- 1Department of Endocrine and Metabolic Diseases, First Teaching Hospital of Tianjin University of Traditional Chinese Medicine, Xiqing, Tianjin, China
- 2Tianjin Key Laboratory of Translational Research of TCM Prescription and Syndrome, First Teaching Hospital of Tianjin University of Traditional Chinese Medicine, Xiqing, Tianjin, China
- 3National Clinical Research Center for Chinese Medicine Acupuncture and Moxibustion, Xiqing, Tianjin, China
Diabetic cardiomyopathy (DCM) is a pathophysiological condition of cardiac structure and function changes in diabetic patients without coronary artery disease, hypertension, and other types of heart diseases. DCM is not uncommon in people with diabetes, which increases the risk of heart failure. However, the treatment is scarce, and the prognosis is poor. Since 1972, one clinical study after another on DCM has been conducted. However, the complex phenotype of DCM still has not been fully revealed. This dilemma hinders the pace of understanding the essence of DCM and makes it difficult to carry out penetrating clinical or basic research. This review summarizes the literature on DCM over the last 40 years and discusses the overall perspective of DCM, phase of progression, potential clinical indicators, diagnostic and screening criteria, and related randomized controlled trials to understand DCM better.
Introduction
The current diabetes mellitus (DM) prevalence is 463 million, 9.3% of the world population (1). In this vast group, heart failure (HF) has emerged as the most common cardiovascular complication of diabetes (2). Meanwhile, patients with type 2 diabetes mellitus (T2D) are more likely to be hospitalized and re-admitted for HF and have a higher risk of cardiovascular and all-cause mortality than those without diabetes and HF (3, 4); this may be due to long-term DM leading to pathological changes that contribute to the development and progression of HF, including myocardial structural, functional, and metabolic changes (5), independent of myocardial ischemia or atherosclerotic disease processes.
This distinct clinical entity was first proposed by Lundbaek (6) in 1954 as diabetic heart disease independent of hypertension and coronary artery disease (CAD) that commonly coexist with T2D. In 1972, the existence of DCM had been confirmed through postmortem pathological findings in four patients with diabetes who manifested HF symptoms, and DCM became validated as a distinct entity (2). Bertoni et al. (7) conducted a large nationwide case-control study in the United States in 1995, which confirmed an association between nonischemic idiopathic cardiomyopathy and diabetes. After these initial studies, DCM gained increased attention from epidemiologists and clinicians.
Despite the rapid increase in the number of preclinical and clinical studies on diabetic cardiomyopathy in the past decades, the process of DCM remains unclear. As a result, no consensus has been reached regarding the most effective preventive or therapeutic approaches to diabetic cardiomyopathy. For this reason, this review summarizes the recent theory and clinical finding achievements to understand this controversial disease better.
Phases of progression
Diabetic cardiomyopathy is organic heart disease. The exact evolution of DCM pathological changes has not been fully studied. The existing Spatio-temporal evolution models of DCM are generally divided into two categories. One, which is the traditional understanding, is that DCM has only one phenotype, from diastolic to systolic dysfunction, and is accompanied by structural remodeling such as left ventricular hypertrophy (LVH), which is a gradual development process. The other believes that DCM is a disease with two independent phenotypes. The diastolic dysfunction phenotype eventually develops into HFpEF, and the systolic dysfunction phenotype finally develops into HFrEF, which is a new understanding.
Specifically, five specific Spatio-temporal models have been proposed, and their pathological mechanisms have been briefly summarized (Figure 1). Moreover, a hypothesis is argued: there may be subclinical hyperfunction in the ultra-early stage of DCM.
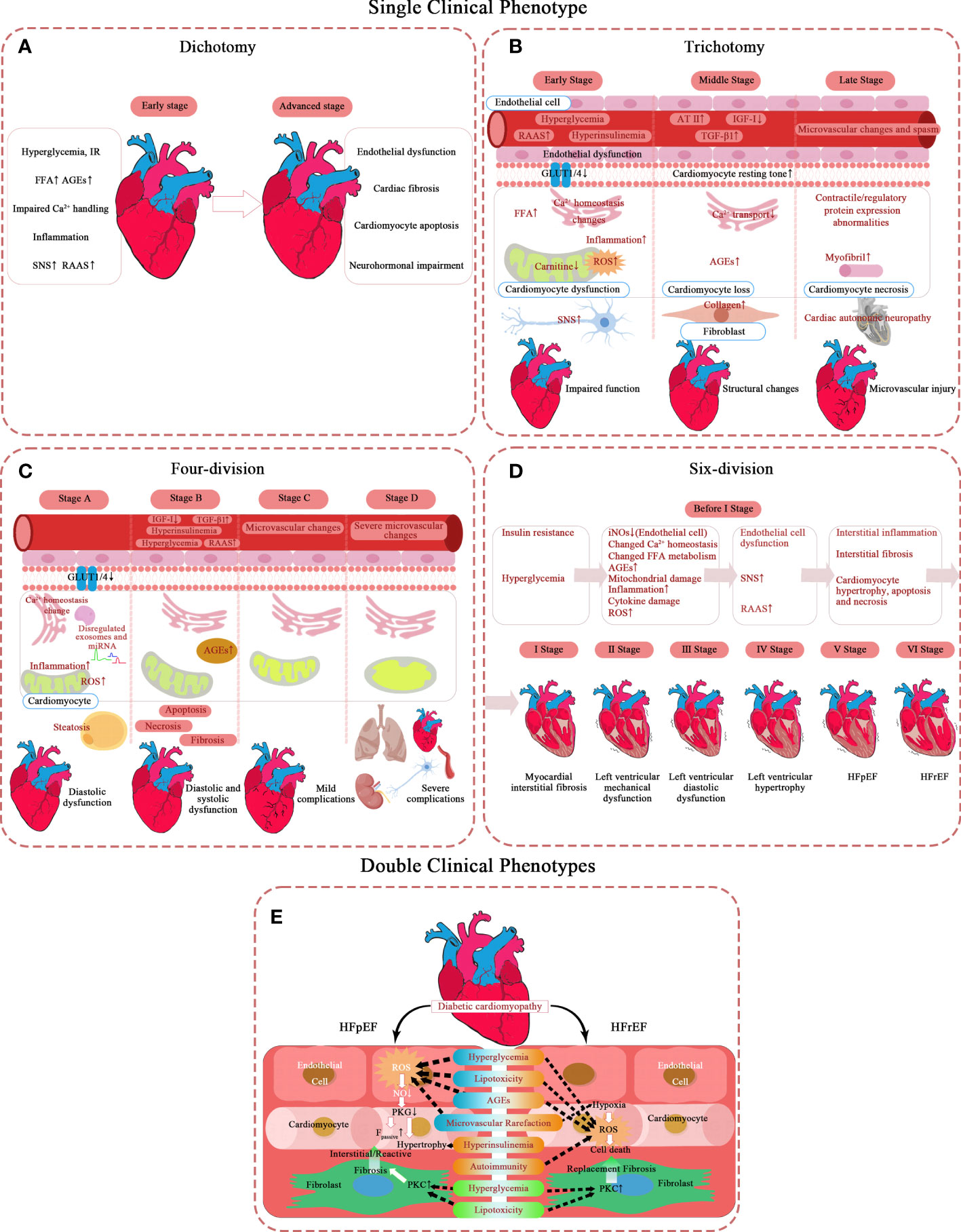
Figure 1 Pathological mechanism of DCM in different types of phases of progression. (A–D) Single clinical phenotype. (E) Double clinical phenotypes. AT-II, angiotensin II; AGEs, advanced glycation end-products; Fpassive, cardiomyocyte resting tension; FFA, free fatty acid; GLUT1, glucose transporter 1; GLUT4, glucose transporter 4; HFrEF, heart failure with reduced ejection fraction; HFpEF, heart failure with preserved ejection fraction; IR, insulin resistance; IGF-I, insulin-like growth factor 1; NO, nitric oxide; PKC, protein kinase C; PKG, protein kinase G; RAAS, renin-angiotensin-aldosterone system; ROS, reactive oxygen species; SNS, sympathetic nervous system; TGF-β1, transforming growth factor β1.
Single clinical phenotype
Dichotomy
The simplest model describes DCM as two stages, mainly in the left ventricle (8). The first is the stage of diastolic dysfunction, which is asymptomatic, but has a series of characteristics of ultrasonic cardiogram (9): elevated LV end-diastolic pressure, increased ventricular stiffness, and possibly accompanied by left ventricular concentric hypertrophy and left atrial enlargement.
The second stage of HF displays clinical symptoms and signs based on systolic dysfunction and severe cardiac remodeling, such as LVH and terminal LV dilatation.
Trichotomy
Scholars who hold the trisection view believe diabetic cardiomyopathy successively involves cardiac function – cardiac structure – microvascular (10–20). It takes years to induce significant LV dysfunction by gradually accumulating subcellular structural damage at the beginning (10). Diastolic dysfunction is the earliest clinical abnormality, with myocardial fibrosis and hypertrophy as the main reason. Systolic dysfunction only develops in the late stage of the disease (10) and often coexists with severe CAD and cardiac autonomic neuropathy (CAN) (11).
The early stage lasts only a short time. The entire initial stage is completely asymptomatic (12). At the very beginning, hyperglycemia and insulin resistance (IR) of DM patients have led the metabolic disorders in their hearts (21). At this stage, the heart structure is close to normal as a result of the compensatory adaptation of the heart to metabolic disorders, and only changes in myocardial cell substructure and endothelial dysfunction are observed (22–24). First, the level of GLUT4 on myocardial cell membrane decreases (25), and the activity of PPARα increases (26), which decreases the level of intracellular glucose oxidation (GLOX) (27). On the other hand, the signal transduction mediated by insulin receptor increases (28), which promotes the transport of fatty acid transferase (FAT/CD36) to the plasma membrane (29), and then increases the uptake of FA and the level of fatty acid oxidation (FAO). These unbalanced substrate metabolisms reduce the efficiency of myocardial ATP production, so that work efficiency of cardiomyocytes deteriorates. Discrete subclinical diastolic dysfunction can be detected clinically. The initial characteristics of DCM are increased atrial filling, decreased ventricular early diastolic filling, and increased myocardial relaxation damage and stiffness (10). The ventricular filling reduction is characterized by a slow E acceleration peak, deceleration peak, and peak filling rate, which can be captured by magnetic resonance imaging (30). Echocardiographic findings of ventricular septal annual wall motion damage also confirmed this (13). It is worth mentioning that the decrease of myocardial blood flow reserve can be identified by load imaging technology (31), which may be related to impaired insulin signal transduction (14).
In the middle stage, the aggravation of myocardial cell injury makes diastolic cardiac function significantly abnormal, but the ejection function is only slightly affected (EF 40%-50%) (15). With the progress of the disease, the effects of metabolic disorders gradually expand, in which dysfunctional mitochondria is a key role (32). The increase of FAO and the decrease of GLOX lead to the accumulation of toxic lipid metabolites, like DAG and ceramide, and the increase of oxidative stress, especially the release of ROS and NOS in cardiomyocytes (33, 34). Lipotoxicity caused by toxic lipid metabolites can lead to the remodeling of mitochondrial membrane, and oxidative stress can damage the proteins involved in oxidative phosphorylation (35) and activate uncoupling protein (UCP) (36), further destroying the function of mitochondria. In addition, under the influence of hyperglycemia, the levels of advanced glycation end products (AGE) increases both inside and outside the cells, and their formation on the SERCA2a and Ryr of the sarcoplasmic reticulum interfered with the dynamics of Ca2+ (37, 38), thus affecting the function of mitochondria (39). Mitochondria plays as a mediator in this process, which activates the apoptotic cascades under the influence of abovementioned factors (40). In short, the subcellular mechanisms, such as impaired insulin signal transduction, mitochondria metabolic disorders and impaired calcium dynamics, lead to cardiomyocyte loss and fibrosis, and may lead to mild CAN (15). This stage begins to show clinical symptoms. Although the change in cardiac structure is still slight, it can be detected by conventional echocardiography: LV diameter, wall thickness, or mass increases, and compliance decreases (14). Myocardial microvascular structural damage is not obvious at this stage.
This stage is a critical period of fibrosis progression because cardiac magnetic resonance (CMR) can detect diffuse fibrosis (16). The increase of perivascular and myofibrillar interstitial fibrosis was observed in the myocardial samples without coronary heart disease and hypertension (41). Stiff collagen and its crosslinks accumulate in the heart interstitial, causing the gradual loss of muscle cells. Notably, this collagen deposition and accumulation of advanced glycation end products (AGEs) are important features of increased LV stiffness in heart failure patients with reduced EF, which may be related to the impairment of ejection function (41).
In the last stage, cardiac interstitial fibrosis exacerbates and eventually turns into HFrEF. Patients will have prominent exercise intolerance (12). The change in myocardial microcirculation is noticeable now. Severe collagen deposition results in coronary arteriolar sclerosis, basement membrane thickening, and capillary microaneurysm (20). Cardiac remodeling exceeds myocardial repair capacity. LV mass, volume, and wall thickness continue to increase (12, 15), accompanied by LV dilatation. At this time, focal fibrosis can be detected by positron emission tomography (PET), computed tomography (CT), and magnetic resonance imaging (MRI) (16). Based on diastolic dysfunction, the signs of systolic dysfunction begin to appear, manifested as shortened ejection period, prolonged performance before ejection, and increased filling pressure. Ischemic heart disease and severe CAN are the two major complications at this stage (17–20). At the subcellular level, increased ROS and inflammation (13), contractile and regulatory protein expression abnormalities, and impaired myocardial insulin signal transduction lead to decreased endothelial nitric oxide synthase activation and bioavailable nitric oxide levels (42).
Even if this trisection model seems perfect, some believe that LVH is the first performance of the first stage rather than in the middle (15). Because at the cellular level, the activation of neurohumoral mechanisms such as hyperglycemia, insulin resistance, renin-angiotensin-aldosterone system (RAAS), and sympathetic nervous system (SNS) lead to cardiomyocyte hypertrophy, stiffness, and fibrosis, which is sufficient to trigger this process. Meanwhile, free fatty acid (FFA) accumulation, calcium homeostasis imbalance, and GLUT-1 and -4 depletion contribute to myocyte injury at the molecular level (15).
Furthermore, some pathological mechanisms like microvascular perfusion damage remain debatable (16). The microcirculation disturbance is recognized in the late stage of this disease, but it is not clear in the early stage. The current known decrease in myocardial blood flow reserve is most likely related. DCM is undoubtedly a DM microcirculation complication if microcirculation disturbance runs through the process.
Four-division
Some scholars divide DCM into four stages with a clear hierarchy based on NYHA classification and AHA staging (43–46). They emphasize that DCM is the earliest contributor to HF in patients with DM in an ideal state. In this model, the presence or absence of comorbidities is regarded as the watershed of HF. The early stages (stages A and B) are characterized by simple DCM, mainly caused by the pathophysiology of diabetes. The latter stages (stages C and D) show that DCM coexists with other complications, and diabetes is a secondary factor. This scheme extends the traditional definition of diabetic cardiomyopathy, considering the pathophysiological characteristics, echocardiography changes, and periodic changes in serum biomarkers. The stages are:
Stage A (diastolic dysfunction): no clinical symptoms. Patients usually have normal cardiac structure and function, at most subclinical cardiac structure and function abnormalities, normal ejection fraction (LVEF), and no non-DCM complications. However, patients often show hypertrophic and restrictive phenotypes because LVH increases ventricular stiffness, leading to diastolic dysfunction. This is the earliest stage of DCM and can be detected in 28% to 75% of asymptomatic diabetic patients (14). Finally, 36.9% of patients with stage A will progress to symptomatic heart failure (47).
Stage B (systolic and diastolic dysfunction): mild/moderate physical activity limitation. Structural and functional abnormalities appear in the heart, but no non-DCM complication exists. In addition to diastolic dysfunction, patients may have decreased ventricular dilatation and ejection fraction. Secondary complications such as coronary heart disease and hypertension are possible but are not severe.
Stage C (mild complications): severe physical activity limitation. Cardiac dysfunction and LVEF decline worsen. Non-DCM complications, such as hypertension, microvascular disease, and viral heart disease, begin to appear. Patients may have myocarditis and coronary atherosclerosis but no coronary heart disease (14).
Stage D (severe complications): there are still symptoms or imminent death at rest. Biventricular refractory heart failure lasts. Non-DCM complications are severe, including dilatation, fibrosis, microvascular and macrovascular lesions, and obvious native coronary heart disease. It can also be combined with myocardial overload after myocardial infarction, with or without hypertensive heart disease.
In addition, there are different beliefs. Because DM is a clear risk factor for HF, some researchers believe it enters phase A as long as it occurs. Further progress is manifested as simple diastolic dysfunction; this is a split in phase A of the above program, but this model does not refer to complications. The specific stages can be summarized as follows: stage A (risk factor stage): simple diabetes; stage B (HFpEF): left ventricular diastolic dysfunction (LVDD) without symptoms but with LVH; phase C (HFrEF): EF decreased with obvious symptoms and signs such as dyspnea and pulmonary congestion; stage D (terminal stage): heart failure that is difficult to treat (48).
Six-division
After nearly 30 years of accumulation of CMR-derived myocardial mechanics data, according to the order of structure and dysfunction, a view of the DCM six points method was created (49).
In the first stage, myocardial interstitial fibrosis occurs; this is detected in a diagnostic test. Researchers used sensitive methods to measure the left ventricle’s multidirectional strain and strain rate. The overall longitudinal, circumferential, and radial strains were not significantly different compared to the healthy control group, but ECV (extracellular volume, representing interstitial fibrosis) in T2D patients was significantly high (50). AGEs accumulation or myocardial interstitial neovascularization may cause ECV dilatation. An animal study also showed that ECV first increased after three months of diabetes induction. The ultrasonic-derived LV radial strain rate changed after six months, and radial strain damage was observed after nine months (51). These studies have confirmed that extracellular fibrosis develops first, and mechanical function impairs. The early stage of interstitial fibrosis may not be sufficient to cause mechanical damage to the left ventricle, which is the reason for independently dividing it into stages.
The second stage of diabetic cardiomyopathy is LV mechanical dysfunction. Echocardiography and nuclear magnetic resonance studies have shown that in the systolic and early diastolic stages (52), the multidirectional strain of myocardial layers in diabetic patients decreased significantly (53–56), and a few strains increased compensatorily (53). Liu et al. (57) confirmed the decrease of diastolic longitudinal and circumferential strain rates in patients with T2D. In contrast, longitudinal strain and peak systolic longitudinal strain rates decreased in patients with T2D over 5 years (57).
The third stage is LVDD. Nuclear magnetic resonance (30, 58) studies have shown diastolic dysfunction in pre-diabetes (59), type 1 diabetes (T1D) (60, 61), and T2D (55) patients (children (62) and adults (63)). Moreover, from this stage on, changes can be detected by conventional echocardiography (55, 59, 60).
The fourth stage is LVH, the fifth stage is HFpEF, and the final stage is HFrEF. Not all diabetic patients will progress through all stages.
Double clinical phenotypes
One thing in common in the above views regardless of the number of DCM phases: admitting that DCM has only one phenotype. However, some scholars believe that DCM has two phenotypes. Furthermore, these two phenotypes are not continuous stages of DCM but independently evolved into heart failure with LVEF preservation or reduction (64).
Researchers have found the phenotype-specific mechanisms of HFpEF and HFrEF, namely coronary microvascular endothelial dysfunction in HFpEF and myocardial cell death in HFrEF. The involvement priority of endothelial cell or cardiomyocyte cell compartments determines the development direction of DCM. In obese patients with T2D, abnormal glucose, lipid metabolism, and insulin resistance coexist and tend to occur in DCM’s restrictive/HFpEF phenotype. T1D patients with autoimmune tendency are more likely to develop into dilated/HFrEF phenotype.
A hypothesis: subclinical hyperfunction
Early DCM was not well understood. It was generally believed before that the cardiac function of patients with DCM remained normal initially. However, a new hypothesis is that DCM has a stage of myocardial systolic hyperfunction (65). This myocardial hyperdynamic state is short-lived and is the onset of the asymptomatic subclinical stage.
Based on the findings of a clinical study, this hypothesis adds to the early stage of DCM evolution. Hensel KO et al. used speckle tracking echocardiography to study the short course (4.3 ± 3.5 years) of type 1 diabetic adolescents without complications (62). Compared to the healthy control group, patients with type 1 diabetes showed overall longitudinal and circumferential LV myocardial systolic ability enhancement under rest and load. However, this situation is more evident in patients with a longer disease course, indicating that a high dynamic state may continue for several years.
Some evidence has been found in human and animal models. M-mode and Doppler ultrasound studies found that LV systolic ability increased in children with simple diabetes (66, 67). This phenomenon occurred only in normal albuminuria. When microalbuminuria occurred, LV systolic ability returned to normal (68). Studies on MRI also found that young adults with T1D showed signs of increased LV torsion at the early stage (69, 70). Few studies have revealed its pathophysiological mechanism. Only one animal model study suggests that it may be associated with increased plasma volume and sympathetic activation (27).
In short, this high LV systolic capacity is more like compensation for reduced myocardial efficiency. However, this is not widely reported because myocardial hypercontraction has no clinical symptom and can only be detected using sensitive methods. Therefore, more clinical data are required to confirm this hypothesis.
Overall clinical findings
Many studies have reported that DCM does not have any obvious clinical manifestations. It has nonspecific symptoms and signs of heart failure only when it progresses to the advanced stage. Therefore, auxiliary examination methods are the only ways to diagnose DCM. Previously, it was difficult to diagnose DCM because noninvasive techniques were inaccurate. Restrictive phenotype determination still required cardiac catheterization, while dilated phenotype required myocardial biopsy (48, 71); this made it difficult for humans to uncover the mysterious veil of DCM.
Noninvasive cardiac imaging has come a long way in the last 40 years, and because of its safety, simplicity, and accuracy, it is becoming increasingly important in diagnosing DCM. Nowadays, people can characterize DCM in metabolism, structure, and function. Numerous diagnostic clinical findings have been accumulated. They are summarized and listed below (Tables 1–3). All cardiac indicators differ meaningfully between diabetic patients and healthy people are included. Because DCM’s clinical phenotypes and mechanisms are different in T1D and T2D, we sorted them out according to the type (64, 166). Given that the mixed population study (including T1D and T2D) still has a certain reference value, we summarized these studies together because it represents the general rule of DM.
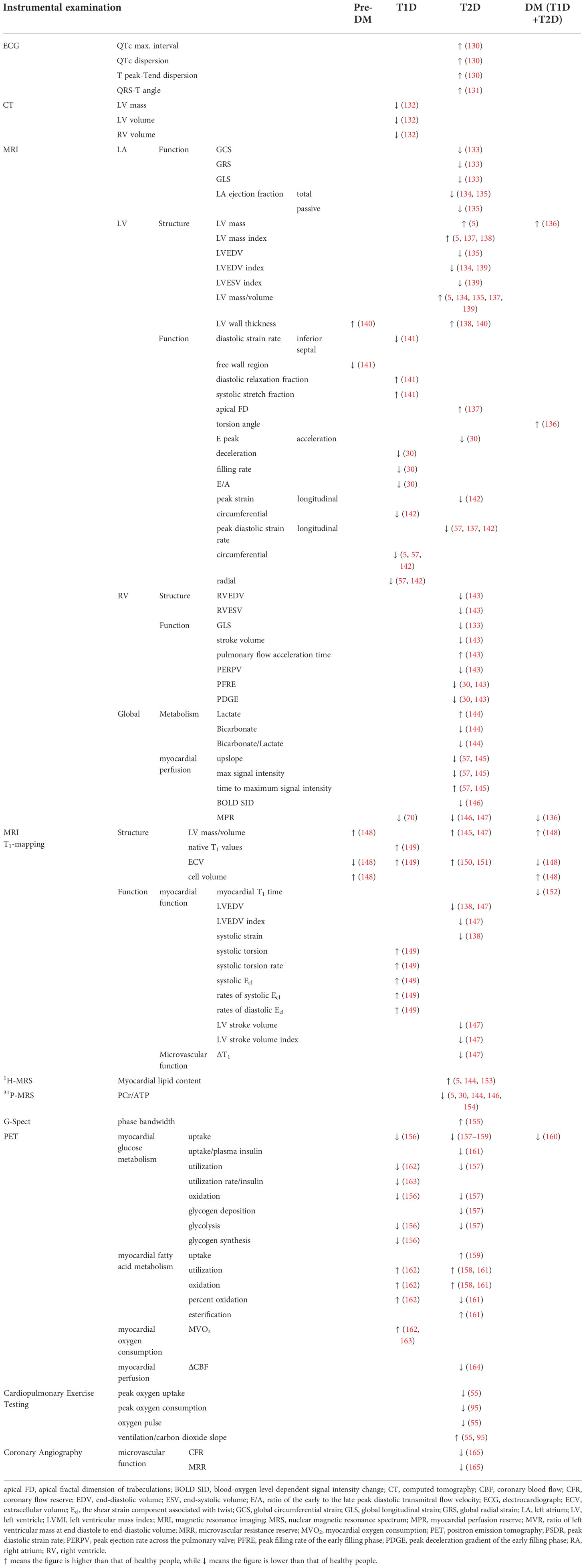
Table 3 Main clinical findings of CT/MRI/PET and other methods for cardiac dysfunction in T1D and T2D patients.
Serological markers, echocardiography, cardiac magnetic resonance imaging, and positron emission tomography are widely used as the main tools to explore the changes in DCM heart. Because CMR is readily unavailable and expensive and has contraindications, and may not be suitable for patients with autism or metal implants, the application of CMR remains in the field of scientific research. Although echocardiography has inter-observer variability, and the assessment of right ventricular structure and function is often more nonstandard in the heart’s four chambers, it is generally the preferred method for diagnosing DCM and tracking disease progression (48). Adding a series of new serological markers makes it more possible to implement a large screening because this is the simplest method. Many plasma/serum substances reflect changes in myocardial metabolism, structure, and function and may indicate prognosis (167). Metabolic changes in DCM can be detected using emerging magnetic resonance spectroscopy imaging, which appears to be the earliest detectable change in DCM. We also included DCM heart changes found by invasive techniques (Tables 2, 3), hoping to present a full spectrum of DCM lesions.
Screening and diagnosis criteria
Screening criterion
Diabetic cardiomyopathy screening knowledge is still insufficient to build up a criterion, but some useful information has emerged. Asymptomatic patients with T1D or T2D are considered to need further examination when they have risk factors as follows (168) (1): Longstanding DM (2); Poorly controlled DM (3); Microvascular complications of DM: diabetic kidney disease (↑UACR, ↓eGFR), retinopathy, and neuropathy. It is thought to be more suspicious if evidence of cardiac LV dysfunction or LVH as well as exercise intolerance can be found by doppler ultrasound or cardiopulmonary exercise test (168, 169). Additionally, five serological or urinary markers may be currently available for screening. Earlier researches have shown that, while BNP is a more visible indicator of diastolic dysfunction than hs-CRP, its sensitivity and specificity are low (170, 171). However, with the advancement of research, increasing evidence has confirmed that BNP or NT-proBNP is closely related to heart failure and is still recommended as an early screening for DCM (172, 173). Two prospective randomized controlled trials have confirmed that a new standard (BNP≥50 pg/mL or NT-proBNP>125 pg/ml) can screen high-risk DM populations properly to reduce the incidence of cardiovascular hospitalizations/death or heart failure (173, 174). A study has also shown that NT-proBNP level below 125 pg/mL helps role out the possibility of asymptomatic LV dysfunction in the DM population (73). What’s more, HbA1c levels are proportional to the degree and frequency of diastolic dysfunction (175). Interestingly, testing microalbuminuria in people with diabetes helps identify their risk of diastolic dysfunction (78). However, the detection results of these indicators cannot directly confirm myocardial changes, so they must be determined by imaging tests.
Diagnosis criterion
As mentioned above, diabetic cardiomyopathy shows metabolic, structural, and functional changes. Therefore, many scholars are trying to find a cut-off point to establish the diagnosis of DCM. A study published in 2013 provided initial diagnosis clues of DCM (176). After other heart diseases are ruled out, changes as follows should be evaluated: The structural changes include (1) LV hypertrophy assessed by 2D echocardiography or CMR (2); Increased integrated backscatter in the LV (septal and posterior wall); and (3) Late Gd-enhancement of the myocardium in CMR. The functional changes include (1) LVDD assessed by pulsed Doppler echocardiography and TDI (2); LV systolic dysfunction (LVSD) demonstrated by TDI/SRI; and (3) Limited systolic and/or diastolic functional reserve assessed by exercise TDI. The metabolic changes include (1) Reduced cardiac PCr/ATP detected by 31P-MRS; (2) Elevated myocardial triglyceride content detected by 1H-MRS.
In 2015, researchers proposed different phenotypes of DCM development and their diagnostic criteria (64). The phenotypes should both meet the following conditions first: (1) Presence of DM; (2) Exclusion of CAD, valvular, or congenital heart disease; and (3) Exclusion of hypertensive heart disease (=DBP<90 mmHg). Based on this, the diagnostic criterion of Dilated/HFrEF Phenotype should include (1) Exclusion of viral myocarditis by endomyocardial biopsy; (2) LVEF<50%, LVEDVI>97 mL/m2. While the criterion of Restrictive/HFpEF Phenotype should include: (1) Exclusion of infiltrative heart disease by endomyocardial biopsy; (2) LVEF>50%, LVEDVI<97 mL/m2; and (3) E/e’ >15 or 8<E/e’ <15+LAVI>40 mL/m2 or 8<E/e’ <15+BNP>200 pg/mL or 8<E/e’ <15+atrial fibrillation or 8<E/e’<15+LVH (LVMI♀>122 g/m2; LVMI♂>149 g/m2).
In 2021, a study (168) put forward a new diagnostic criterion based on the four-division method. Acknowledging only one phenotype exists, they believe stage B is the best time to diagnose DCM. Patients should have at least one of the following echocardiographic abnormalities: (1) LVH, defined as LV mass index (LVMi) > 115 g/m2 in men and > 95 g/m2 in women; (2) LAE, defined as left atrial volume index (LAVi) ≥ 34 mL/m2; (3) abnormal ratio of mitral inflow peak early diastolic velocity (E) to tissue Doppler mitral annular early diastolic velocity (e’), defined as E/e’ ≥ 13; (4) impaired GLS, defined as GLS < 18%.
Clinical practice
Currently, the treatment for DCM is mainly divided into three kinds: conventional cardiovascular drugs, anti-glycemic drugs, and new therapies such as CoQ10, MicroRNA and Stem cell therapy (14). Many reviews have focused on the outcomes of the trials of these therapies (14, 168, 177). Although the number of the trials is large, most of them recruited mixed population, resulting in invalid data for judging whether it is effective for treating DCM. What’s worse, each kind has certain limitations now. For example, conventional cardiovascular drugs only apply when DCM develops into more obvious cardiac symptoms. Conventional anti-glycemic drugs have an insignificant benefit, and only SGLT2i (a new kind of anti-glycemic drug) is recommended as the first-line medicine for DCM. Moreover, new therapies still have defects such as clinical trial failure and insignificant safety. Therefore, more studies must be conducted to find suitable drugs. The following table (Table 4) summarizes the randomized controlled trials (RCTs) related to DCM in the last 10 years, hoping to inspire clinical drug use.
Discussion
Phase of progression
Although the progression phase contains some theoretical components, it stems from our understanding of the entire disease process, which has important guiding significance for clinical practice and scientific research. The differences among them are mainly reflected in three points: the phenotypes of the disease, the beginning of the disease, and the stages of the disease. Different phenotypes require different treatments, which is relevant to developing guidelines. If DCM only has one phenotype, it is necessary to consider the secondary prevention of HFpEF progression to HFrEF, and appropriate animal models should also be considered in basic research. If DCM exhibits two distinct phenotypes, clinical trials and basic research must be divided into sub-fields to be further investigated. It is also important to define the beginning of the disease. Although many researchers agree that the myocardial injury caused by diabetes begins at the onset of diabetes, some scholars have pointed out through epidemiological analysis that the natural history of diabetic cardiomyopathy has begun as early as the metabolic syndrome period. Patients who have elevated inflammatory markers and microalbuminuria are at risk of developing heart failure (14). Therefore, clarifying the starting point of DCM is of great value for formulating disease screening strategies, and early treatment can delay the progress of DCM. Furthermore, the division of disease stages, which provides strong support for clinicians in evaluating the severity of DCM patients and selecting the intervention time, should not be ignored. Moreover, it also affects the evaluation of drug efficacy because drugs have different benefits for different stages.
We have summarized all the proposed DCM Spatio-temporal evolution models (Table 5). The dichotomy is the most concise model for clinicians to master and can be easily popularized in communities. However, it should be noted that structural and metabolic changes that go unnoticed are the culprits of dysfunction. The trisection model does not advance much more than the dichotomy but emphasizes the discreteness, concealment, and progressive fibrosis of early dysfunction. Unless in medical institutions with advanced technological means, the clinical practice remains a dichotomy because early changes cannot be detected promptly.
Due to the origin of AHA and NYHA classification, the quartering model has strong practicability and popularity, which is definitely a good paradigm for examining DCM from a macro perspective and considering DCM complications. Six-division model is based on nuclear magnetic resonance, so it is more inclined to stage DCM from the perspective of imaging. If MRI is easy to obtain, this model is undoubtedly more refined, which is significant for clinical research. Unfortunately, although patients can be stratified, we lack the drugs to treat the lesions at each stage, particularly interstitial fibrosis, LV mechanical change, LVH, and HFpEF.
If DCM has two independent phenotypes, its value is enormous because it can save a lot of clinical resources to distinguish the stage and monitor the progression. The clinical test results of active cardiovascular drugs on HFpEF and HFrEF are different (64). However, the two-phenotype theory has a mixed reputation. LVSD is scattered in T2D. Most literature shows that resting LVEF in patients with T2D is normal. Therefore, whether T2D eventually develops into HFrEF is a controversial issue (9). Seferovic et al. proposed the hypothesis that T1D and T2D evolved respectively, which is convincing (64), but more research is required to confirm it. However, it was reported that LVEF in patients with relatively simple T2D at rest has a downward trend (194, 195). In fact, observational studies are mostly cross-sectional. Usually, only one of diastolic dysfunction and systolic dysfunction is included. Moreover, there is no prospective study to observe whether patients progress from diastolic to systolic dysfunction, so the evolution of DCM phenotype over time is still unknown. In addition, LVEF may improve or deteriorate during follow-up (196), and the measurement has a certain variability (197), which makes accurate classification difficult.
Notably, existing Spatio-temporal evolution models are mostly theoretical because the clinical diagnosis of DCM is challenging, so their data are mainly from experimental models (12). However, the proposed stages allow clinicians and researchers to see the whole picture. Moreover, the full spectrum of DCM clinical indicators will help optimize the evolution model and contribute to improvements in diagnosis since they all come from human studies.
An overall picture of DCM
There is evidence that early in pre-diabetes, cardiometabolic recession appears alone (153, 158), followed by myocardial hypertrophy (148). Moreover, LVDD may appear. However, until the early stage of diabetes, heart damage remains tiny and discrete. As the disease progresses, the following phenomena occur (Figure 2):
● The myocardial energy metabolism substrates lose flexibility. Specifically, glucose uptake and utilization decrease, and fatty acid uptake and utilization increase, resulting in a large number of triglyceride deposition and a decrease in ATP production (144). Although the relationship between cardiac diastolic function and energy metabolism parameters is uncertain (30, 159), it has been confirmed that cardiac triglyceride deposition and myocardial energy damage are related to concentric LV remodeling and systolic dysfunction (138).
● Myocardial deformability decreases before diastolic dysfunction. Three layers of LV myocardium, including subendocardial (longitudinal fiber), medium (radial fiber), and subepicardial (circumferential fiber), are all damaged. Similar to the coronary ischemic myocardial injury (198), the damage to the subendocardial myocardium is the most serious, followed by the middle myocardium and the subepicardial myocardium (55). As for the left atrium, the function of all segments in the whole cardiac cycle is damaged (115). The unique feature of T1D is that the myocardial deformability of children is intact. The circumferential strain of the epicardium and middle layer and the longitudinal strain of the whole layer begins to decrease in late adolescence, followed by the decrease of conventional cardiac parameters years after (121).
● Remodeling involves in three chambers (left atrium, left and right ventricle), with systolic and diastolic function all impaired. LV remodeling is concentric hypertrophy characterized by an absolute and relative increase in LV wall thickness, increased LV mass (about 3.5 g) (136), and reduced LV cavity volume. RV remodeling is also restrictive, characterized by increased right ventricular stiffness and reduced right ventricular volume, similar to LV (143). In many diabetic patients, systolic and diastolic dysfunction often go hand in hand (103). In severe cases, LVEF and RVEF decreases.
● The epicardial adipose tissue becomes thickened.
● Myocardial perfusion decreases in both coronary artery and microvessels. This change is sufficient to be detected at rest and more obvious at stress (dobutamine and exercise).
● Cardiopulmonary function decreases, manifested as a decrease in myocardial oxygen uptake, and increase in oxygen consumption.
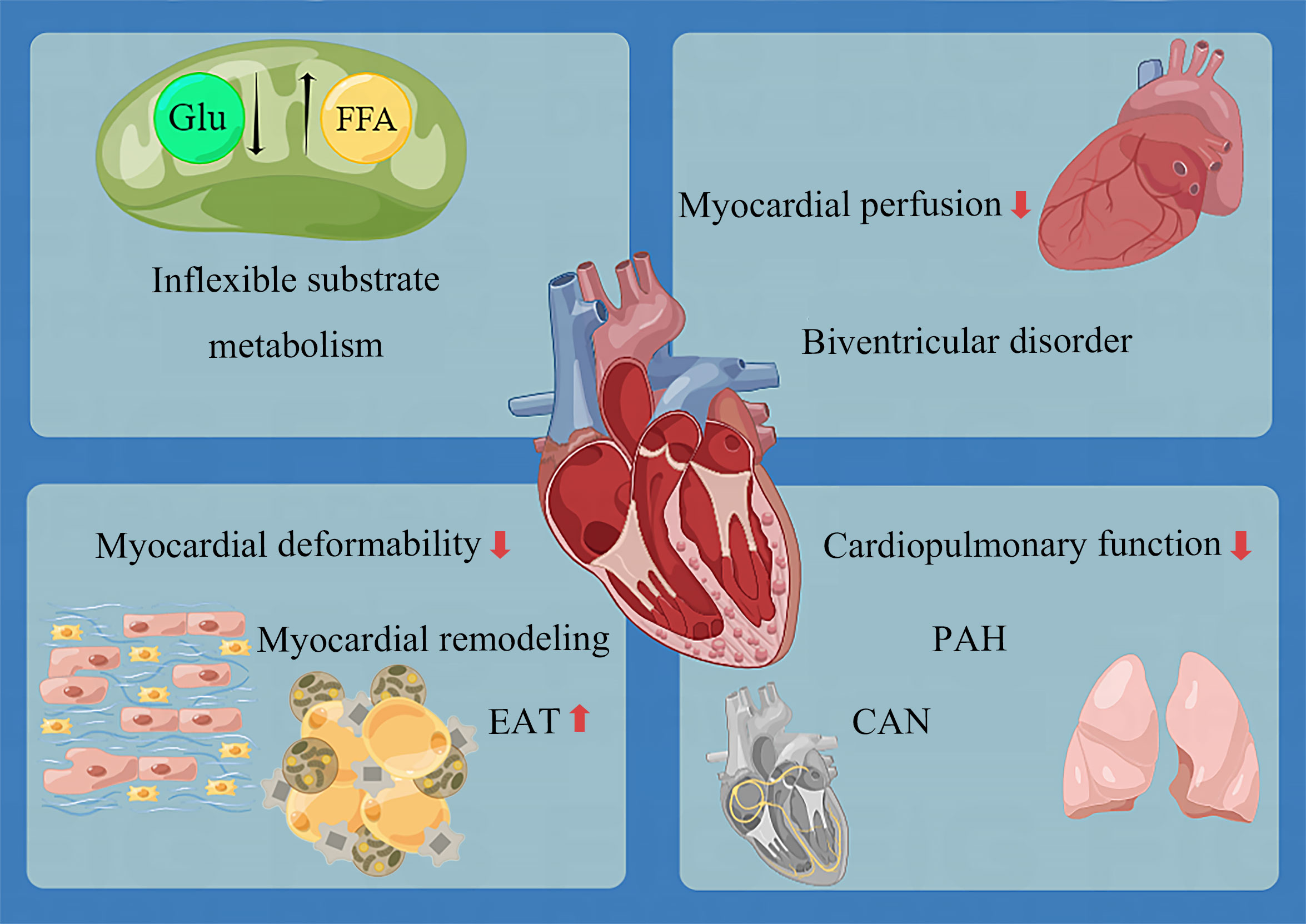
Figure 2 Overview of lesions in the development of DCM. CAN, cardiac autonomic neuropathy; EAT, epicardial adipose tissue; FFA, free fatty acid; Glu, glucose; PAH, pulmonary artery hypertension.
Except these alterations, the pulmonary artery adjacent to the RV is not spared. As early as 2005, the link between T2D and PAH (Pulmonary Artery Hypertension) had been demonstrated by an epidemiological study, showing an increased risk of PAH in diabetic patients independent of smoking, coronary heart disease, hypertension, or congestive heart failure (199). PAH is a disease characterized by pulmonary vascular remodeling, which accelerates the pace of right heart failure by increasing the right ventricular afterload. Insulin resistance, frequently found in diabetic patients with increased pulmonary artery pressure, increases pulmonary stiffness and decreases pulmonary elasticity (48). The prognosis of PAH is poor, and the 10-year survival rate of PAH patients with diabetes is worse (200). DCM may be a cardiopulmonary disease to some extent. Unfortunately, interventions to improve RV function and prognosis in patients with DCM are yet to be studied.
DCM eventually develops into biventricular disorder (48, 201). Although the original research data on T1D are not as detailed as those of T2D, we can find that the cardiac phenotype changes of T1D and T2D are approximately the same. The only noteworthy finding was evidence of cardiac hyperdynamic contraction in T1D but not T2D; this may be attributed to the fact that the course of disease of patients included in T2D research is generally too long, and the opportunity to find this phenomenon is missed. In addition, only sporadic studies have focused on the exact changes in the heart of patients with pre-diabetes. More data accumulation is still needed to understand the impact of pre-diabetes on the heart.
Some scholars believe that DCM affects the whole heart (46). However, we are temporarily unable to decide the situation of right atrium (RA). Although some studies (202–204) found that DM causes RA volume expansion and systolic and diastolic function decline, the subjects were mixed with CAD or hypertension patients, making accurate judgments difficult. However, we confirm that the impact of diabetes on the heart will damage the heart metabolism, structure, function, perfusion, epicardium, and pulmonary vascular, which is comprehensive. In addition to the changes in the heart itself, DM will also cause a series of hematological changes, mainly on the entry of information substances secreted by the heart and other tissues into the plasma.
Another fact is that the pathogenesis of DCM includes CAN (14), making it difficult to separate these two diseases. There is evidence that in T2D patients with normal blood pressure, CAN precedes LVH and diastolic dysfunction, which is a very early change in the heart of DM (205). Furthermore, CAN is gradually aggravated parallel to the progression of cardiomyopathy. Because of the close relationship between nerve and muscle in the heart, the extent of CAN participation in DCM is worth exploring because it is related to the essence of DCM, either nerve-muscle or simple muscle disease.
Diagnostic marker
ECG
Changes in myocardial electrophysiology can be found in DCM patients, such as the prolongation of QT interval, the increase of QT dispersion, and T peak-Tend dispersion. These repolarization abnormalities represent the asynchronous myocardial movement and have been reported to reflect LVDD (130). However, ECG is not specific, making it difficult to diagnose DCM simply by ECG.
Serum marker
Some new markers appear to be promising for use in clinical diagnosis and treatment. Fibrotic markers play a major role. The increase of active MMP-9 and MMP-7 and the decrease of TIMP-1/active MMP-9 ratio have been detected in DM-DD (diastolic dysfunction) (82). It was also found that serum PIP level was negatively correlated with A-Ar (estimated passive diastolic function) in early T2D, suggesting that fibrosis might indeed be the cause of diastolic dysfunction (83). Some other markers have also been studied. GDF-15 is a stress response cytokine that is elevated in asymptomatic DCM and significantly associated with E/e’ (diastolic function index) (80). IGFBP-7 is expected to be a marker for DCM as it increased progressively in patients with DM, DM-DD, and DM-SD (systolic dysfunction) and did not increase in DD patients without diabetes (79). Another study confirmed that NLR was positively correlated with impaired LVDD and was an independent risk factor for subclinical DCM (77), but NLR is also well correlated with other diabetic complications (206). In addition, some crucial biomarkers deserve attention: FABP3, activin A, CT-1, YKL40, galectin-3, and FGF21. They are involved in various pathological mechanisms and have good early diagnostic potential (207).
In recent years, non-coding RNA has been developing rapidly in the field of diagnosis, but many original studies have focused on DM, and there are few studies on DCM markers (208). miRNAs are small non-coding RNAs responsible for post-transcriptional regulation of gene expression. miRNAs can be isolated from tissues and body fluids, and its level can be detected by qPCR, in situ hybridization array, and RNA sequencing (209). Two DCM-related miRNAs (Table 1) have been found. It is striking that one study seems to have found a specific lncRNA marker for DCM. Researchers reported that lncRNA NKILA was up-regulated in the plasma of DCM patients but not in patients with other complications (87). Plasma lncRNA NKILA mRNA levels six months before diagnosis were sufficient to screen DCM in DM patients. Eight years of follow-up research revealed that the expression of the lncRNA NKILA was specifically up-regulated when DCM was present. LncRNA NKILA is a molecule that promotes cardiomyocyte apoptosis, which may be involved in the occurrence and development of DCM and has a bright future in diagnosis or treatment (87).
Some argue that DCM cannot be diagnosed by a single serological marker, so a combination may be needed to solve this problem. Some researchers had begun to endeavor. A study showed that the AUCs of IL-6, TNF-α, and AGEs were 0.905, 0.845, and 0.807, respectively, which could not diagnose DCM. However, the combination of these biomarkers significantly increased AUC to 0.924, with a sensitivity of 84.8% and a specificity of 88.2% (79). The union of TNF-α, AGEs, creatinine, and insulin helped diagnose DM-DD (AUC 0.913, specificity 100%) (79). Nevertheless, the combination of IL-6 and AGEs was found helpful for further differential diagnosis of DM-SD and DM-DD, with an AUC of 0.795 and sensitivity of 90.6%, which was significantly better than that of a separate diagnosis (79).
Echocardiogram
In recent years, LV GLS has become the most commonly used strain value (117) for evaluating LV function in diabetic patients. GLS damage is likely the first ultrasonic sign of preclinical diabetic cardiomyopathy, confirmed in both T1D and T2D (98, 103). In theory, longitudinal myocardial fibers are more prone to ischemia and fibrosis because compensatory ventricular remodeling may increase short-axis function (210). A meta-analysis showed that (126) three-dimensional GLS was 2.4% lower in diabetic patients than in healthy controls, and it is the most obvious indicator of three-dimensional LV systolic strain in all directions. GLS can also be used to exclude DCM. The detection of NPV is up to 0.94 when joined with Gal-3 (81). It should not be ignored that LA strain has changed before LV strain changes, so as an early parameter, it may be more sensitive than LV strain for detecting early DCM (133); this is because LA is a fragile monolayer wall and is sensitive to subtle stimuli (211).
Cardiac magnetic resonance
CMR is accurate for myocardial imaging. Furthermore, one-stop detection of DM heart may be realized in the future due to its excellent differential diagnosis ability of coronary atherosclerotic heart disease and lack of need for a contrast agent (212).
The participation of myocardial microvascular dysfunction in DCM remains uncertain because even ischemia has been excluded, obese patients are inevitably mixed in T2D, and it can also aggravate the adverse effect of diabetes on microvascular function. However, a new study has confirmed that, rather than BMI, HbA1c is the only independent risk factor for myocardial microvascular function in T2D patients (145). Myocardial microvascular dysfunction begins in the early stage of T2D and accumulates with the course extension. A CMR study has reported upslope, Max SI (max signal intensity), and TTM (time to maximum signal intensity) changes, which are indicators of coronary microcirculation impairment in T2D. Multivariate regression analysis shows that TTM and upslope are independently associated with longitudinal PSSR (peak systolic strain rate), suggesting that there might be a mechanical linkage between myocardial perfusion impairment and subclinical myocardial dysfunction in T2D patients (57).
As a result of the application of T1 mapping technology, interstitial fibrosis becomes easy to measure. The more severe interstitial fibrosis is, the worse LV diastolic function becomes (152). ECV can measure dilated ECM (extracellular matrix), so ECV can reflect myocardial fibrosis when there is no myocardial edema or protein deposition (150). The ECV value of diabetic patients has been proven significantly increased, especially those with poor blood glucose control (150). So it may be a sensitive parameter to detect early remodeling before dysfunction. A study between obese adolescents and healthy volunteers found that increased ECV occurred parallel with changes in LV mass and volume, but LV function remained unchanged (151).
As for T1D, researchers introduced a new CMR marker, DRF indice, which can detect ventricular diastolic efficiency and dynamic changes in the diabetic heart between 16-21 years old so that it may be a sensitive marker of cardiac dysfunction in adolescents (141). However, the study of CMR in T1D patients is still lacking.
The diagnostic marker of DCM should be a humoral or structural/functional indicator directly related to its pathogenesis and has good exclusiveness. Unfortunately, no mature auxiliary examination marker has been reliably tested in large clinical trials to diagnose DCM up to this point.
Clinical treatment
hypoglycemic drugs
For DCM treatment, people’s first consideration is whether the hypoglycemic drugs can inhibit the process of impaired cardiac function in DM patients. However, the results are still a little far from satisfaction. Among conventional oral antidiabetic drugs, only gliclazide significantly reduced LVM (213), and their cardiac benefits are tiny (14).
Some scientists focused on DPP4i (sitagliptin, alogliptin, and saxagliptin). All three agents have been evaluated in large clinical trials, but they did not affect the composite primary outcome, which included cardiovascular (CV) mortality, nonfatal MI, and nonfatal stroke (214). As for the influence on DCM, Sitagliptin had a better hypoglycemic effect than voglibose, but it did not improve LV function in 24 weeks of treatment (178). While years later, another study showed that the addition of sitagliptin to the conventional hypoglycemic regimen for 24 months alleviated LVDD (179).
GLP-1RA (liraglutide, exenatide, semaglutide, lixisenatide, and dulaglutide) has attracted much attention as a new star, because it has been shown to have CV protective effects as well as weight loss (215), but its performance is mediocre in DCM. Liraglutide is an injection drug given once a day, whose 3-point MACE (major adverse CV events) benefits has been proved by LEADER trial (216). Two studies have shown that early application of liraglutide (for about six months) improved cardiac function (187, 188), and Liraglutide is more effective than sitagliptin and linagliptin, both in terms of improving diastolic function and proteinuria (217). While another study has shown that the exact duration of liraglutide treatment did not improve cardiac function in patients (189). As for once-weekly Exenatide, who showed no influence on 3-point MACE (218), a study showed that it can improve cardiac function and reduce arterial stiffness, but it is not accompanied by an improvement in exercise capacity (185). However, there is also evidence that exenatide does not affect cardiac function, perfusion, or oxidative metabolism in T2D patients with LVSD (186). Despite Liraglutide seems a little better than Exenatide in CV effect, its frequent injections and increase of heart beats should not be ignored (219). But in any case, these drugs have a positive effect on coronary atherosclerotic events (215). Although not all five GLP-1RA have CV benefits, their CV safety is indisputable, so they are still worthy of consideration in obese DCM patients with mixed atherosclerotic disease.
Of note, SGLT-2i, which includes Dapagliflozin, Empagliflozin and Canagliflozin, have all been proved to help decrease the rate of CV events or heart failure and even death (220–222). When it comes to DCM, Dapagliflozin can prevent and reverse the development of ventricular remodeling (180, 181). The efficacy of Empagliflozin is diverse. 12 weeks’ empagliflozin treatment not only enhances cardiac function, attenuates adverse remodeling, but also improves myocardial energy metabolism, which is not found in the other two (184). And another study showed that the earlier the intervention implements, the better the improvement in left ventricular diastolic and systolic function will be (183). There is a study which has shown that 3 months of canagliflozin treatment can improve left ventricular diastolic function, but it should be noted that the study population was mixed with ischemic heart disease patients, limiting the reference value (182).
Besides, both Dapagliflozin and Canagliflozin showed significant renal benefits (220, 221). It is highly likely that DCM is associated with renal injury because microalbuminuria is associated with early DCM as mentioned above, which makes these two drugs worthy of further investigation. By the way, a meta-analysis showed that the efficacy of SGLT-2i was sex specific, which reduces MACE better in men than in women. However, GLP-1RA did not show this difference (223). In general, SGLT-2i is more promising than GLP-1RA in DCM. Because the former has better performance in preventing heart failure and improving renal outcomes while similar to the latter in atherosclerotic benefits, and is injection-independent (224). In addition to this, GLP-1RA on top of SGLT-2i is a hopeful direction. Preliminary studies have shown that the combination of liraglutide and empagliflozin for 12 months significantly improves effective myocardial work and cardiac function in patients with type 2 diabetes mellitus in high CV risk. However, similar work needs to be done in patients excluding coronary heart disease and hypertension to determine whether the effect persists.
Cardiovascular drugs
Although conventional cardiovascular drugs (ACEI, ARB, and β blocker) are widely used in the diabetic population, there is a lack of evidence regarding the clinical efficacy of DCM. But new heart failure drugs are likely to play an important role in DCM induced HFrEF in the near future. Large studies have shown that patients with combined use of new drugs (ARNi, β-blocker, MRA and SGLT2i) can delay 2.7 years (80 years) to 8.3 years (55 years) without death from cardiovascular disease or first hospitalization for heart failure, and increase life expectancy by 1.4 years (80 years) to 6.3 years (55 years), compared with conventional therapies (225). Notably, three large cohorts each had more than 30% of patients with DM, heralding a promising future for these agents in DM.
Sacubitril/valsartan inhibits neprilysin through its active metabolite of sacubitril, and at the same time blocks angiotensin II type 1 receptor through valsartan, which plays an anti-heart failure role. Sacubitril/valsartan reduced NT-proBNP to a greater extent than valsartan at 12 weeks and was well tolerated in patients with HFpEF (226). And the benefit of Sacubitril/valsartan is across all age groups from 18 to 96 years old (227). However, in patients with HFpEF (EF ≥45%), sacubitril/valsartan did not significantly reduce heart failure hospitalizations or cardiovascular mortality (228). Unexpectedly, the investigators also found a beneficial effect of sacubitril/valsartan on glycemic control. In patients with diabetes and HFrEF enrolled in the PARADIGM-HF study, long-term reductions in HBA1C and delayed time to initiation of oral glucose-lowering therapy were observed (229).
Vericiguat, approved by the U.S. FDA in 2021, is the first soluble guanylate cyclase (sGC) agonist for the treatment of patients with symptomatic HFrEF. Patients with chronic HFrEF who received vericiguat had a lower incidence of cardiovascular death or hospitalization for heart failure than those who received placebo (230). However, in patients with decompensated HFpEF, vericiguat treatment for 24 weeks did not improve activity tolerance and quality of life which were measured by KCCQ (231). The safety and tolerability profile of vericiguat were good when sacubitril/valsartan was used together for at least 3 months, suggesting that the combination may provide additional benefit (232).
Elevated resting heart rate is a risk factor for poor outcomes in heart failure (233). when heart rate is ≥70 beats/min, the risk of cardiovascular outcomes increases in patients with CAD and LVSD (234). This sparked scientists’ interest in Ivabradine, a drug that lowers the heart rate. Ivabradine was shown to be effective and safe in patients with HFrEF regardless of diabetes status, and significantly reduced the primary composite endpoint (PCE) in both diabetic and non-diabetic patients (235). However, a randomized, double-blind, placebo-controlled trial found that heart rate reduction with ivabradine did not improve outcomes in HFpEF patients (236).
Omecamtiv mecarbil is the first potent cardiac myosin activator that can specifically activate the cardiac myosin S1 structure. A preclinical report has shown that it increases myocardial contractility without increasing myocardial oxygen consumption (237), and in clinical trials, it improved the composite rate of heart failure events or death from cardiovascular causes (238). The improvement of cardiac function by Omecamtiv was also very significant. Omecamtiv treatment for 20 weeks directly improved LV myocardial deformation in patients with HFrEF, whch means improved GLS by about 0.8%, and also improved GCS, while reducing heart rate and NT-proBNP concentrations, significantly increasing systolic ejection time, stroke volume, and reversing LV remodeling have been reported (239). Each 1% improvement in GLS is statistically associated with a 24% reduction in the risk of HF hospitalization and death (240). What’s more, omecamtiv also results in a small reduction in heart rate (241). Unfortunately, omecamtiv did not improve exercise capacity compared with placebo over a period of 20 weeks in patients with chronic HFrEF (242).
Other than those, some non-classical cardiovascular drugs appear to show some different potential. The critical role of inflammatory cytokines in developing cardiovascular disease in individuals with and without diabetes is well established. A study has shown that trimetazidine treatment could significantly improve cardiac function and physical tolerance, accompanied by a reduction in inflammation (193), but it could not explain if there is a link between inflammation and the outcome. Another study found that sildenafil could control DCM progression by targeting IL-8 at the systemic and cellular levels (192). A previous study had also shown that sildenafil could achieve its anti-remodeling effect by reducing LV concentric hypertrophy in patients with early DCM (191). In addition, an aldosterone receptor antagonist, Spironolactone, has been proven to be failed to improve DCM’s changes in myocardial structure and diastolic function (243).
According to the existing drug research results and HF guidelines (244, 245), we summarized a therapeutic algorithm (Figure 3). To date, neither the long-term survival problem caused by HFpEF nor the limitation of exercise in HF patients can be improved by any drug. To make matters worse, no drug independently treats DCM as a primary role.
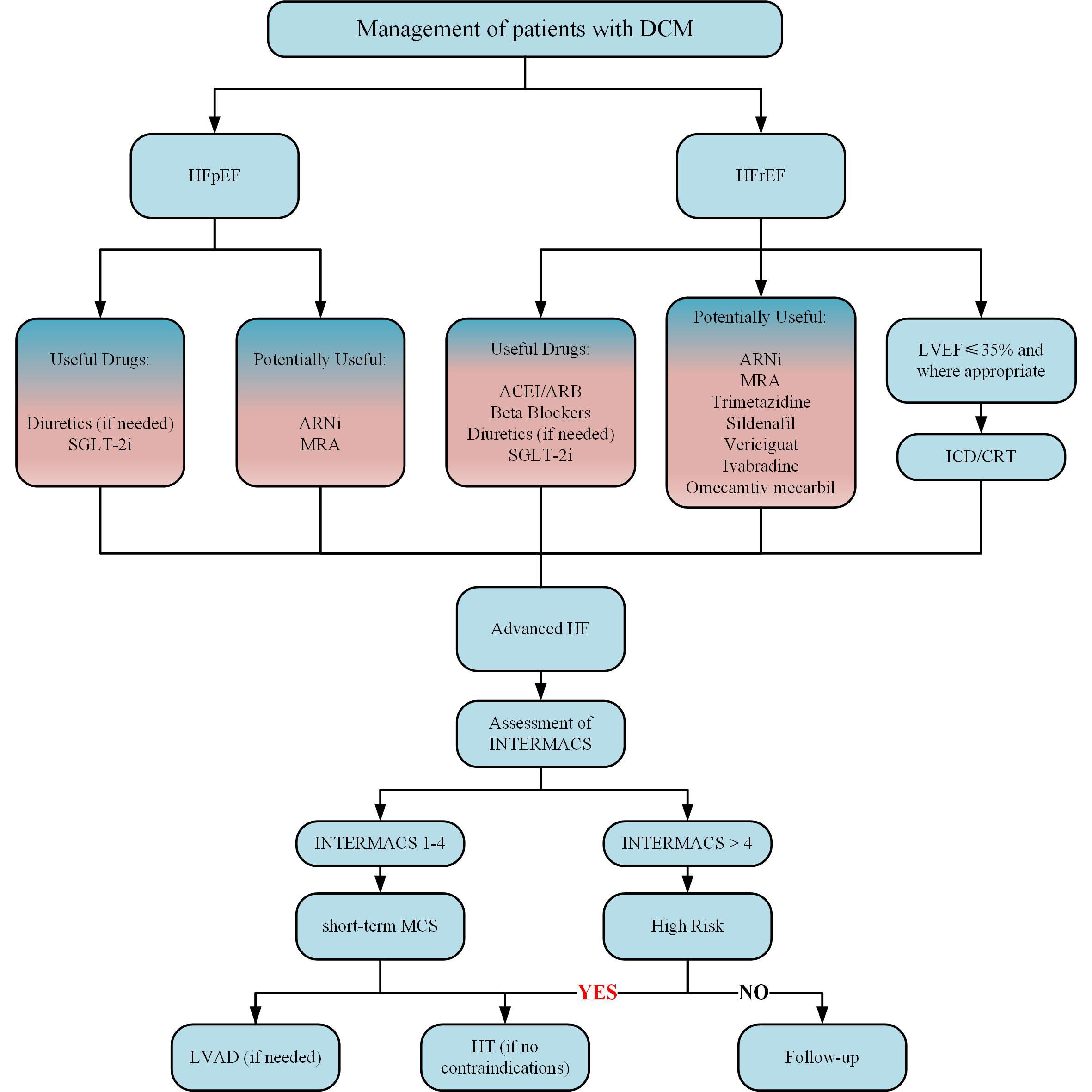
Figure 3 Treatment algorithm of DCM. ACEI, indicates angiotensin-converting enzyme inhibitor; ARB, angiotensin receptor blocker; ARNi, angiotensin receptor-neprilysin inhibitor; CRT, cardiac resynchronization therapy; HF, heart failure; HFrEF, heart failure with reduced ejection fraction; HFpEF, heart failure with preserved ejection fraction; HT, heart transplant; ICD, implantable cardioverter-defibrillator; INTERMACS, Interagency Registry for Mechanically Assisted Circulatory Support; LVAD, left ventricular assist device; MCS, mechanical circulatory support; MRA, mineralocorticoid receptor antagonist; SGLT2i, sodium-glucose cotransporter 2 inhibitor.
Conclusion
As a complication of DM, DCM has been studied for 50 years. The vast accumulation of knowledge has painted the outline of DCM so humans can overlook the panorama of clinical phenotypes. More observational clinical studies are needed to continuously improve the undiscovered corners of DCM, correct previous studies’ results, and explore indicators that can be used for accurate diagnosis. Screening criteria still need to be further defined, which is vital for finding high-risk populations of DCM. Nevertheless, it is gratifying that the diagnostic criteria have been established, which allows the real focus on implementing clinical intervention studies of DCM, even if the threshold is high. The accumulation of DCM research is significant. When achieving development of safe and effective diabetic cardiomyopathy drugs, whether combined with diabetes will affect the classification, diagnosis, and treatment of HF and change the dilemma that the prognosis of HF patients with diabetes is worse than that of HF patients alone. And the dawn will eventually come.
Author contributions
XZ: Writing- Original draft preparation. SL: Writing- Reviewing and Editing. GF: Conceptualization, Methodology. BW: Supervision. XW: Software, Visualization. SW: Funding acquisition. PP, QY, SD and JL: Data curation. YC: Methodology. All authors contributed to the article and approved the submitted version.
Funding
This work is supported by National Administration of Traditional Chinese Medicine (No: ZYYCXTD-D-202207, and National Chinese Medicine Education Letter [2022] No.75).
Conflict of interest
The authors declare that the research was conducted in the absence of any commercial or financial relationships that could be construed as a potential conflict of interest.
Publisher’s note
All claims expressed in this article are solely those of the authors and do not necessarily represent those of their affiliated organizations, or those of the publisher, the editors and the reviewers. Any product that may be evaluated in this article, or claim that may be made by its manufacturer, is not guaranteed or endorsed by the publisher.
References
1. World Health Organization G. The top 10 causes of death [Updated 201]. WHO (2020) Available at: https://www.who.int/news-room/fact-sheets/detail/the-top-10-causes-of-death.
2. Kannel WB, McGee DL. Diabetes and cardiovascular disease. Framingham Study. JAMA (1979) 241(19):2035–8. doi: 10.1001/jama.241.19.2035
3. Gagnum V, Stene LC, Jenssen TG, Berteussen LM, Sandvik L, Joner G, et al. Causes of death in childhood-onset type 1 diabetes: Long-term follow-up. Diabetic Med J Br Diabetic Assoc (2017) 34(1):56–63. doi: 10.1111/dme.13114
4. Di Angelantonio E, Kaptoge S, Wormser D, Willeit P, Butterworth AS, Bansal N, et al. Association of cardiometabolic multimorbidity with mortality. Jama (2015) 314(1):52–60. doi: 10.1001/jama.2015.7008
5. Levelt E, Pavlides M, Banerjee R, Mahmod M, Kelly C, Sellwood J, et al. Ectopic and visceral fat deposition in lean and obese patients with type 2 diabetes. J Am Coll Cardiol (2016) 68(1):53–63. doi: 10.1016/j.jacc.2016.03.597
6. Lundbaek K. Diabetic angiopathy: A specific vascular disease. Lancet (London England) (1954) 266(6808):377–9. doi: 10.1016/s0140-6736(54)90924-1
7. Bertoni AG, Tsai A, Kasper EK, Brancati FL. Diabetes and idiopathic cardiomyopathy: A nationwide case-control study. Diabetes Care (2003) 26(10):2791–5. doi: 10.2337/diacare.26.10.2791
8. Paolillo S, Marsico F, Prastaro M, Renga F, Esposito L, De Martino F, et al. Diabetic cardiomyopathy: Definition, diagnosis, and therapeutic implications. Heart Fail Clin (2019) 15(3):341–7. doi: 10.1016/j.hfc.2019.02.003
9. Westermeier F, Riquelme JA, Pavez M, Garrido V, Díaz A, Verdejo HE, et al. New molecular insights of insulin in diabetic cardiomyopathy. Front Physiol (2016) 7:125. doi: 10.3389/fphys.2016.00125
10. Falcao-Pires I, Leite-Moreira AF. Diabetic cardiomyopathy: Understanding the molecular and cellular basis to progress in diagnosis and treatment. Heart Fail Rev (2012) 17(3):325–44. doi: 10.1007/s10741-011-9257-z
11. Goyal BR, Mehta AA. Diabetic cardiomyopathy: Pathophysiological mechanisms and cardiac dysfuntion. Hum Exp Toxicol (2013) 32(6):571–90. doi: 10.1177/0960327112450885
12. Kumric M, Ticinovic Kurir T, Borovac JA, Bozic J. Role of novel biomarkers in diabetic cardiomyopathy. World J Diabetes (2021) 12(6):685–705. doi: 10.4239/wjd.v12.i6.685
13. Jia GH, DeMarco VG, Sowers JR. Insulin resistance and hyperinsulinaemia in diabetic cardiomyopathy. Nat Rev Endocrinol (2016) 12(3):144–53. doi: 10.1038/nrendo.2015.216
14. Murtaza G, Virk HUH, Khalid M, Lavie CJ, Ventura H, Mukherjee D, et al. Diabetic cardiomyopathy - a comprehensive updated review. Prog Cardiovasc Dis (2019) 62(4):315–26. doi: 10.1016/j.pcad.2019.03.003
15. Chavali V, Tyagi SC, Mishra PK. Predictors and prevention of diabetic cardiomyopathy. Diabetes Metab syndrome Obes Targets Ther (2013) 6:151–60. doi: 10.2147/DMSO.S30968
16. Mordi IR. Non-invasive imaging in diabetic cardiomyopathy. J Cardiovasc Dev Dis (2019) 6(2):18. doi: 10.3390/jcdd6020018
17. Lee WS, Kim J. Diabetic cardiomyopathy: Where we are and where we are going. Korean J Intern Med (2017) 32(3):404–21. doi: 10.3904/kjim.2016.208
18. Fang ZY, Prins JB, Marwick TH. Diabetic cardiomyopathy: Evidence, mechanisms, and therapeutic implications. Endocr Rev (2004) 25(4):543–67. doi: 10.1210/er.2003-0012
19. Seferović PM, Milinković I, Ristić AD, Seferović Mitrović JP, Lalić K, Jotić A, et al. Diabetic cardiomyopathy: Ongoing controversies in 2012. Herz (2012) 37(8):880–6. doi: 10.1007/s00059-012-3720-z
20. Marfella R, Sardu C, Mansueto G, Napoli C, Paolisso G. Evidence for human diabetic cardiomyopathy. Acta Diabetol (2021) 58(8):983–8. doi: 10.1007/s00592-021-01705-x
21. Fuentes-Antrás J, Picatoste B, Ramírez E, Egido J, Tuñón J, Lorenzo Ó. Targeting metabolic disturbance in the diabetic heart. Cardiovasc Diabetol (2015) 14:17. doi: 10.1186/s12933-015-0173-8
22. Adameova A, Dhalla NS. Role of microangiopathy in diabetic cardiomyopathy. Heart Failure Rev (2014) 19(1):25–33. doi: 10.1007/s10741-013-9378-7
23. Dhalla NS, Liu X, Panagia V, Takeda N. Subcellular remodeling and heart dysfunction in chronic diabetes. Cardiovasc Res (1998) 40(2):239–47. doi: 10.1016/S0008-6363(98)00186-2
24. Mandavia CH, Pulakat L, DeMarco V, Sowers JR. Over-nutrition and metabolic cardiomyopathy. Metabolism: Clin Exp (2012) 61(9):1205–10. doi: 10.1016/j.metabol.2012.02.013
25. Wright JJ, Kim J, Buchanan J, Boudina S, Sena S, Bakirtzi K, et al. Mechanisms for increased myocardial fatty acid utilization following short-term high-fat feeding. Cardiovasc Res (2009) 82(2):351–60. doi: 10.1093/cvr/cvp017
26. Boudina S, Abel ED. Diabetic cardiomyopathy revisited. Circulation (2007) 115(25):3213–23. doi: 10.1161/circulationaha.106.679597
27. Buchanan J, Mazumder PK, Hu P, Chakrabarti G, Roberts MW, Yun UJ, et al. Reduced cardiac efficiency and altered substrate metabolism precedes the onset of hyperglycemia and contractile dysfunction in two mouse models of insulin resistance and obesity. Endocrinology (2005) 146(12):5341–9. doi: 10.1210/en.2005-0938
28. Cook SA, Varela-Carver A, Mongillo M, Kleinert C, Khan MT, Leccisotti L, et al. Abnormal myocardial insulin signalling in type 2 diabetes and left-ventricular dysfunction. Eur Heart J (2010) 31(1):100–11. doi: 10.1093/eurheartj/ehp396
29. Coort SL, Hasselbaink DM, Koonen DP, Willems J, Coumans WA, Chabowski A, et al. Enhanced sarcolemmal Fat/Cd36 content and triacylglycerol storage in cardiac myocytes from obese zucker rats. Diabetes (2004) 53(7):1655–63. doi: 10.2337/diabetes.53.7.1655
30. Diamant M, Lamb HJ, Groeneveld Y, Endert EL, Smit JWA, Bax JJ, et al. Diastolic dysfunction is associated with altered myocardial metabolism in asymptomatic normotensive patients with well-controlled type 2 diabetes mellitus. J Am Coll Cardiol (2003) 42(2):328–35. doi: 10.1016/S0735-1097(03)00625-9
31. Atar AI, Altuner TK, Bozbas H, Korkmaz ME. Coronary flow reserve in patients with diabetes mellitus and prediabetes. Echocardiography (Mount Kisco NY) (2012) 29(6):634–40. doi: 10.1111/j.1540-8175.2012.01668.x
32. Duncan JG. Mitochondrial dysfunction in diabetic cardiomyopathy. Biochim Biophys Acta (2011) 1813(7):1351–9. doi: 10.1016/j.bbamcr.2011.01.014
33. Wende AR, Symons JD, Abel ED. Mechanisms of lipotoxicity in the cardiovascular system. Curr Hypertens Rep (2012) 14(6):517–31. doi: 10.1007/s11906-012-0307-2
34. Anderson EJ, Kypson AP, Rodriguez E, Anderson CA, Lehr EJ, Neufer PD. Substrate-specific derangements in mitochondrial metabolism and redox balance in the atrium of the type 2 diabetic human heart. J Am Coll Cardiol (2009) 54(20):1891–8. doi: 10.1016/j.jacc.2009.07.031
35. Boudina S, Bugger H, Sena S, O'Neill BT, Zaha VG, Ilkun O, et al. Contribution of impaired myocardial insulin signaling to mitochondrial dysfunction and oxidative stress in the heart. Circulation (2009) 119(9):1272–83. doi: 10.1161/circulationaha.108.792101
36. Boudina S, Abel ED. Mitochondrial uncoupling: A key contributor to reduced cardiac efficiency in diabetes. Physiol (Bethesda Md) (2006) 21:250–8. doi: 10.1152/physiol.00008.2006
37. Bidasee KR, Nallani K, Yu Y, Cocklin RR, Zhang Y, Wang M, et al. Chronic diabetes increases advanced glycation end products on cardiac ryanodine Receptors/Calcium-release channels. Diabetes (2003) 52(7):1825–36. doi: 10.2337/diabetes.52.7.1825
38. Bidasee KR, Zhang Y, Shao CH, Wang M, Patel KP, Dincer UD, et al. Diabetes increases formation of advanced glycation end products on Sarco(Endo)Plasmic reticulum Ca2+-atpase. Diabetes (2004) 53(2):463–73. doi: 10.2337/diabetes.53.2.463
39. Anderson EJ, Rodriguez E, Anderson CA, Thayne K, Chitwood WR, Kypson AP. Increased propensity for cell death in diabetic human heart is mediated by mitochondrial-dependent pathways. Am J Physiol Heart Circ Physiol (2011) 300(1):H118–24. doi: 10.1152/ajpheart.00932.2010
40. Malek V, Gaikwad AB. Telmisartan and thiorphan combination treatment attenuates fibrosis and apoptosis in preventing diabetic cardiomyopathy. Cardiovasc Res (2019) 115(2):373–84. doi: 10.1093/cvr/cvy226
41. van Heerebeek L, Hamdani N, Handoko ML, Falcao-Pires I, Musters RJ, Kupreishvili K, et al. Diastolic stiffness of the failing diabetic heart: Importance of fibrosis, advanced glycation end products, and myocyte resting tension. Circulation (2008) 117(1):43–51. doi: 10.1161/CIRCULATIONAHA.107.728550
42. Grubić Rotkvić P, Planinić Z, Liberati Pršo AM, Šikić J, Galić E, Rotkvić L. The mystery of diabetic cardiomyopathy: From early concepts and underlying mechanisms to novel therapeutic possibilities. Int J Mol Sci (2021) 22(11):5973. doi: 10.3390/ijms22115973
43. Gilca GE, Stefanescu G, Badulescu O, Tanase DM, Bararu I, Ciocoiu M. Diabetic cardiomyopathy: Current approach and potential diagnostic and therapeutic targets. J Diabetes Res (2017) 2017:1310265. doi: 10.1155/2017/1310265
44. Maisch B, Alter P, Pankuweit S. Diabetic cardiomyopathy–fact or fiction? Herz (2011) 36(2):102–15. doi: 10.1007/s00059-011-3429-4
45. Tillquist MN, Maddox TM. Update on diabetic cardiomyopathy: Inches forward, miles to go. Curr Diabetes Rep (2012) 12(3):305–13. doi: 10.1007/s11892-012-0274-7
46. Triposkiadis F, Xanthopoulos A, Bargiota A, Kitai T, Katsiki N, Farmakis D, et al. Diabetes mellitus and heart failure. J Clin Med (2021) 10(16):3682. doi: 10.3390/jcm10163682
47. From AM, Scott CG, Chen HH. The development of heart failure in patients with diabetes mellitus and pre-clinical diastolic dysfunction a population-based study. J Am Coll Cardiol (2010) 55(4):300–5. doi: 10.1016/j.jacc.2009.12.003
48. Kang Y, Wang S, Huang J, Cai L, Keller BB. Right ventricular dysfunction and remodeling in diabetic cardiomyopathy. Am J Physiol Heart Circ Physiol (2019) 316(1):H113–H22. doi: 10.1152/ajpheart.00440.2018
49. Tadic M, Cuspidi C, Calicchio F, Grassi G, Mancia G. Diabetic cardiomyopathy: How can cardiac magnetic resonance help? Acta Diabetol (2020) 57(9):1027–34. doi: 10.1007/s00592-020-01528-2
50. Cao Y, Zeng W, Cui Y, Kong X, Wang M, Yu J, et al. Increased myocardial extracellular volume assessed by cardiovascular magnetic resonance T1 mapping and its determinants in type 2 diabetes mellitus patients with normal myocardial systolic strain. Cardiovasc Diabetol (2018) 17(1):7. doi: 10.1186/s12933-017-0651-2
51. Zeng M, Qiao Y, Wen Z, Liu J, Xiao E, Tan C, et al. The association between diffuse myocardial fibrosis on cardiac magnetic resonance T1 mapping and myocardial dysfunction in diabetic rabbits. Sci Rep (2017) 7:44937. doi: 10.1038/srep44937
52. Ng ACT, Prevedello F, Dolci G, Roos CJ, Djaberi R, Bertini M, et al. Impact of diabetes and increasing body mass index category on left ventricular systolic and diastolic function. J Am Soc Echocardiography (2018) 31(8):916–25. doi: 10.1016/j.echo.2018.02.012
53. Fonseca CG, Dissanayake AM, Doughty RN, Whalley GA, Gamble GD, Cowan BR, et al. Three-dimensional assessment of left ventricular systolic strain in patients with type 2 diabetes mellitus, diastolic dysfunction, and normal ejection fraction. Am J Cardiol (2004) 94(11):1391–5. doi: 10.1016/j.amjcard.2004.07.143
54. Kosmala W, Przewlocka-Kosmala M, Mazurek W. Subclinical right ventricular dysfunction in diabetes mellitus–an ultrasonic Strain/Strain rate study. Diabetic Med J Br Diabetic Assoc (2007) 24(6):656–63. doi: 10.1111/j.1464-5491.2007.02101.x
55. Vukomanovic V, Suzic-Lazic J, Celic V, Cuspidi C, Petrovic T, Grassi G, et al. The relationship between functional capacity and left ventricular strain in patients with uncomplicated type 2 diabetes. J Hypertension (2019) 37(9):1871–6. doi: 10.1097/HJH.0000000000002125
56. Nakai H, Takeuchi M, Nishikage T, Lang RM, Otsuji Y. Subclinical left ventricular dysfunction in asymptomatic diabetic patients assessed by two-dimensional speckle tracking echocardiography: Correlation with diabetic duration. Eur J Echocardiogr (2009) 10(8):926–32. doi: 10.1093/ejechocard/jep097
57. Liu X, Yang ZG, Gao Y, Xie LJ, Jiang L, Hu BY, et al. Left ventricular subclinical myocardial dysfunction in uncomplicated type 2 diabetes mellitus is associated with impaired myocardial perfusion: A contrast-enhanced cardiovascular magnetic resonance study. Cardiovasc Diabetol (2018) 17(1):139. doi: 10.1186/s12933-018-0782-0
58. Korosoglou G, Humpert PM, Ahrens J, Oikonomou D, Osman NF, Gitsioudis G, et al. Left ventricular diastolic function in type 2 diabetes mellitus is associated with myocardial triglyceride content but not with impaired myocardial perfusion reserve. J Magnetic Resonance Imaging JMRI (2012) 35(4):804–11. doi: 10.1002/jmri.22879
59. Milwidsky A, Maor E, Kivity S, Berkovitch A, Zekry SB, Tenenbaum A, et al. Impaired fasting glucose and left ventricular diastolic dysfunction in middle-age adults: A retrospective cross-sectional analysis of 2971 subjects. Cardiovasc Diabetol (2015) 14:119. doi: 10.1186/s12933-015-0282-4
60. Berceanu M, Mirea O, Donoiu I, Militaru C, Săftoiu A, Istrătoaie O. Myocardial function assessed by multi-layered two-dimensional speckle tracking analysis in asymptomatic young subjects with diabetes mellitus type 1. Cardiology (2020) 145(2):80–7. doi: 10.1159/000504532
61. Khattab AA, Soliman MA. Biventricular function and glycemic load in type 1 diabetic children: Doppler tissue-imaging study. Pediatr Cardiol (2015) 36(2):423–31. doi: 10.1007/s00246-014-1030-3
62. Hensel KO, Grimmer F, Roskopf M, Jenke AC, Wirth S, Heusch A. Subclinical alterations of cardiac mechanics present early in the course of pediatric type 1 diabetes mellitus: A prospective blinded speckle tracking stress echocardiography study. J Diabetes Res (2016) 2016:2583747. doi: 10.1155/2016/2583747
63. Karamitsos TD, Karvounis HI, Dalamanga EG, Papadopoulos CE, Didangellos TP, Karamitsos DT, et al. Early diastolic impairment of diabetic heart: The significance of right ventricle. Int J Cardiol (2007) 114(2):218–23. doi: 10.1016/j.ijcard.2006.02.003
64. Seferovic PM, Paulus WJ. Clinical diabetic cardiomyopathy: A two-faced disease with restrictive and dilated phenotypes. Eur Heart J (2015) 36(27):1718–27, 27a-27c. doi: 10.1093/eurheartj/ehv134
65. Hensel KO. Non-ischemic diabetic cardiomyopathy may initially exhibit a transient subclinical phase of hyperdynamic myocardial performance. Med Hypotheses (2016) 94:7–10. doi: 10.1016/j.mehy.2016.06.002
66. Gøtzsche O, Sørensen K, McIntyre B, Henningsen P. Reduced left ventricular afterload and increased contractility in children with insulin-dependent diabetes mellitus: An m-mode and Doppler-echocardiographic evaluation of left ventricular diastolic and systolic function. Pediatr Cardiol (1991) 12(2):69–73. doi: 10.1007/BF02238405
67. Gøtzsche O, Darwish A, Gøtzsche L, Hansen LP, Sørensen KE. Incipient cardiomyopathy in young insulin-dependent diabetic patients: A seven-year prospective Doppler echocardiographic study. Diabetic Med J Br Diabetic Assoc (1996) 13(9):834–40. doi: 10.1002/(SICI)1096-9136(199609)13:9<834::AID-DIA225>3.0.CO;2-M
68. Christiansen EH, Mølgaard H, Christensen PD, Sørensen KE, Christensen CK, Mogensen CE. Increased left ventricular systolic function in insulin dependent diabetic patients with normal albumin excretion. Eur Heart J (1998) 19(11):1735–9. doi: 10.1053/euhj.1998.1090
69. Chung J, Abraszewski P, Yu X, Liu W, Krainik AJ, Ashford M, et al. Paradoxical increase in ventricular torsion and systolic torsion rate in type I diabetic patients under tight glycemic control. J Am Coll Cardiol (2006) 47(2):384–90. doi: 10.1016/j.jacc.2005.08.061
70. Shivu GN, Abozguia K, Phan TT, Ahmed I, Weaver R, Narendran P, et al. Increased left ventricular torsion in uncomplicated type 1 diabetic patients: The role of coronary microvascular function. Diabetes Care (2009) 32(9):1710–2. doi: 10.2337/dc09-0408
71. Gil KE, Pawlak A, Gil RJ, Frontczak-Baniewicz M, Bil J. The role of invasive diagnostics and its impact on the treatment of dilated cardiomyopathy: A systematic review. Adv Med Sci (2016) 61(2):331–43. doi: 10.1016/j.advms.2016.07.001
72. Yazici D, Yavuz DG, Toprak A, Deyneli O, Akalin S. Impaired diastolic function and elevated nt-probnp levels in type 1 diabetic patients without overt cardiovascular disease. Acta Diabetol (2013) 50(2):155–61. doi: 10.1007/s00592-010-0235-z
73. Betti I, Castelli G, Barchielli A, Beligni C, Boscherini V, De Luca L, et al. The role of n-terminal pro-brain natriuretic peptide and echocardiography for screening asymptomatic left ventricular dysfunction in a population at high risk for heart failure. the probe-hf study. J Cardiac Failure (2009) 15(5):377–84. doi: 10.1016/j.cardfail.2008.12.002
74. Kristensen SL, Mogensen UM, Jhund PS, Petrie MC, Preiss D, Win S, et al. Clinical and echocardiographic characteristics and cardiovascular outcomes according to diabetes status in patients with heart failure and preserved ejection fraction: A report from the I-preserve trial (Irbesartan in heart failure with preserved ejection fraction). Circulation (2017) 135(8):724–35. doi: 10.1161/CIRCULATIONAHA.116.024593
75. Romano S, Di Mauro M, Fratini S, Guarracini L, Guarracini F, Poccia G, et al. Early diagnosis of left ventricular diastolic dysfunction in diabetic patients: A possible role for natriuretic peptides. Cardiovasc Diabetol (2010) 9:89. doi: 10.1186/1475-2840-9-89
76. Dencker M, Stagmo M, Dorkhan M. Relationship between natriuretic peptides and echocardiography parameters in patients with poorly regulated type 2 diabetes. Vasc Health Risk Manage (2010) 6:373–82. doi: 10.2147/VHRM.S9332
77. Huang X, Qin Z, Xu M, Zhang F, Jiang X, Hua F, et al. Neutrophil: Lymphocyte ratio is positively associated with subclinical diabetic cardiomyopathy. BMC Endocr Disord (2020) 20(1):99. doi: 10.1186/s12902-020-00571-y
78. Swoboda PP, McDiarmid AK, Erhayiem B, Ripley DP, Dobson LE, Garg P, et al. Diabetes mellitus, microalbuminuria, and subclinical cardiac disease: Identification and monitoring of individuals at risk of heart failure. J Am Heart Assoc (2017) 6(7):e005539. doi: 10.1161/jaha.117.005539
79. Abdelrahman AH, Salama II, Salama SI, Elmosalami DM, Ibrahim MH, Hassan EM, et al. Role of some serum biomarkers in the early detection of diabetic cardiomyopathy. Future Sci OA (2021) 7(5):Fso682. doi: 10.2144/fsoa-2020-0184
80. Dominguez-Rodriguez A, Abreu-Gonzalez P, Avanzas P. Usefulness of growth differentiation factor-15 levels to predict diabetic cardiomyopathy in asymptomatic patients with type 2 diabetes mellitus. Am J Cardiol (2014) 114(6):890–4. doi: 10.1016/j.amjcard.2014.06.020
81. Flores-Ramírez R, Azpiri-López JR, González-González JG, Ordaz-Farías A, González-Carrillo LE, Carrizales-Sepúlveda EF, et al. Global longitudinal strain as a biomarker in diabetic cardiomyopathy. a comparative study with gal-3 in patients with preserved ejection fraction. Archivos cardiologia Mexico (2017) 87(4):278–85. doi: 10.1016/j.acmx.2016.06.002
82. Ban CR, Twigg SM, Franjic B, Brooks BA, Celermajer D, Yue DK, et al. Serum mmp-7 is increased in diabetic renal disease and diabetic diastolic dysfunction. Diabetes Res Clin Pract (2010) 87(3):335–41. doi: 10.1016/j.diabres.2010.01.004
83. Ihm S-H, Youn H-J, Shin D-I, Jang S-W, Park C-S, Kim PJ, et al. Serum carboxy-terminal propeptide of type I procollagen (Pip) is a marker of diastolic dysfunction in patients with early type 2 diabetes mellitus. Int J Cardiol (2007) 122(3):e36–e8. doi: 10.1016/j.ijcard.2007.07.057
84. Gamella-Pozuelo L, Fuentes-Calvo I, Gómez-Marcos MA, Recio-Rodriguez JI, Agudo-Conde C, Fernández-Martín JL, et al. Plasma cardiotrophin-1 as a marker of hypertension and diabetes-induced target organ damage and cardiovascular risk. Medicine (2015) 94(30):e1218. doi: 10.1097/MD.0000000000001218
85. Lu H, Buchan RJ, Cook SA. Microrna-223 regulates Glut4 expression and cardiomyocyte glucose metabolism. Cardiovasc Res (2010) 86(3):410–20. doi: 10.1093/cvr/cvq010
86. Tao L, Huang X, Xu M, Qin Z, Zhang F, Hua F, et al. Value of circulating mirna-21 in the diagnosis of subclinical diabetic cardiomyopathy. Mol Cell Endocrinol (2020) 518:110944. doi: 10.1016/j.mce.2020.110944
87. Li Q, Li P, Su J, Liu S, Yang X, Yang Y, et al. Lncrna nkila was upregulated in diabetic cardiomyopathy with early prediction values. Exp Ther Med (2019) 18(2):1221–5. doi: 10.3892/etm.2019.7671
88. Chambers MA, Shaibi GQ, Kapadia CR, Vander Wyst KB, Campos A, Pimentel J, et al. Epicardial adipose thickness in youth with type 1 diabetes. Pediatr Diabetes (2019) 20(7):941–5. doi: 10.1111/pedi.12893
89. Yazıcı D, Özben B, Yavuz D, Deyneli O, Aydın H, Tarcin Ö, et al. Epicardial adipose tissue thickness in type 1 diabetic patients. Endocrine (2011) 40(2):250–5. doi: 10.1007/s12020-011-9478-x
90. AsoĞlu R, Özdemİr M, AladaĞ N, AsoĞlu E. Evaluation of epicardial adipose tissue by echocardiography and its correlation with aortic velocity propagation and carotid intima-media thickness in patients of type 2 diabetes mellitus. Acad Bras Cienc (2020) 92(4):e20191457. doi: 10.1590/0001-3765202020191457
91. Graeff DB, Foppa M, Pires JCG, Vigo A, Schmidt MI, Lotufo PA, et al. Epicardial fat thickness: Distribution and association with diabetes mellitus, hypertension and the metabolic syndrome in the Elsa-brasil study. Int J Cardiovasc Imaging (2016) 32(4):563–72. doi: 10.1007/s10554-015-0810-z
92. Loncarevic B, Trifunovic D, Soldatovic I, Vujisic-Tesic B. Silent diabetic cardiomyopathy in everyday practice: A clinical and echocardiographic study. BMC Cardiovasc Disord (2016) 16(1):242. doi: 10.1186/s12872-016-0395-z
93. Raghothama S, Rao A. Revelation of subclinical left ventricular diastolic dysfunction in patients with type 2 diabetes mellitus using 2016 ase/ eacvi guidelines. Caspian J Internal Med (2021) 12(4):586–92. doi: 10.22088/cjim.12.4.586
94. Tadic M, Vukomanovic V, Cuspidi C, Suzic-Lazic J, Stanisavljevic D, Celic V. Left atrial phasic function and heart rate variability in asymptomatic diabetic patients. Acta Diabetologica (2017) 54(3):301–8. doi: 10.1007/s00592-016-0962-x
95. Vukomanovic V, Suzic-Lazic J, Celic V, Cuspidi C, Grassi G, Galderisi M, et al. Is there association between left atrial function and functional capacity in patients with uncomplicated type 2 diabetes? Int J Cardiovasc Imaging (2020) 36(1):15–22. doi: 10.1007/s10554-019-01680-z
96. Liu JE, Palmieri V, Roman MJ, Bella JN, Fabsitz R, Howard BV, et al. The impact of diabetes on left ventricular filling pattern in normotensive and hypertensive adults: The strong heart study. J Am Coll Cardiol (2001) 37(7):1943–9. doi: 10.1016/s0735-1097(01)01230-x
97. Nicolino A, Longobardi G, Furgi G, Rossi M, Zoccolillo N, Ferrara N, et al. Left ventricular diastolic filling in diabetes mellitus with and without hypertension. Am J hypertension (1995) 8(4 Pt 1):382–9. doi: 10.1016/0895-7061(95)00022-H
98. Hodzic A, Ribault V, Maragnes P, Milliez P, Saloux E, Labombarda F. Decreased regional left ventricular myocardial strain in type 1 diabetic children: A first sign of diabetic cardiomyopathy? J Trans Internal Med (2016) 4(2):81–7. doi: 10.1515/jtim-2016-0025
99. Raev DC. Which left ventricular function is impaired earlier in the evolution of diabetic cardiomyopathy? an echocardiographic study of young type I diabetic patients. Diabetes Care (1994) 17(7):633–9. doi: 10.2337/diacare.17.7.633
100. Schannwell CM, Schneppenheim M, Perings S, Plehn G, Strauer BE. Left ventricular diastolic dysfunction as an early manifestation of diabetic cardiomyopathy. Cardiology (2002) 98(1-2):33–9. doi: 10.1159/000064682
101. Santos CD, Souza AM, Pereira RM, Boguszewski MC, França SN, Vieira CG, et al. [Assessment of diastolic function in children and adolescents with type 1 diabetes mellitus - are there early signs of diabetic cardiomyopathy?]. Arquivos brasileiros endocrinologia e metabologia (2012) 56(4):226–32. doi: 10.1590/s0004-27302012000400002
102. Andersen NH, Poulsen SH, Eiskjaer H, Poulsen PL, Mogensen CE. Decreased left ventricular longitudinal contraction in normotensive and normoalbuminuric patients with type ii diabetes mellitus: A Doppler tissue tracking and strain rate echocardiography study. Clin Sci (London Engl 1979) (2003) 105(1):59–66. doi: 10.1042/CS20020303
103. Ernande L, Bergerot C, Rietzschel ER, De Buyzere ML, Thibault H, Pignonblanc PG, et al. Diastolic dysfunction in patients with type 2 diabetes mellitus: Is it really the first marker of diabetic cardiomyopathy? J Am Soc Echocardiography Off Publ Am Soc Echocardiography (2011) 24(11):1268–1275.e1. doi: 10.1016/j.echo.2011.07.017
104. Atas H, Kepez A, Atas DB, Kanar BG, Dervisova R, Kivrak T, et al. Effects of diabetes mellitus on left atrial volume and functions in normotensive patients without symptomatic cardiovascular disease. J Diabetes Its Complications (2014) 28(6):858–62. doi: 10.1016/j.jdiacomp.2014.07.010
105. Adal E, Koyuncu G, Aydin A, Celebi A, Kavunoğlu G, Cam H. Asymptomatic cardiomyopathy in children and adolescents with type 1 diabetes mellitus: Association of echocardiographic indicators with duration of diabetes mellitus and metabolic parameters. J Pediatr Endocrinol Metab JPEM (2006) 19(5):713–26. doi: 10.1515/JPEM.2006.19.5.713
106. Ng ACT, Delgado V, Bertini M, van der Meer RW, Rijzewijk LJ, Shanks M, et al. Findings from left ventricular strain and strain rate imaging in asymptomatic patients with type 2 diabetes mellitus. Am J Cardiol (2009) 104(10):1398–401. doi: 10.1016/j.amjcard.2009.06.063
107. Riggs TW, Transue D. Doppler Echocardiographic evaluation of left ventricular diastolic function in adolescents with diabetes mellitus. Am J Cardiol (1990) 65(13):899–902. doi: 10.1016/0002-9149(90)91433-7
108. Zairi I, Mzoughi K, Kamoun S, Moussa FB, Rezgallah R, Maatoug J, et al. Impairment of left and right ventricular longitudinal strain in asymptomatic children with type 1 diabetes. Indian Heart J (2019) 71(3):249–55. doi: 10.1016/j.ihj.2019.04.008
109. Ahmed TA, Ahmed YA, Arafa AI, Salah RA. Detection of occult right ventricular dysfunction in young egyptians with type 1 diabetes mellitus by two-dimensional speckle tracking echocardiography. Indian Heart J (2018) 70(5):665–71. doi: 10.1016/j.ihj.2018.06.019
110. Stevanovic A, Dekleva M. The importance of subclinical left ventricular dysfunction and blood pressure pattern in asymptomatic type-2 diabetic patients: The diagnostic and prognostic significance of tissue Doppler parameters, left ventricular global longitudinal strain, and nighttime blood pressure during sleep. J Diabetes Complications (2018) 32(1):41–7. doi: 10.1016/j.jdiacomp.2017.07.022
111. Zoppini G, Bergamini C, Mantovani A, Dauriz M, Targher G, Rossi A, et al. The E/E' ratio difference between subjects with type 2 diabetes and controls. a meta-analysis of clinical studies. PloS One (2018) 13(12):e0209794. doi: 10.1371/journal.pone.0209794
112. Pattoneri P, Sozzi FB, Catellani E, Piazza A, Iotti R, Michelini M, et al. Myocardial involvement during the early course of type 2 diabetes mellitus: Usefulness of myocardial performance index. Cardiovasc ultrasound (2008) 6:27. doi: 10.1186/1476-7120-6-27
113. Vukomanovic V, Tadic M, Suzic-Lazic J, Kocijancic V, Celic V. The relationship between heart rate variability and left ventricular layer-specific deformation in uncomplicated diabetic patients. Int J Cardiovasc Imaging (2017) 33(4):481–90. doi: 10.1007/s10554-016-1023-9
114. Labombarda F, Leport M, Morello R, Ribault V, Kauffman D, Brouard J, et al. Longitudinal left ventricular strain impairment in type 1 diabetes children and adolescents: A 2d speckle strain imaging study. Diabetes Metab (2014) 40(4):292–8. doi: 10.1016/j.diabet.2014.03.007
115. Kadappu KK, Boyd A, Eshoo S, Haluska B, Yeo AET, Marwick TH, et al. Changes in left atrial volume in diabetes mellitus: More than diastolic dysfunction? Eur Heart J Cardiovasc Imaging (2012) 13(12):1016–23. doi: 10.1093/ehjci/jes084
116. Ifuku M, Takahashi K, Hosono Y, Iso T, Ishikawa A, Haruna H, et al. Left atrial dysfunction and stiffness in pediatric and adult patients with type 1 diabetes mellitus assessed with speckle tracking echocardiography. Pediatr Diabetes (2021) 22(2):303–19. doi: 10.1111/pedi.13141
117. Liao L, Shi B, Ding Z, Chen L, Dong F, Li J, et al. Echocardiographic study of myocardial work in patients with type 2 diabetes mellitus. BMC Cardiovasc Disord (2022) 22(1):59. doi: 10.1186/s12872-022-02482-3
118. Jędrzejewska I, Król W, Światowiec A, Wilczewska A, Grzywanowska-Łaniewska I, Dłużniewski M, et al. Left and right ventricular systolic function impairment in type 1 diabetic young adults assessed by 2d speckle tracking echocardiography. Eur Heart J Cardiovasc Imaging (2016) 17(4):438–46. doi: 10.1093/ehjci/jev164
119. Enomoto M, Ishizu T, Seo Y, Yamamoto M, Suzuki H, Shimano H, et al. Subendocardial systolic dysfunction in asymptomatic normotensive diabetic patients. Circ J (2015) 79(8):1749–55. doi: 10.1253/circj.CJ-15-0012
120. Mochizuki Y, Tanaka H, Matsumoto K, Sano H, Shimoura H, Ooka J, et al. Impact of left ventricular longitudinal functional mechanics on the progression of diastolic function in diabetes mellitus. Int J Cardiovasc Imaging (2017) 33(12):1905–14. doi: 10.1007/s10554-017-1198-8
121. Iso T, Takahashi K, Yazaki K, Ifuku M, Nii M, Fukae T, et al. In-depth insight into the mechanisms of cardiac dysfunction in patients with type 1 diabetes mellitus using layer-specific strain analysis. Circ J Off J Japanese Circ Soc (2019) 83(6):1330–7. doi: 10.1253/circj.CJ-18-1245
122. Todo S, Tanaka H, Yamauchi Y, Yokota S, Mochizuki Y, Shiraki H, et al. Association of left ventricular longitudinal myocardial function with subclinical right ventricular dysfunction in type 2 diabetes mellitus. Cardiovasc Diabetol (2021) 20(1):212. doi: 10.1186/s12933-021-01404-5
123. Li XY, Dong YY, Zheng C, Wang PF, Xu MS, Zou CP, et al. Assessment of real-time three-dimensional echocardiography as a tool for evaluating left atrial volume and function in patients with type 2 diabetes mellitus. Aging-Us (2021) 13(1):991–1000. doi: 10.18632/aging.202218
124. Chen X, Guo H, Yang Q, Fang J, Kang X. Quantitative evaluation of subclinical left ventricular dysfunction in patients with type 2 diabetes mellitus by three-dimensional echocardiography. Int J Cardiovasc Imaging (2020) 36(7):1311–9. doi: 10.1007/s10554-020-01833-5
125. Ringle A, Dornhorst A, Rehman MB, Ruisanchez C, Nihoyannopoulos P. Evolution of subclinical myocardial dysfunction detected by two-dimensional and three-dimensional speckle tracking in asymptomatic type 1 diabetic patients: A Long−Term follow-up study. Echo Res Pract (2017) 4(4):73–81. doi: 10.1530/erp-17-0052
126. Ghoreyshi-Hefzabad SM, Jeyaprakash P, Gupta A, Vo HQ, Pathan F, Negishi K. Three-dimensional global left ventricular myocardial strain reduced in all directions in subclinical diabetic cardiomyopathy: A systematic review and meta-analysis. J Am Heart Assoc (2021) 10(19):e020811. doi: 10.1161/jaha.121.020811
127. Ha J-W, Lee H-C, Kang E-S, Ahn C-M, Kim J-M, Ahn J-A, et al. Abnormal left ventricular longitudinal functional reserve in patients with diabetes mellitus: Implication for detecting subclinical myocardial dysfunction using exercise tissue Doppler echocardiography. Heart (British Cardiac Society) (2007) 93(12):1571–6. doi: 10.1136/hrt.2006.101667
128. Philouze C, Obert P, Nottin S, Benamor A, Barthez O, Aboukhoudir F. Dobutamine stress echocardiography unmasks early left ventricular dysfunction in asymptomatic patients with uncomplicated type 2 diabetes: A comprehensive two-dimensional speckle-tracking imaging study. J Am Soc Echocardiography Off Publ Am Soc Echocardiography (2018) 31(5):587–97. doi: 10.1016/j.echo.2017.12.006
129. Yonaha O, Matsubara T, Naruse K, Ishii H, Murohara T, Nakamura J, et al. Effects of reduced coronary flow reserve on left ventricular function in type 2 diabetes. Diabetes Res Clin Pract (2008) 82(1):98–103. doi: 10.1016/j.diabres.2008.06.020
130. Jani Y, Kamberi A, Xhunga S, Pocesta B, Ferati F, Lala D, et al. The influence of type 2 diabetes and gender on ventricular repolarization dispersion in patients with Sub-clinic left ventricular diastolic dysfunction. Am J Cardiovasc Dis (2015) 5(4):155–66.
131. Voulgari C, Tentolouris N, Moyssakis I, Dilaveris P, Gialafos E, Papadogiannis D, et al. Spatial qrs-T angle: Association with diabetes and left ventricular performance. Eur J Clin Invest (2006) 36(9):608–13. doi: 10.1111/j.1365-2362.2006.01697.x
132. Hjortkjær H, Jensen T, Hilsted J, Mogensen UM, Corinth H, Rossing P, et al. Cardiac ventricular sizes are reduced in patients with long-term, normoalbuminuric type 1 diabetes compared to the non-diabetic background population. Diabetes Vasc Dis Res (2019) 16(3):289–96. doi: 10.1177/1479164118819961
133. Shao G, Cao Y, Cui Y, Han X, Liu J, Li Y, et al. Early detection of left atrial and bi-ventricular myocardial strain abnormalities by mri feature tracking in normotensive or hypertensive T2dm patients with preserved lv function. BMC Cardiovasc Disord (2020) 20(1):196. doi: 10.1186/s12872-020-01469-2
134. Athithan L, Chowdhary A, Swarbrick D, Gulsin GS, Singh A, Jex N, et al. Male Sex adversely affects the phenotypic expression of diabetic heart disease. Ther Adv Endocrinol Metab (2020) 11:2042018820927179. doi: 10.1177/2042018820927179
135. Shang Y, Zhang X, Leng W, Lei X, Chen L, Liang Z, et al. Left atrium passive ejection fraction is the most sensitive index of type 2 diabetes mellitus-related cardiac changes. Int J Cardiovasc Imaging (2018) 34(1):141–51. doi: 10.1007/s10554-017-1213-0
136. Larghat AM, Swoboda PP, Biglands JD, Kearney MT, Greenwood JP, Plein S. The microvascular effects of insulin resistance and diabetes on cardiac structure, function, and perfusion: A cardiovascular magnetic resonance study. Eur Heart J Cardiovasc Imaging (2014) 15(12):1368–76. doi: 10.1093/ehjci/jeu142
137. Shang Y, Zhang X, Leng W, Lei X, Chen L, Zhou X, et al. Increased fractal dimension of left ventricular trabeculations is associated with subclinical diastolic dysfunction in patients with type-2 diabetes mellitus. Int J Cardiovasc Imaging (2019) 35(4):665–73. doi: 10.1007/s10554-018-1492-0
138. Levelt E, Mahmod M, Piechnik SK, Ariga R, Francis JM, Rodgers CT, et al. Relationship between left ventricular structural and metabolic remodeling in type 2 diabetes. Diabetes (2016) 65(1):44–52. doi: 10.2337/db15-0627
139. Shang Y, Zhang X, Chen L, Leng W, Lei X, Yang Q, et al. Assessment of left ventricular structural remodelling in patients with diabetic cardiomyopathy by cardiovascular magnetic resonance. J Diabetes Res (2016) 2016:4786925. doi: 10.1155/2016/4786925
140. Rospleszcz S, Schafnitzel A, Koenig W, Lorbeer R, Auweter S, Huth C, et al. Association of glycemic status and segmental left ventricular wall thickness in subjects without prior cardiovascular disease: A cross-sectional study. BMC Cardiovasc Disord (2018) 18(1):162. doi: 10.1186/s12872-018-0900-7
141. Schäfer M, Bjornstad P, Frank BS, Baumgartner A, Truong U, Enge D, et al. Frequency of reduced left ventricular contractile efficiency and discoordinated myocardial relaxation in patients aged 16 to 21 years with type 1 diabetes mellitus (from the emerald study). Am J Cardiol (2020) 128:45–53. doi: 10.1016/j.amjcard.2020.04.041
142. Zhou FL, Deng MY, Deng LL, Li YM, Mo D, Xie LJ, et al. Evaluation of the effects of glycated hemoglobin on cardiac function in patients with short-duration type 2 diabetes mellitus: A cardiovascular magnetic resonance study. Diabetes Res Clin Pract (2021) 178:108952. doi: 10.1016/j.diabres.2021.108952
143. Widya RL, van der Meer RW, Smit JW, Rijzewijk LJ, Diamant M, Bax JJ, et al. Right ventricular involvement in diabetic cardiomyopathy. Diabetes Care (2013) 36(2):457–62. doi: 10.2337/dc12-0474
144. Rider OJ, Apps A, Miller J, Lau JYC, Lewis AJM, Peterzan MA, et al. Noninvasive in vivo assessment of cardiac metabolism in the healthy and diabetic human heart using hyperpolarized (13)C mri. Circ Res (2020) 126(6):725–36. doi: 10.1161/circresaha.119.316260
145. Jiang L, Shi K, Guo YK, Ren Y, Li ZL, Xia CC, et al. The additive effects of obesity on myocardial microcirculation in diabetic individuals: A cardiac magnetic resonance first-pass perfusion study. Cardiovasc Diabetol (2020) 19(1):52. doi: 10.1186/s12933-020-01028-1
146. Levelt E, Rodgers CT, Clarke WT, Mahmod M, Ariga R, Francis JM, et al. Cardiac energetics, oxygenation, and perfusion during increased workload in patients with type 2 diabetes mellitus. Eur Heart J (2016) 37(46):3461–9. doi: 10.1093/eurheartj/ehv442
147. Levelt E, Piechnik SK, Liu A, Wijesurendra RS, Mahmod M, Ariga R, et al. Adenosine stress cmr T1-mapping detects early microvascular dysfunction in patients with type 2 diabetes mellitus without obstructive coronary artery disease. J Cardiovasc magnetic resonance Off J Soc Cardiovasc Magnetic Resonance (2017) 19(1):81. doi: 10.1186/s12968-017-0397-8
148. Storz C, Hetterich H, Lorbeer R, Heber SD, Schafnitzel A, Patscheider H, et al. Myocardial tissue characterization by contrast-enhanced cardiac magnetic resonance imaging in subjects with prediabetes, diabetes, and normal controls with preserved ejection fraction from the general population. Eur Heart Journal-Cardiovascular Imaging (2018) 19(6):701–8. doi: 10.1093/ehjci/jex190
149. Vasanji Z, Sigal RJ, Eves ND, Isaac DL, Friedrich MG, Chow K, et al. Increased left ventricular extracellular volume and enhanced twist function in type 1 diabetic individuals. J Appl Physiol (Bethesda Md 1985) (2017) 123(2):394–401. doi: 10.1152/japplphysiol.00012.2017
150. Gao Y, Yang ZG, Ren Y, Liu X, Jiang L, Xie LJ, et al. Evaluation of myocardial fibrosis in diabetes with cardiac magnetic resonance T1-mapping: Correlation with the high-level hemoglobin A1c. Diabetes Res Clin Pract (2019) 150:72–80. doi: 10.1016/j.diabres.2019.03.004
151. Shah RV, Abbasi SA, Neilan TG, Hulten E, Coelho-Filho O, Hoppin A, et al. Myocardial tissue remodeling in adolescent obesity. J Am Heart Assoc (2013) 2(4):e000279. doi: 10.1161/JAHA.113.000279
152. Ng ACT, Auger D, Delgado V, van Elderen SGC, Bertini M, Siebelink H-M, et al. Association between diffuse myocardial fibrosis by cardiac magnetic resonance contrast-enhanced T1 mapping and subclinical myocardial dysfunction in diabetic patients: A pilot study. Circ Cardiovasc Imaging (2012) 5(1):51–9. doi: 10.1161/CIRCIMAGING.111.965608
153. McGavock JM, Lingvay I, Zib I, Tillery T, Salas N, Unger R, et al. Cardiac steatosis in diabetes mellitus: A 1h-magnetic resonance spectroscopy study. Circulation (2007) 116(10):1170–5. doi: 10.1161/CIRCULATIONAHA.106.645614
154. Scheuermann-Freestone M, Madsen PL, Manners D, Blamire AM, Buckingham RE, Styles P, et al. Abnormal cardiac and skeletal muscle energy metabolism in patients with type 2 diabetes. Circulation (2003) 107(24):3040–6. doi: 10.1161/01.CIR.0000072789.89096.10
155. Hosseinzadeh E, Ghodsirad MA, Alirezaei T, Arfenia M, Pirayesh, Amoiee M, et al. Comparing left ventricular mechanical dyssynchrony between diabetic and non-diabetic patients with normal gated spect mpi. Int J Cardiovasc Imaging (2021) 38(1):249–256. doi: 10.1007/s10554-021-02358-1
156. Herrero P, McGill J, Lesniak DS, Dence CS, Scott SW, Kisrieva-Ware Z, et al. Pet detection of the impact of dobutamine on myocardial glucose metabolism in women with type 1 diabetes mellitus. J Nucl Cardiol Off Publ Am Soc Nucl Cardiol (2008) 15(6):791–9. doi: 10.1007/BF03007360
157. Peterson LR, Herrero P, Coggan AR, Kisrieva-Ware Z, Saeed I, Dence C, et al. Type 2 diabetes, obesity, and sex difference affect the fate of glucose in the human heart. Am J Physiol Heart Circulatory Physiol (2015) 308(12):H1510–H6. doi: 10.1152/ajpheart.00722.2014
158. McGill JB, Peterson LR, Herrero P, Saeed IM, Recklein C, Coggan AR, et al. Potentiation of abnormalities in myocardial metabolism with the development of diabetes in women with obesity and insulin resistance. J Nucl Cardiol Off Publ Am Soc Nucl Cardiol (2011) 18(3):421–9. doi: 10.1007/s12350-011-9362-3
159. Rijzewijk LJ, van der Meer RW, Lamb HJ, de Jong HWAM, Lubberink M, Romijn JA, et al. Altered myocardial substrate metabolism and decreased diastolic function in nonischemic human diabetic cardiomyopathy: Studies with cardiac positron emission tomography and magnetic resonance imaging. J Am Coll Cardiol (2009) 54(16):1524–32. doi: 10.1016/j.jacc.2009.04.074
160. Hu L, Qiu C, Wang X, Xu M, Shao X, Wang Y. The association between diabetes mellitus and reduction in myocardial glucose uptake: A population-based f-fdg Pet/Ct study. BMC Cardiovasc Disord (2018) 18(1):203. doi: 10.1186/s12872-018-0943-9
161. Peterson LR, Saeed IM, McGill JB, Herrero P, Schechtman KB, Gunawardena R, et al. Sex and type 2 diabetes: Obesity-independent effects on left ventricular substrate metabolism and relaxation in humans. Obes (Silver Spring Md) (2012) 20(4):802–10. doi: 10.1038/oby.2011.208
162. Herrero P, Peterson LR, McGill JB, Matthew S, Lesniak D, Dence C, et al. Increased myocardial fatty acid metabolism in patients with type 1 diabetes mellitus. J Am Coll Cardiol (2006) 47(3):598–604. doi: 10.1016/j.jacc.2005.09.030
163. Peterson LR, Herrero P, McGill J, Schechtman KB, Kisrieva-Ware Z, Lesniak D, et al. Fatty acids and insulin modulate myocardial substrate metabolism in humans with type 1 diabetes. Diabetes (2008) 57(1):32–40. doi: 10.2337/db07-1199
164. Kjaer A, Meyer C, Nielsen FS, Parving H-H, Hesse B. Dipyridamole, cold pressor test, and demonstration of endothelial dysfunction: A pet study of myocardial perfusion in diabetes. J Nucl Med Off publication Soc Nucl Med (2003) 44(1):19–23.
165. Gallinoro E, Paolisso P, Candreva A, Bermpeis K, Fabbricatore D, Esposito G, et al. Microvascular dysfunction in patients with type ii diabetes mellitus: Invasive assessment of absolute coronary blood flow and microvascular resistance reserve. Front Cardiovasc Med (2021) 8:765071. doi: 10.3389/fcvm.2021.765071
166. Holscher ME, Bode C, Bugger H. Diabetic cardiomyopathy: Does the type of diabetes matter? Int J Mol Sci (2016) 17(12):2136. doi: 10.3390/ijms17122136
167. Pappachan JM, Varughese GI, Sriraman R, Arunagirinathan G. Diabetic cardiomyopathy: Pathophysiology, diagnostic evaluation and management. World J Diabetes (2013) 4(5):177–89. doi: 10.4239/wjd.v4.i5.177
168. Stanton AM, Vaduganathan M, Chang LS, Turchin A, Januzzi JL Jr., Aroda VR. Asymptomatic diabetic cardiomyopathy: An underrecognized entity in type 2 diabetes. Curr Diabetes Rep (2021) 21(10):41. doi: 10.1007/s11892-021-01407-2
169. Kiencke S, Handschin R, von Dahlen R, Muser J, Brunner-Larocca HP, Schumann J, et al. Pre-clinical diabetic cardiomyopathy: Prevalence, screening, and outcome. Eur J Heart failure (2010) 12(9):951–7. doi: 10.1093/eurjhf/hfq110
170. Fang ZY, Schull-Meade R, Leano R, Mottram PM, Prins JB, Marwick TH. Screening for heart disease in diabetic subjects. Am Heart J (2005) 149(2):349–54. doi: 10.1016/j.ahj.2004.06.021
171. Redfield MM, Rodeheffer RJ, Jacobsen SJ, Mahoney DW, Bailey KR, Burnett JC Jr. Plasma brain natriuretic peptide to detect preclinical ventricular systolic or diastolic dysfunction: A community-based study. Circulation (2004) 109(25):3176–81. doi: 10.1161/01.Cir.0000130845.38133.8f
172. Yancy CW, Jessup M, Bozkurt B, Butler J, Casey DE Jr., Drazner MH, et al. Accf/Aha guideline for the management of heart failure: Executive summary: A report of the American college of cardiology Foundation/American heart association task force on practice guidelines. Circulation (2013) 128(16):1810–52. doi: 10.1161/CIR.0b013e31829e8807
173. Ledwidge M, Gallagher J, Conlon C, Tallon E, O'Connell E, Dawkins I, et al. Natriuretic peptide-based screening and collaborative care for heart failure: The stop-hf randomized trial. Jama (2013) 310(1):66–74. doi: 10.1001/jama.2013.7588
174. Huelsmann M, Neuhold S, Resl M, Strunk G, Brath H, Francesconi C, et al. Pontiac (Nt-probnp selected prevention of cardiac events in a population of diabetic patients without a history of cardiac disease): A prospective randomized controlled trial. J Am Coll Cardiol (2013) 62(15):1365–72. doi: 10.1016/j.jacc.2013.05.069
175. Devereux RB, Roman MJ, Paranicas M, O'Grady MJ, Lee ET, Welty TK, et al. Impact of diabetes on cardiac structure and function: The strong heart study. Circulation (2000) 101(19):2271–6. doi: 10.1161/01.CIR.101.19.2271
176. Miki T, Yuda S, Kouzu H, Miura T. Diabetic cardiomyopathy: Pathophysiology and clinical features. Heart Fail Rev (2013) 18(2):149–66. doi: 10.1007/s10741-012-9313-3
177. Ahmed HM, Khraishah H, Cho L. Cardioprotective anti-hyperglycaemic medications: A review of clinical trials. Eur Heart J (2018) 39(25):2368–75. doi: 10.1093/eurheartj/ehx668
178. Oe H, Nakamura K, Kihara H, Shimada K, Fukuda S, Takagi T, et al. Comparison of effects of sitagliptin and voglibose on left ventricular diastolic dysfunction in patients with type 2 diabetes: Results of the 3d trial. Cardiovasc Diabetol (2015) 14:83. doi: 10.1186/s12933-015-0242-z
179. Yamada H, Tanaka A, Kusunose K, Amano R, Matsuhisa M, Daida H, et al. Effect of sitagliptin on the echocardiographic parameters of left ventricular diastolic function in patients with type 2 diabetes: A subgroup analysis of the prologue study. Cardiovasc Diabetol (2017) 16(1):63. doi: 10.1186/s12933-017-0546-2
180. Brown AJM, Lang C, McCrimmon R, Struthers A. Does dapagliflozin regress left ventricular hypertrophy in patients with type 2 diabetes? a prospective, double-blind, randomised, placebo-controlled study. BMC Cardiovasc Disord (2017) 17(1):229. doi: 10.1186/s12872-017-0663-6
181. Brown AJM, Gandy S, McCrimmon R, Houston JG, Struthers AD, Lang CC. A randomized controlled trial of dapagliflozin on left ventricular hypertrophy in people with type two diabetes: The dapa-lvh trial. Eur Heart J (2020) 41(36):3421–32. doi: 10.1093/eurheartj/ehaa419
182. Matsutani D, Sakamoto M, Kayama Y, Takeda N, Horiuchi R, Utsunomiya K. Effect of canagliflozin on left ventricular diastolic function in patients with type 2 diabetes. Cardiovasc Diabetol (2018) 17(1):73. doi: 10.1186/s12933-018-0717-9
183. Oka S, Kai T, Hoshino K, Watanabe K, Nakamura J, Abe M, et al. Effects of empagliflozin in different phases of diabetes mellitus-related cardiomyopathy: A prospective observational study. BMC Cardiovasc Disord (2021) 21(1):217. doi: 10.1186/s12872-021-02024-3
184. Thirunavukarasu S, Jex N, Chowdhary A, Hassan IU, Straw S, Craven TP, et al. Empagliflozin treatment is associated with improvements in cardiac energetics and function and reductions in myocardial cellular volume in patients with type 2 diabetes. Diabetes (2021) 70(12):2810–22. doi: 10.2337/db21-0270
185. Scalzo RL, Moreau KL, Ozemek C, Herlache L, McMillin S, Gilligan S, et al. Exenatide improves diastolic function and attenuates arterial stiffness but does not alter exercise capacity in individuals with type 2 diabetes. J Diabetes Complications (2017) 31(2):449–55. doi: 10.1016/j.jdiacomp.2016.10.003
186. Chen WJY, Diamant M, de Boer K, Harms HJ, Robbers L, van Rossum AC, et al. Effects of exenatide on cardiac function, perfusion, and energetics in type 2 diabetic patients with cardiomyopathy: A randomized controlled trial against insulin glargine. Cardiovasc Diabetol (2017) 16(1):67. doi: 10.1186/s12933-017-0549-z
187. Lambadiari V, Pavlidis G, Kousathana F, Varoudi M, Vlastos D, Maratou E, et al. Effects of 6-month treatment with the glucagon like peptide-1 analogue liraglutide on arterial stiffness, left ventricular myocardial deformation and oxidative stress in subjects with newly diagnosed type 2 diabetes. Cardiovasc Diabetol (2018) 17(1):8. doi: 10.1186/s12933-017-0646-z
188. Bizino MB, Jazet IM, Westenberg JJM, van Eyk HJ, Paiman EHM, Smit JWA, et al. Effect of liraglutide on cardiac function in patients with type 2 diabetes mellitus: Randomized placebo-controlled trial. Cardiovasc Diabetol (2019) 18(1):55. doi: 10.1186/s12933-019-0857-6
189. Paiman EHM, van Eyk HJ, van Aalst MMA, Bizino MB, van der Geest RJ, Westenberg JJM, et al. Effect of liraglutide on cardiovascular function and myocardial tissue characteristics in type 2 diabetes patients of south Asian descent living in the Netherlands: A double-blind, randomized, placebo-controlled trial. J Magn Reson Imaging (2020) 51(6):1679–88. doi: 10.1002/jmri.27009
190. Ikonomidis I, Pavlidis G, Thymis J, Birba D, Kalogeris A, Kousathana F, et al. Effects of glucagon-like peptide-1 receptor agonists, sodium-glucose cotransporter-2 inhibitors, and their combination on endothelial glycocalyx, arterial function, and myocardial work index in patients with type 2 diabetes mellitus after 12-month treatment. J Am Heart Assoc (2020) 9(9):e015716. doi: 10.1161/JAHA.119.015716
191. Giannetta E, Isidori AM, Galea N, Carbone I, Mandosi E, Vizza CD, et al. Chronic inhibition of cgmp phosphodiesterase 5a improves diabetic cardiomyopathy: A randomized, controlled clinical trial using magnetic resonance imaging with myocardial tagging. Circulation (2012) 125(19):2323–33. doi: 10.1161/CIRCULATIONAHA.111.063412
192. Giannattasio S, Corinaldesi C, Colletti M, Di Luigi L, Antinozzi C, Filardi T, et al. The phosphodiesterase 5 inhibitor sildenafil decreases the proinflammatory chemokine il-8 in diabetic cardiomyopathy: In vivo and in vitro evidence. J Endocrinol Invest (2019) 42(6):715–25. doi: 10.1007/s40618-018-0977-y
193. Zhao P, Zhang J, Yin XG, Maharaj P, Narraindoo S, Cui LQ, et al. The effect of trimetazidine on cardiac function in diabetic patients with idiopathic dilated cardiomyopathy. Life Sci (2013) 92(11):633–8. doi: 10.1016/j.lfs.2012.03.015
194. Annonu AK, Fattah AA, Mokhtar MS, Ghareeb S, Elhendy A. Left ventricular systolic and diastolic functional abnormalities in asymptomatic patients with non-Insulin-Dependent diabetes mellitus. J Am Soc Echocardiography Off Publ Am Soc Echocardiography (2001) 14(9):885–91. doi: 10.1067/mje.2001.112892
195. Markus MRP, Stritzke J, Wellmann J, Duderstadt S, Siewert U, Lieb W, et al. Implications of prevalent and incident diabetes mellitus on left ventricular geometry and function in the ageing heart: The Monica/Kora augsburg cohort study. Nutrition Metabolism Cardiovasc Dis NMCD (2011) 21(3):189–96. doi: 10.1016/j.numecd.2009.09.005
196. Julian MT, Alonso N, Lupon J, Gavidia-Bovadilla G, Ferrer E, de Antonio M, et al. Long-term lvef trajectories in patients with type 2 diabetes and heart failure: Diabetic cardiomyopathy may underlie functional decline. Cardiovasc Diabetol (2020) 19(1):38. doi: 10.1186/s12933-020-01011-w
197. Pellikka PA, She L, Holly TA, Lin G, Varadarajan P, Pai RG, et al. Variability in ejection fraction measured by echocardiography, gated single-photon emission computed tomography, and cardiac magnetic resonance in patients with coronary artery disease and left ventricular dysfunction. JAMA network Open (2018) 1(4):e181456. doi: 10.1001/jamanetworkopen.2018.1456
198. Sarvari SI, Haugaa KH, Zahid W, Bendz B, Aakhus S, Aaberge L, et al. Layer-specific quantification of myocardial deformation by strain echocardiography may reveal significant cad in patients with non-St-Segment elevation acute coronary syndrome. JACC Cardiovasc Imaging (2013) 6(5):535–44. doi: 10.1016/j.jcmg.2013.01.009
199. Movahed M-R, Hashemzadeh M, Jamal MM. The prevalence of pulmonary embolism and pulmonary hypertension in patients with type ii diabetes mellitus. Chest (2005) 128(5):3568–71. doi: 10.1016/S0012-3692(15)52932-2
200. Benson L, Brittain EL, Pugh ME, Austin ED, Fox K, Wheeler L, et al. Impact of diabetes on survival and right ventricular compensation in pulmonary arterial hypertension. Pulmonary Circ (2014) 4(2):311–8. doi: 10.1086/675994
201. Movahed M-R, Milne N. Presence of biventricular dysfunction in patients with type ii diabetes mellitus. Congest Heart Fail (2007) 13(2):78–80. doi: 10.1111/j.1527-5299.2007.888138.x
202. Tadic M, Ilic S, Cuspidi C, Ivanovic B, Bukarica L, Kostic N, et al. Left and right atrial phasic function and deformation in untreated patients with prediabetes and type 2 diabetes mellitus. Int J Cardiovasc Imaging (2015) 31(1):65–76. doi: 10.1007/s10554-014-0536-3
203. Tadic M, Celic V, Cuspidi C, Ilic S, Pencic B, Radojkovic J, et al. Right heart mechanics in untreated normotensive patients with prediabetes and type 2 diabetes mellitus: A two- and three-dimensional echocardiographic study. J Am Soc Echocardiography Off Publ Am Soc Echocardiography (2015) 28(3):317–27. doi: 10.1016/j.echo.2014.11.017
204. Jensen MT, Fung K, Aung N, Sanghvi MM, Chadalavada S, Paiva JM, et al. Changes in cardiac morphology and function in individuals with diabetes mellitus: The uk biobank cardiovascular magnetic resonance substudy. Circ Cardiovasc Imaging (2019) 12(9):e009476. doi: 10.1161/circimaging.119.009476
205. Felício JS, Koury CC, Carvalho CT, Abrahão Neto JF, Miléo KB, Arbage TP, et al. Present insights on cardiomyopathy in diabetic patients. Curr Diabetes Rev (2016) 12(4):384–95. doi: 10.2174/1573399812666150914120529
206. Ulu SM, Dogan M, Ahsen A, Altug A, Demir K, Acartürk G, et al. Neutrophil-to-Lymphocyte ratio as a quick and reliable predictive marker to diagnose the severity of diabetic retinopathy. Diabetes Technol Ther (2013) 15(11):942–7. doi: 10.1089/dia.2013.0097
207. Palomer X, Pizarro-Delgado J, Vázquez-Carrera M. Emerging actors in diabetic cardiomyopathy: Heartbreaker biomarkers or therapeutic targets? Trends Pharmacol Sci (2018) 39(5):452–67. doi: 10.1016/j.tips.2018.02.010
208. Kong L, Zhu J, Han W, Jiang X, Xu M, Zhao Y, et al. Significance of serum micrornas in pre-diabetes and newly diagnosed type 2 diabetes: A clinical study. Acta Diabetologica (2011) 48(1):61–9. doi: 10.1007/s00592-010-0226-0
209. Ahmed U, Ashfaq UA, Qasim M, Ahmad I, Ahmad HU, Tariq M, et al. Dysregulation of circulating mirnas promotes the pathogenesis of diabetes-induced cardiomyopathy. PloS One (2021) 16(4):e0250773. doi: 10.1371/journal.pone.0250773
210. Lumens J, Delhaas T, Arts T, Cowan BR, Young AA. Impaired subendocardial contractile myofiber function in asymptomatic aged humans, as detected using mri. Am J Physiol Heart Circulatory Physiol (2006) 291(4):H1573–H9. doi: 10.1152/ajpheart.00074.2006
211. Cameli M, Mandoli GE, Lisi E, Ibrahim A, Incampo E, Buccoliero G, et al. Left atrial, ventricular and atrio-ventricular strain in patients with subclinical heart dysfunction. Int J Cardiovasc Imaging (2019) 35(2):249–58. doi: 10.1007/s10554-018-1461-7
212. Korosoglou G, Giusca S, Hofmann NP, Patel AR, Lapinskas T, Pieske B, et al. Strain-encoded magnetic resonance: A method for the assessment of myocardial deformation. ESC Heart Fail (2019) 6(4):584–602. doi: 10.1002/ehf2.12442
213. Ida S, Kaneko R, Murata K. Effects of oral antidiabetic drugs on left ventricular mass in patients with type 2 diabetes mellitus: A network meta-analysis. Cardiovasc Diabetol (2018) 17(1):129. doi: 10.1186/s12933-018-0773-1
214. Borghetti G, von Lewinski D, Eaton DM, Sourij H, Houser SR, Wallner M. Diabetic cardiomyopathy: Current and future therapies. Beyond Glycemic Control. Front Physiol (2018) 9:1514. doi: 10.3389/fphys.2018.01514
215. Bonaventura A, Carbone S, Dixon DL, Abbate A, Montecucco F. Pharmacologic strategies to reduce cardiovascular disease in type 2 diabetes mellitus: Focus on sglt-2 inhibitors and glp-1 receptor agonists. J Internal Med (2019) 286(1):16–31. doi: 10.1111/joim.12890
216. Marso SP, Daniels GH, Brown-Frandsen K, Kristensen P, Mann JFE, Nauck MA, et al. Liraglutide and cardiovascular outcomes in type 2 diabetes. New Engl J Med (2016) 375(4):311–22. doi: 10.1056/NEJMoa1603827
217. Hiramatsu T, Asano Y, Mabuchi M, Imai K, Iguchi D, Furuta S. Liraglutide relieves cardiac dilated function than dpp-4 inhibitors. Eur J Clin Invest (2018) 48(10):e13007. doi: 10.1111/eci.13007
218. Holman RR, Bethel MA, Mentz RJ, Thompson VP, Lokhnygina Y, Buse JB, et al. Effects of once-weekly exenatide on cardiovascular outcomes in type 2 diabetes. New Engl J Med (2017) 377(13):1228–39. doi: 10.1056/NEJMoa1612917
219. Jorsal A, Kistorp C, Holmager P, Tougaard RS, Nielsen R, Hänselmann A, et al. Effect of liraglutide, a glucagon-like peptide-1 analogue, on left ventricular function in stable chronic heart failure patients with and without diabetes (Live)-a multicentre, double-blind, randomised, placebo-controlled trial. Eur J Heart failure (2017) 19(1):69–77. doi: 10.1002/ejhf.657
220. Wiviott SD, Raz I, Bonaca MP, Mosenzon O, Kato ET, Cahn A, et al. Dapagliflozin and cardiovascular outcomes in type 2 diabetes. New Engl J Med (2019) 380(4):347–57. doi: 10.1056/NEJMoa1812389
221. Neal B, Perkovic V, Mahaffey KW, de Zeeuw D, Fulcher G, Erondu N, et al. Canagliflozin and cardiovascular and renal events in type 2 diabetes. New Engl J Med (2017) 377(7):644–57. doi: 10.1056/NEJMoa1611925
222. Zinman B, Wanner C, Lachin JM, Fitchett D, Bluhmki E, Hantel S, et al. Empagliflozin, cardiovascular outcomes, and mortality in type 2 diabetes. New Engl J Med (2015) 373(22):2117–28. doi: 10.1056/NEJMoa1504720
223. Singh AK, Singh R. Gender difference in cardiovascular outcomes with sglt-2 inhibitors and glp-1 receptor agonist in type 2 diabetes: A systematic review and meta-analysis of cardio-vascular outcome trials. Diabetes Metab syndrome (2020) 14(3):181–7. doi: 10.1016/j.dsx.2020.02.012
224. Zelniker TA, Wiviott SD, Raz I, Im K, Goodrich EL, Furtado RHM, et al. Comparison of the effects of glucagon-like peptide receptor agonists and sodium-glucose cotransporter 2 inhibitors for prevention of major adverse cardiovascular and renal outcomes in type 2 diabetes mellitus. Circulation (2019) 139(17):2022–31. doi: 10.1161/CIRCULATIONAHA.118.038868
225. Vaduganathan M, Claggett BL, Jhund PS, Cunningham JW, Pedro Ferreira J, Zannad F, et al. Estimating lifetime benefits of comprehensive disease-modifying pharmacological therapies in patients with heart failure with reduced ejection fraction: A comparative analysis of three randomised controlled trials. Lancet (London England) (2020) 396(10244):121–8. doi: 10.1016/S0140-6736(20)30748-0
226. Solomon SD, Zile M, Pieske B, Voors A, Shah A, Kraigher-Krainer E, et al. The angiotensin receptor neprilysin inhibitor Lcz696 in heart failure with preserved ejection fraction: A phase 2 double-blind randomised controlled trial. Lancet (London England) (2012) 380(9851):1387–95. doi: 10.1016/S0140-6736(12)61227-6
227. Jhund PS, Fu M, Bayram E, Chen C-H, Negrusz-Kawecka M, Rosenthal A, et al. Efficacy and safety of Lcz696 (Sacubitril-valsartan) according to age: Insights from paradigm-hf. Eur Heart J (2015) 36(38):2576–84. doi: 10.1093/eurheartj/ehv330
228. Solomon SD, McMurray JJV, Anand IS, Ge J, Lam CSP, Maggioni AP, et al. Angiotensin-neprilysin inhibition in heart failure with preserved ejection fraction. New Engl J Med (2019) 381(17):1609–20. doi: 10.1056/NEJMoa1908655
229. Seferovic JP, Claggett B, Seidelmann SB, Seely EW, Packer M, Zile MR, et al. Effect of Sacubitril/Valsartan versus enalapril on glycaemic control in patients with heart failure and diabetes: A post-hoc analysis from the paradigm-hf trial. Lancet Diabetes Endocrinol (2017) 5(5):333–40. doi: 10.1016/S2213-8587(17)30087-6
230. Armstrong PW, Pieske B, Anstrom KJ, Ezekowitz J, Hernandez AF, Butler J, et al. Vericiguat in patients with heart failure and reduced ejection fraction. New Engl J Med (2020) 382(20):1883–93. doi: 10.1056/NEJMoa1915928
231. Armstrong PW, Lam CSP, Anstrom KJ, Ezekowitz J, Hernandez AF, O'Connor CM, et al. Effect of vericiguat vs placebo on quality of life in patients with heart failure and preserved ejection fraction: The vitality-hfpef randomized clinical trial. Jama (2020) 324(15):1512–21. doi: 10.1001/jama.2020.15922
232. Senni M, Alemayehu WG, Sim D, Edelmann F, Butler J, Ezekowitz J, et al. Efficacy and safety of vericiguat in patients with heart failure with reduced ejection fraction treated with Sacubitril/Valsartan: Insights from the Victoria trial. Eur J Heart failure (2022) 24(9):1614–22. doi: 10.1002/ejhf.2608
233. Swedberg K, Komajda M, Böhm M, Borer JS, Ford I, Dubost-Brama A, et al. Ivabradine and outcomes in chronic heart failure (Shift): A randomised placebo-controlled study. Lancet (London England) (2010) 376(9744):875–85. doi: 10.1016/S0140-6736(10)61198-1
234. Fox K, Ford I, Steg PG, Tendera M, Robertson M, Ferrari R. Heart rate as a prognostic risk factor in patients with coronary artery disease and left-ventricular systolic dysfunction (Beautiful): A subgroup analysis of a randomised controlled trial. Lancet (London England) (2008) 372(9641):817–21. doi: 10.1016/S0140-6736(08)61171-X
235. Komajda M, Tavazzi L, Francq BG, Böhm M, Borer JS, Ford I, et al. Efficacy and safety of ivabradine in patients with chronic systolic heart failure and diabetes: An analysis from the shift trial. Eur J Heart failure (2015) 17(12):1294–301. doi: 10.1002/ejhf.347
236. Komajda M, Isnard R, Cohen-Solal A, Metra M, Pieske B, Ponikowski P, et al. Effect of ivabradine in patients with heart failure with preserved ejection fraction: The edify randomized placebo-controlled trial. Eur J Heart failure (2017) 19(11):1495–503. doi: 10.1002/ejhf.876
237. Malik FI, Hartman JJ, Elias KA, Morgan BP, Rodriguez H, Brejc K, et al. Cardiac myosin activation: A potential therapeutic approach for systolic heart failure. Science (2011) 331(6023):1439–43. doi: 10.1126/science.1200113
238. Teerlink JR, Diaz R, Felker GM, McMurray JJV, Metra M, Solomon SD, et al. Cardiac myosin activation with omecamtiv mecarbil in systolic heart failure. New Engl J Med (2021) 384(2):105–16. doi: 10.1056/NEJMoa2025797
239. Biering-Sørensen T, Minamisawa M, Claggett B, Liu J, Felker GM, McMurray JJV, et al. Cardiac myosin activator omecamtiv mecarbil improves left ventricular myocardial deformation in chronic heart failure: The cosmic-hf trial. Circ Heart failure (2020) 13(12):e008007. doi: 10.1161/CIRCHEARTFAILURE.120.008007
240. Pouleur A-C, Knappe D, Shah AM, Uno H, Bourgoun M, Foster E, et al. Relationship between improvement in left ventricular dyssynchrony and contractile function and clinical outcome with cardiac resynchronization therapy: The madit-crt trial. Eur Heart J (2011) 32(14):1720–9. doi: 10.1093/eurheartj/ehr185
241. Cleland JGF, Teerlink JR, Senior R, Nifontov EM, Mc Murray JJV, Lang CC, et al. The effects of the cardiac myosin activator, omecamtiv mecarbil, on cardiac function in systolic heart failure: A double-blind, placebo-controlled, crossover, dose-ranging phase 2 trial. Lancet (London England) (2011) 378(9792):676–83. doi: 10.1016/S0140-6736(11)61126-4
242. Lewis GD, Voors AA, Cohen-Solal A, Metra M, Whellan DJ, Ezekowitz JA, et al. Effect of omecamtiv mecarbil on exercise capacity in chronic heart failure with reduced ejection fraction: The meteoric-hf randomized clinical trial. Jama (2022) 328(3):259–69. doi: 10.1001/jama.2022.11016
243. Jellis CL, Sacre JW, Wright J, Jenkins C, Haluska B, Jeffriess L, et al. Biomarker and imaging responses to spironolactone in subclinical diabetic cardiomyopathy. Eur Heart J Cardiovasc Imaging (2014) 15(7):776–86. doi: 10.1093/ehjci/jeu013
244. Heidenreich PA, Bozkurt B, Aguilar D, Allen LA, Byun JJ, Colvin MM, et al. Aha/Acc/Hfsa guideline for the management of heart failure: A report of the American college of Cardiology/American heart association joint committee on clinical practice guidelines. Circulation (2022) 145(18):e895–e1032. doi: 10.1161/CIR.0000000000001063
Keywords: diabetic cardiomyopathy, phase of progression, screening, diagnosis, treatment, review
Citation: Zhao X, Liu S, Wang X, Chen Y, Pang P, Yang Q, Lin J, Deng S, Wu S, Fan G and Wang B (2022) Diabetic cardiomyopathy: Clinical phenotype and practice. Front. Endocrinol. 13:1032268. doi: 10.3389/fendo.2022.1032268
Received: 30 August 2022; Accepted: 22 November 2022;
Published: 07 December 2022.
Edited by:
Sarah Costantino, University of Zurich, SwitzerlandReviewed by:
Oscar Lorenzo, Health Research Institute Foundation Jimenez Diaz (IIS-FJD), SpainAkshyaya Pradhan, King George Medical Univeirsty, India
Copyright © 2022 Zhao, Liu, Wang, Chen, Pang, Yang, Lin, Deng, Wu, Fan and Wang. This is an open-access article distributed under the terms of the Creative Commons Attribution License (CC BY). The use, distribution or reproduction in other forums is permitted, provided the original author(s) and the copyright owner(s) are credited and that the original publication in this journal is cited, in accordance with accepted academic practice. No use, distribution or reproduction is permitted which does not comply with these terms.
*Correspondence: Bin Wang, robin_e@sina.com; Guanwei Fan, fgw1005@hotmail.com
†These authors have contributed equally to this work and share first authorship