51) from Mycobacterium tuberculosis in Complex with Azole Inhibitors. Proc. Natl. Acad. Sci. U.S.A. 98, 3068–3073. doi:10.1073/pnas.061562898
Roelofs, M. J. E., Temming, A. R., Piersma, A. H., van den Berg, M., and van Duursen, M. B. M. (2014). Conazole Fungicides Inhibit Leydig Cell Testosterone Secretion and Androgen Receptor Activation In Vitro. Toxicol. Rep. 1, 271–283. doi:10.1016/j.toxrep.2014.05.006
Roumie, C. L., and Griffin, M. R. (2004). Over-the-Counter Analgesics in Older Adults. Drugs & Aging 21, 485–498. doi:10.2165/00002512-200421080-00001
Schwartz, C. L., Christiansen, S., Vinggaard, A. M., Axelstad, M., Hass, U., and Svingen, T. (2019). Anogenital Distance as a Toxicological or Clinical Marker for Fetal Androgen Action and Risk for Reproductive Disorders. Arch. Toxicol. 93, 253–272. doi:10.1007/s00204-018-2350-5
Schwartz, C. L., Christiansen, S., Hass, U., Ramhøj, L., Axelstad, M., Löbl, N. M., et al. (2021). On the Use and Interpretation of Areola/Nipple Retention as a Biomarker for Anti-androgenic Effects in Rat Toxicity Studies. Front. Toxicol. 3, 730752. doi:10.3389/ftox.2021.730752
Sharpe, R. M. (2020). Androgens and the Masculinization Programming Window: Human-Rodent Differences. Biochem. Soc. Trans. 48, 1725–1735. doi:10.1042/BST20200200
Sheehan, D. J., Hitchcock, C. A., and Sibley, C. M. (1999). Current and Emerging Azole Antifungal Agents. Clin. Microbiol. Rev. 12, 40–79. doi:10.1128/CMR.12.1.40
Simpson, E. R., Clyne, C., Rubin, G., Boon, W. C., Robertson, K., Britt, K., et al. (2002). Aromatase-A Brief Overview. Annu. Rev. Physiol. 64, 93–127. doi:10.1146/annurev.physiol.64.081601.142703
Skakkebaek, N. E., Rajpert-De Meyts, E., Buck Louis, G. M., Toppari, J., Andersson, A.-M., Eisenberg, M. L., et al. (2016). Male Reproductive Disorders and Fertility Trends: Influences of Environment and Genetic Susceptibility. Physiol. Rev. 96, 55–97. doi:10.1152/physrev.00017.2015
Sobel, J. D. (2007). Vulvovaginal Candidosis. The Lancet 369, 1961–1971. doi:10.1016/S0140-6736(07)60917-9
Svingen, T., and Koopman, P. (2013). Building the Mammalian Testis: Origins, Differentiation, and Assembly of the Component Cell Populations. Genes Dev. 27, 2409–2426. doi:10.1101/gad.228080.113
Taxvig, C., Hass, U., Axelstad, M., Dalgaard, M., Boberg, J., Andeasen, H. R., et al. (2007). Endocrine-disrupting Activities In Vivo of the Fungicides Tebuconazole and Epoxiconazole. Toxicol. Sci. 100, 464–473. doi:10.1093/toxsci/kfm227
Taxvig, C., Vinggaard, A. M., Hass, U., Axelstad, M., Metzdorff, S., and Nellemann, C. (2008). Endocrine-disrupting Properties In Vivo of Widely Used Azole Fungicides. Int. J. Androl. 31, 170–177. doi:10.1111/j.1365-2605.2007.00838.x
Thankamony, A., Pasterski, V., Ong, K. K., Acerini, C. L., and Hughes, I. A. (2016). Anogenital Distance as a Marker of Androgen Exposure in Humans. Andrology 4, 616–625. doi:10.1111/andr.12156
Tiboni, G. M., Marotta, F., Del Corso, A., and Giampietro, F. (2006). Defining Critical Periods for Itraconazole-Induced Cleft Palate, Limb Defects and Axial Skeletal Malformations in the Mouse. Toxicol. Lett. 167, 8–18. doi:10.1016/j.toxlet.2006.08.004
Tiboni, G. M., Marotta, F., and Castigliego, A. P. (2008). Teratogenic Effects in Mouse Fetuses Subjected to the Concurrent In Utero Exposure to Miconazole and Metronidazole. Reprod. Toxicol. 26, 254–261. doi:10.1016/j.reprotox.2008.09.005
Trösken, E. R., Fischer, K., Völkel, W., and Lutz, W. K. (2006). Inhibition of Human CYP19 by Azoles Used as Antifungal Agents and Aromatase Inhibitors, Using a New LC-MS/MS Method for the Analysis of Estradiol Product Formation. Toxicology 219, 33–40. doi:10.1016/j.tox.2005.10.020
U.S. Environmental Protection Agency (2021). CompTox Chemicals Dashboard. Available at: https://comptox.epa.gov/dashboard (Accessed February 21, 2022).
U.S. FDA (2020). Drug Applications for Over-the-counter (OTC) Drugs | FDA. Available at: https://www.fda.gov/drugs/types-applications/drug-applications-over-counter-otc-drugs (Accessed February 8, 2022).
Van der Pas, R., Hofland, L. J., Hofland, J., Taylor, A. E., Arlt, W., Steenbergen, J., et al. (2012). Fluconazole Inhibits Human Adrenocortical Steroidogenesis In Vitro. J. Endocrinol. 215, 403–412. doi:10.1530/JOE-12-0310
Vinggaard, A. M., Hnida, C., Breinholt, V., and Larsen, J. C. (2000). Screening of Selected Pesticides for Inhibition of CYP19 Aromatase Activity In Vitro. Toxicol. Vitro 14, 227–234. doi:10.1016/S0887-2333(00)00018-7
Vinggaard, A. M., Christiansen, S., Laier, P., Poulsen, M. E., Breinholt, V., Jarfelt, K., et al. (2005). Perinatal Exposure to the Fungicide Prochloraz Feminizes the Male Rat Offspring. Toxicol. Sci. 85, 886–897. doi:10.1093/toxsci/kfi150
Vinggaard, A. M., Hass, U., Dalgaard, M., Andersen, H. R., Bonefeld-jorgensen, E., Christiansen, S., et al. (2006). Prochloraz: an Imidazole Fungicide with Multiple Mechanisms of Action. Int. J. Androl. 29, 186–192. doi:10.1111/J.1365-2605.2005.00604.x
Welsh, M., Saunders, P. T. K., Fisken, M., Scott, H. M., Hutchison, G. R., Smith, L. B., et al. (2008). Identification in Rats of a Programming Window for Reproductive Tract Masculinization, Disruption of Which Leads to Hypospadias and Cryptorchidism. J. Clin. Invest. 118, 1479–1490. doi:10.1172/JCI34241
Westerlund, T., Barzi, S., and Bernsten, C. (2017). Consumer Views on Safety of Over-the-counter Drugs, Preferred Retailers and Information Sources in Sweden: after Re-regulation of the Pharmacy Market. Pharm. Pract. 15, 894. doi:10.18549/PharmPract.2017.01.894
Wilkins, L., Jones, H. W., Holman, G. H., and Stempfel, R. S. (1958). Masoulinization of the Female Fetus Associated with Administration of Oral and Intramuscular Progestins during Gestation: Non-adrenal Female Pseudohermaphrodism*. J. Clin. Endocrinol. Metab. 18, 559–585. doi:10.1210/JCEM-18-6-559
Williams, A. J., Grulke, C. M., Edwards, J., McEachran, A. D., Mansouri, K., Baker, N. C., et al. (2017). The CompTox Chemistry Dashboard: a Community Data Resource for Environmental Chemistry. J. Cheminform. 9, 61. doi:10.1186/s13321-017-0247-6
Wolf, C., Lambright, C., Mann, P., Price, M., Cooper, R. L., Ostby, J., et al. (1999). Administration of Potentially Antiandrogenic Pesticides (Procymidone, Linuron, Iprodione, Chlozolinate, P,p′-DDE, and Ketoconazole) and Toxic Substances (Dibutyl- and Diethylhexyl Phthalate, PCB 169, and Ethane Dimethane Sulphonate) during Sexual Differentiation Produces Diverse Profiles of Reproductive Malformations in the Male Rat. Toxicol. Ind. Health 15, 94–118. doi:10.1177/074823379901500109
Ylä-Rautio, H., Siissalo, S., and Leikola, S. (2020). Drug-related Problems and Pharmacy Interventions in Non-prescription Medication, with a Focus on High-Risk Over-the-counter Medications. Int. J. Clin. Pharm. 42, 786–795. doi:10.1007/S11096-020-00984-8
Keywords: fluconazole, reproduction, conazoles, endocrine disrupting chemicals, clotrimazole, reproductive disorders, testis, androgen
Citation: Draskau MK and Svingen T (2022) Azole Fungicides and Their Endocrine Disrupting Properties: Perspectives on Sex Hormone-Dependent Reproductive Development. Front. Toxicology 4:883254. doi: 10.3389/ftox.2022.883254
Received: 24 February 2022; Accepted: 31 March 2022;
Published: 28 April 2022.
Edited by:
David Møbjerg Kristensen, Copenhagen University Hospital, DenmarkReviewed by:
Anderson J Martino-Andrade, Universidade Federal do Paraná, BrazilCopyright © 2022 Draskau and Svingen. This is an open-access article distributed under the terms of the Creative Commons Attribution License (CC BY). The use, distribution or reproduction in other forums is permitted, provided the original author(s) and the copyright owner(s) are credited and that the original publication in this journal is cited, in accordance with accepted academic practice. No use, distribution or reproduction is permitted which does not comply with these terms.
*Correspondence: Terje Svingen, tesv@food.dtu.dk