- 1Department of Zoology, University of Jammu, Jammu, India
- 2Department of Zoology, DAV University, Jalandhar, India
- 3Natural Products and Medicinal Chemistry Division, Council of Scientific and Industrial Research (CSIR)-Indian Institute of Integrative Medicine, Jammu, India
- 4Academy of Scientific and Innovative Research (AcSIR), Ghaziabad, India
- 5Department of Horticulture, Rajmata Vijayaraje Scindia Krishi Vishwa Vidyalaya, Gwalior, India
Phylum Nematoda is of great economic importance. It has been a focused area for various research activities in distinct domains across the globe. Among nematodes, there is a group called entomopathogenic nematodes, which has two families that live in symbiotic association with bacteria of genus Xenorhabdus and Photorhabdus, respectively. With the passing years, researchers have isolated a wide array of bioactive compounds from these symbiotically associated nematodes. In this article, we are encapsulating bioactive compounds isolated from members of the family Heterorhabditidae inhabiting Photorhabdus in its gut. Isolated bioactive compounds have shown a wide range of biological activity against deadly pathogens to both plants as well as animals. Some compounds exhibit lethal effects against fungi, bacteria, protozoan, insects, cancerous cell lines, neuroinflammation, etc., with great potency. The main aim of this article is to collect and analyze the importance of nematode and its associated bacteria, isolated secondary metabolites, and their biomedical potential, which can serve as potential leads for further drug discovery.
Introduction
Bioactive compounds represent substances having biological activity, which mediates some metabolic process leading to better health (Solomon and William, 2003; Bender, 2009). Nature is a reservoir of new bioactive compounds also known as natural products (NPs), whose study is indispensable for drug discovery and development (Ebada et al., 2008; Shen, 2015). These NPs are produced by plants, microorganisms, and animals (Baker et al., 2000) and symbiotic microorganisms. In developing countries, these NPs play an intrinsic role in the life of human beings substituting medicines due to easy availability and low cost. These NPs display a wide range of structural diversity, which correlates with biological activity like antitumor agents and enzyme inhibitors, antibiotics, immunosuppressive agents, growth promoters, herbicides, insecticides, and antiparasitic agents (Carmichael, 1992; Méndez and Salas, 2001). More than 300,000 NPs exist in literature and are mostly classified into five broader categories including alkaloids, steroids, terpenoids, polyketides, and fatty acid-derived substances, shikimate-derived compounds, and non-ribosomal polypeptides (Méndez and Salas, 2001). Among 10,000 biologically active compounds, almost 8,000 are antitumor and antibiotic agents (Brusotti et al., 2014). Since 1928, the year of penicillin discovery, around 20 different classes of antibiotics have been routed to the market (Coates et al., 2002; Powers, 2004). Most of the classes were explored from 1940 to 1962 and protected us from various infections for around 50 years (Coates et al., 2011). Recently, it has been reported that there are around 450,000 NPs out of which 70% are from plant origin (Ntie-Kang and Svozil, 2020). Over the years, the race to explore new biotics for controlling diseases is countered by pathogen resistance following Darwin’s principle. Utility of bioactive compounds in diverse commercial sectors like food, pharmaceutical, and chemical industries indicates the need to explore novel sources of bioactives.
Different living organisms produce a wide array of natural products. They are reported from both eukaryotic and prokaryotic (Prokaryotae, Monera) organisms but the ability to produce secondary metabolites is not uniform in all the species. Unicellular bacteria, filamentous actinomyces, and fungi frequently produce a wide array of secondary metabolites. Over few decades, the races to isolate useful molecules have shown established animal kingdom as a new and rich source of bioactive metabolites. These have been reported from a few marine invertebrates from Porifera, Cnidaria, Anthozoa, Tunicates, Mollusca, Echinodermata, etc. (Cabeza et al., 2021; Chamika et al., 2021; Mayefis and Widiastuti, 2021; Ramesh et al., 2021; Riccio et al., 2021; Sibiya et al., 2021; Singh et al., 2021; Tsvetkov et al., 2021; Wewengkang et al., 2021). The number of bioactive compounds from marine sources has been increasing linearly, i.e., 25 in 1972, 300 in 1982, 1,500 in 1992, and over 6,000 today, and more than 3,300 compounds are reported from sponges only (Bérdy, 2005). Besides this, certain animal microbes (like protozoa and ciliates), worms, insects, amphibians, and some higher vertebrates have been reported to produce bioactive compounds raising their number above 43,000. It includes antimicrobial, antitumor, products from marine sources, and microbial metabolites (Bérdy, 2005; Abd-Elgawad, 2021). In this review, we are presenting the genus Heterorhabditis, a less explored source of bioactive compounds from the gut of entomopathogenic nematode (EPNs), i.e., Heterorhabditidae. This review covers the total number of secondary metabolites reported from genus Heterorhabditis till date with their biomedical potential (Stock et al., 2017; Sivaramakrishnan and Razia, 2021).
Symbiotic Association of Photorhabdus With Heterorhabditis
Photorhabdus belongs to the family Enterobacteriaceae and is a Gram-negative bacterium symbiotically associated with the gut of Heterorhabditis, an entomopathogenic nematode (Forst et al., 1997). It is the only well-known terrestrial bioluminescent bacterium reported from the gut of IJs (infective juveniles) in EPNs. In the case of Heterorhabditidae, IJs do ambush, and they do not move in search of host and enter its body through natural openings (Figure 1). Once, it reaches the blood system of the host, the IJs regurgitates 50–200 bacterial cells (Ciche and Ensign, 2003) to conquer the immune system of the host and kill the host with septicemia. The IJs reproduce and multiply a few generations inside the host. The dead host raises the challenge of the Photorhabdus as it has to successfully compete with saprophytic scavengers, such as protists, other bacteria, nematodes, fungus, and even insects (Waterfield et al., 2009). In response, this symbiont produces a wide range of bioactives to cope up with the challenges for successful establishment and survival (Lulamba et al., 2021). Based on phenotypic characterization and DNA relatedness, Photorhabdus has been classified broadly into three species, i.e., Photorhabdus luminescens, Photorhabdus asymbiotica, and Photorhabdus temperata. This classification has been confirmed through limited microarray analysis (Marokhazi et al., 2003), genomic studies, and multilocus sequence typing (Duchaud et al., 2003; Gerrard et al., 2004). More recently, Machado et al. (2018) have proposed Photorhabdus subspecies to the species level and described one novel Photorhabdus bodei sp. and new Photorhabdus laumonii subspecies based on whole genome study. Photorhabdus species are facultative anaerobic and highly motile rods (Peel et al., 1999). All species produce a unique thin line of annular hemolysis on blood agar and grow well at 28°C. The clinical isolates grow from a temperature range of 37–42°C (Hapeshi et al., 2020). All the strains show bioluminescence on both liquid medium and agar plates, which peaks at exponential phase. The development of Heterorhabditis requires exogenous sterols (Chitwood, 1999), which are provided by the Photorhabdus to ensure successful symbiosis. Photorhabdus produces iso-branched fatty acids (BCFAs) through bkdABC operon (Joyce et al., 2008) for the nematode partner. For nematode nutrition, Photorhabdus produces CipA, CipB (crystalline inclusion proteins), and secondary metabolites, such as stilbene (ST), called 3,5-dihydroxy-4-isopropylstilbene (Joyce et al., 2008). Photorhabdus is the only non-plant organism that produces STs, a polyketide molecule. The biochemical pathway for the production of ST is different from that of plants and is well characterized (Williams et al., 2005; Joyce et al., 2008). InPtNC19, phosphopantethienyl (PPANT) transferase (ngrA gene) is vital for secondary metabolite synthesis, as well as nematode growth and reproduction (Ciche et al., 2001). In Photorhabdus, almost 6% genome is occupied by genes involved in secondary metabolite production, which is much greater than that of Streptomyces, the model organism for the production of secondary metabolite (Duchaud et al., 2003). Streptomyces uses 3.8% genome only and is a source of more than 90% clinically important antibiotics (Waterfield et al., 2009). This emphasizes the significant potential of Photorhabdus as a source of novel bioactive compounds. Studies have reported that Photorhabdus expresses two virulence factors, i.e., Tca (toxin complex A) and PrtA (metalloprotease) (Daborn et al., 2001; Silva et al., 2002). Recently, studies have also reported antimicrobial activities of compounds isolated from Photorhabdus species strain ETL in association with Heterorhabditis zealandica (Lulamba et al., 2021).
An overview of general mode of action of these compounds against different insect pest is shown in Figure 2.
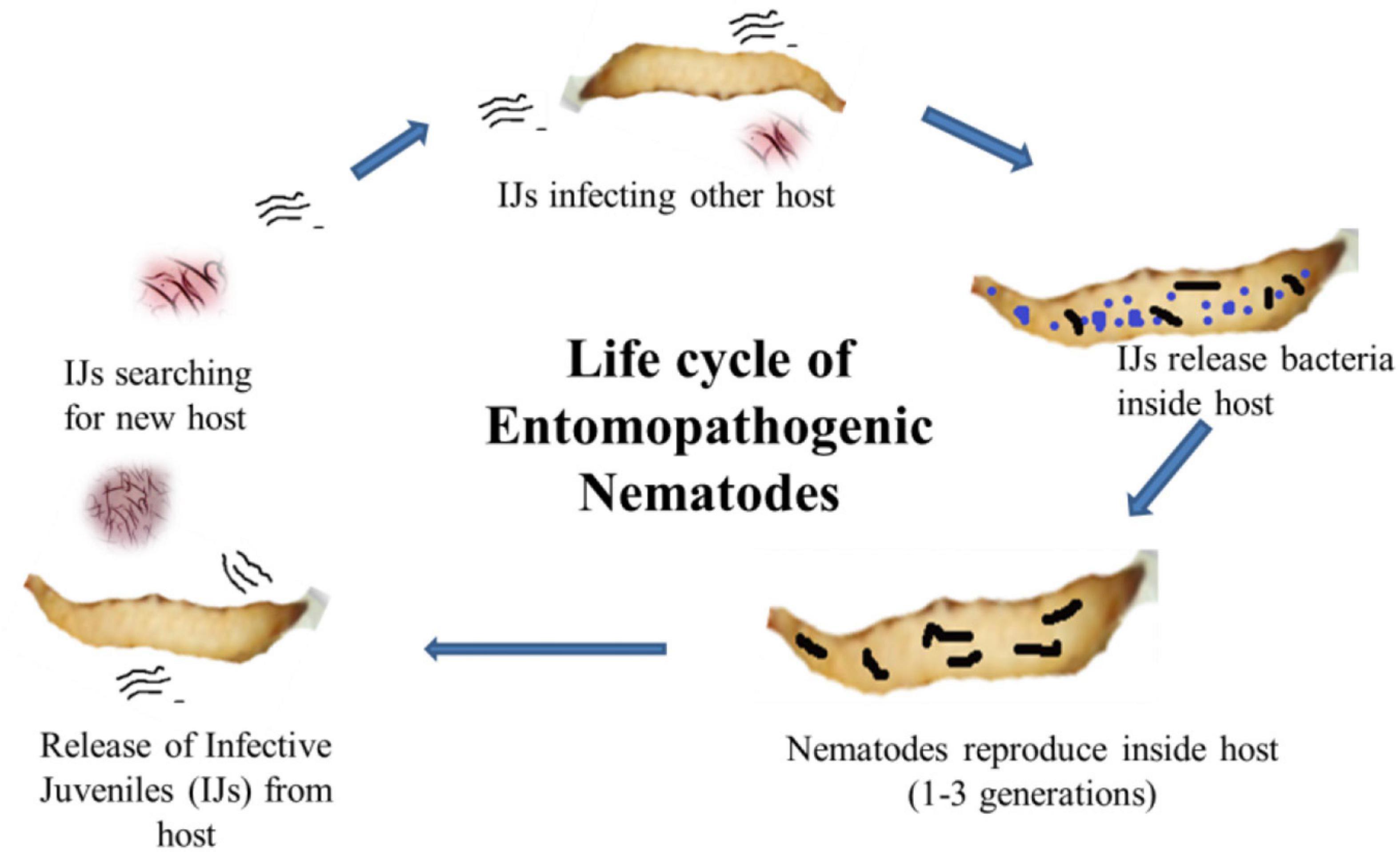
Figure 1. Life cycle of entomopathogenic nematodes showing infective juveniles (IJs) enter into the host body and release symbiotic bacteria from the gut to kill the host. The nematode reproduces inside the dead cadaver, and IJs are released into the soil in search of a new host.
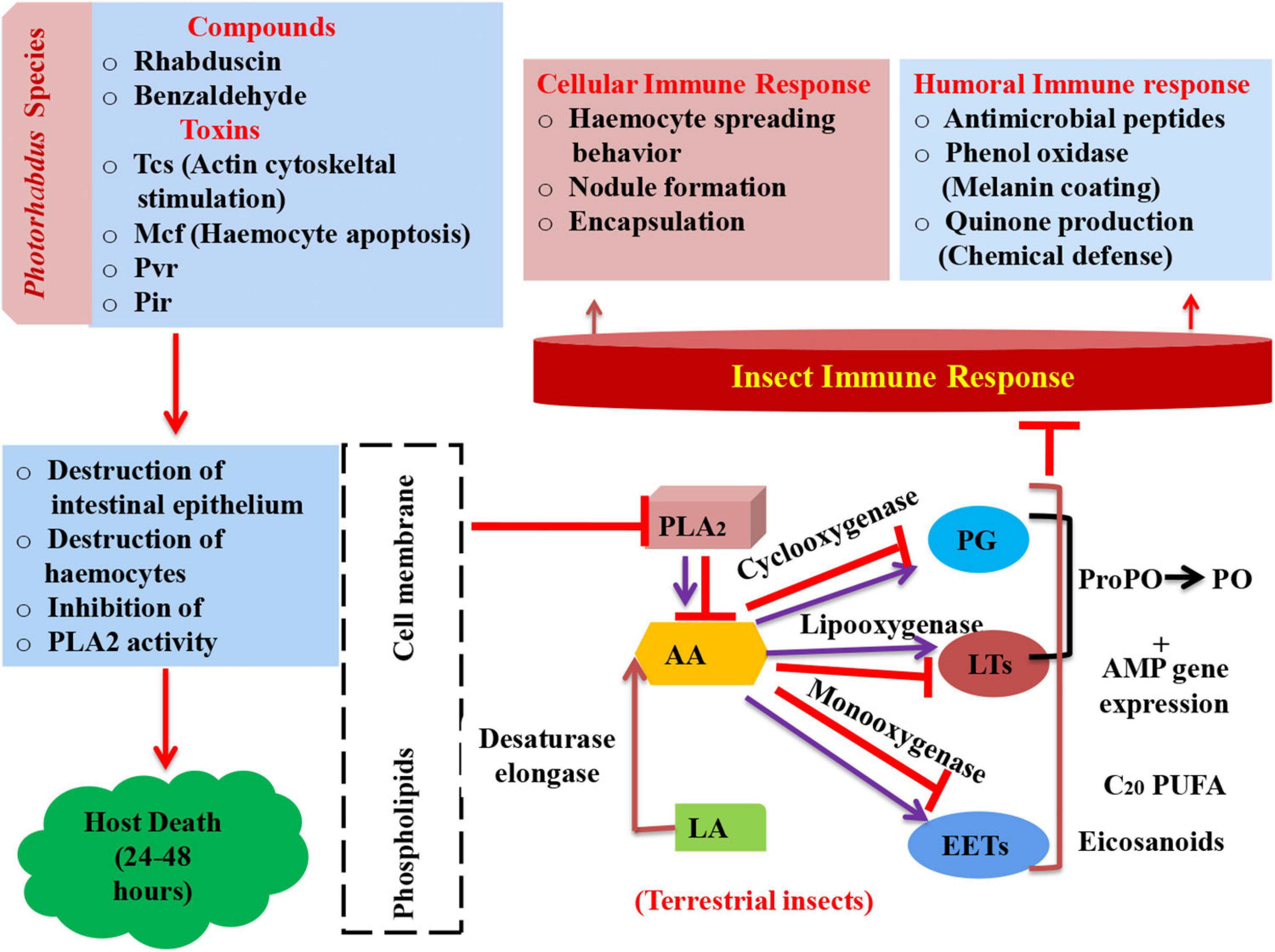
Figure 2. Schematic representation of insect’s immune response and mode of action of Photorhabdus compounds in arresting immune system leading to mortality (PLA2, phospholipase A2; AA, arachidonic acid; LA, linoleic acid; PG, prostaglandin; LTs, leukotrienes; EETs, epoxyeicosatrienoic acids; ProPO, prophenoloxidase; AMP, antimicrobial peptides; PO, phenol oxidase; Tcs, toxin complexes; Mcf, make caterpillars floppy; Pvc, Photorhabdus virulence cassettes; Pir, insect-related protein).
Photorhabdus as Source of Antiprotozoal Compounds
Protozoal parasitism is widespread among cats, dogs, and even humans (Baneth and Solano, 2020). Protozoans have caused a global health problem with diseases, such as malaria, giardiasis, trichomoniasis, trypanosomiasis, and leishmaniasis. Major disease-causing protozoans are Leishmania mexicana, Trypanosoma cruzi, Plasmodium falciparum, Trichomonas vaginalis, and Giardia intestinalis (Lee et al., 2019). These parasites spread worldwide infection due to poor sanitary and unhygienic conditions in developing countries (Garcia et al., 2003; Renslo and McKerrow, 2006; Pozio, 2007). About 200 million people get infected with malaria due to Plasmodium, and about half a million die annually (Ashley et al., 2018). In 2018, WHO reported 99.7% malarial cases in African region, 50% in the South-East Asia Region, 71% in the Eastern Mediterranean, and 65% in the Western Pacific due to Plasmodium falciparum. The WHO reports that in 2019, 12% cases of Trypanosoma brucei rhodesiense were endemic to 13 countries of southern and eastern Africa. Globally, 6–7 million people are infected with Trypanosoma cruzi, a protozoal parasite that causes deadly disease, such as American trypanosomiasis or Chagas disease (World Health Organization [WHO], 2018, 2019). This shows that antiprotozoal drugs lose efficiency to control the disease due to drug resistance and its toxicity. This has necessitated the need to find new source of antiprotozoal drugs. In this race, Photorhabdus, a nematode symbiont, has emerged as a reliable source of antiprotozoal compounds. Some of the antiprotozoal bioactive compounds isolated from Photorhabdus are given below.
Phototemtide A (1)
A new cyclic lipopeptide, phototemtide A (1), was isolated from Escherichia coli expressing the biosynthetic gene cluster pttABC from Photorhabdus temperata Meg1. This new cyclic lipopeptide has three more minor derivatives. It has been reported that this peptide has weak antiprotozoal activity with IC50 = 9.8 μM, against deadly plasmodium species Plasmodium falciparum (Zhao et al., 2020). New peptide drugs have gained attention due to their easy synthesis, low toxicity, fewer side effects, and rapid elimination (Du et al., 2015). The quantity of market active constituents is very less due to unpleasant side effects and observed resistance (Weinke et al., 1991; Steketee et al., 1996; Plowe, 2005; Uhlemann and Krishna, 2005). This compound is selectively effective against Plasmodium falciparum and maybe a potential antiprotozoal alternative in the future.
Photoditritide (2)
A cyclic peptide photoditritide, which was isolated from Photorhabdus temperata Meg1, which contains two rare amino acid D-homoarginine residues and encoded by pdtS gene. The gene pdtS codes for non-ribosomal peptide synthetase having six modules with 18 domains in all. It showed weak antiprotozoal activity against causative agent of African sleeping sickness, i.e., Trypanosoma brucei rhodesiense with IC50 = 13 μM (Zhao et al., 2019). Peptides containing homoarginine, a non-proteinogenic amino acid, have been reported from many marine organisms, such as cyanobacteria, actinomycetes, and sponge, but photoditritide is the only example of peptide containing homoarginine derived from entomopathogenic bacteria (Bonnington et al., 1997; Saito et al., 2001; Cha et al., 2012; Zhao et al., 2019).
Isopropylstilbene
This is a class of natural products produced by Photorhabdus luminescens TT01. Many derivatives of this class have been synthesized by modifying PAL gene and antB gene in Photorhabdus luminescens mutant (BMM901), such as cyclohexanedione (CHD) and dialkylresorcinol (DAR) derivatives (Joyce et al., 2008; Kronenwerth et al., 2014). The biosynthesis of isopropylstilbene from Photorhabdus varies from plant stilbene biosynthesis, whereby two acyl moieties become condensed to form a resorcinol ring (Ferrer et al., 2008; Joyce et al., 2008; Fuchs et al., 2013). Synthetic derivatives, such as 12–14 and 1 and 6, were reported to be more effective against Trypanosoma cruzi (causes Chagas disease) and Plasmodium falciparum (causes malaria), respectively. Some chemically synthesized derivatives were more active against Trypanosoma cruzi (LC50 = 8.80 μM) and Leishmania donovani (LC50 = 3.71 μM) (Kronenwerth et al., 2014). This potent class of antiprotozoal compounds can meet the needs of pharmaceutical sector in the future.
Kolossin A (3)
Photorhabdus luminescens, an entomopathogenic bacterium bears a flag of producing the largest and continuous non-ribosomal peptide synthetase among bacteria (Bode et al., 2015). It possesses a fully functional uninterrupted gene that could produce 15 consecutive modules encoded by kol gene (plu2670, 49.1 kbp). Many gene clusters of its genome are involved in biosynthesis of diverse natural products (Duchaud et al., 2003; Yin et al., 2015). Photorhabdus luminescens produces kolossin A, a d-/l-pentadecapeptide biosynthetic product of non-ribosomal peptide synthetase. It has been reported that stereoisomer of Kolossin A, displays high activity against the causative agent of African sleeping sickness, i.e., Trypanosoma brucei rhodesiense (IC50 = 2.7μM), Plasmodium falciparum (IC50 = 16.1μM), and T. brucei r. (IC50 = 8.9μM) (Bode et al., 2015). This class of antiprotozoal compounds can prove to be useful for drug discovery against deadly protozoal diseases in the near future (Figure 3 and Table 1).
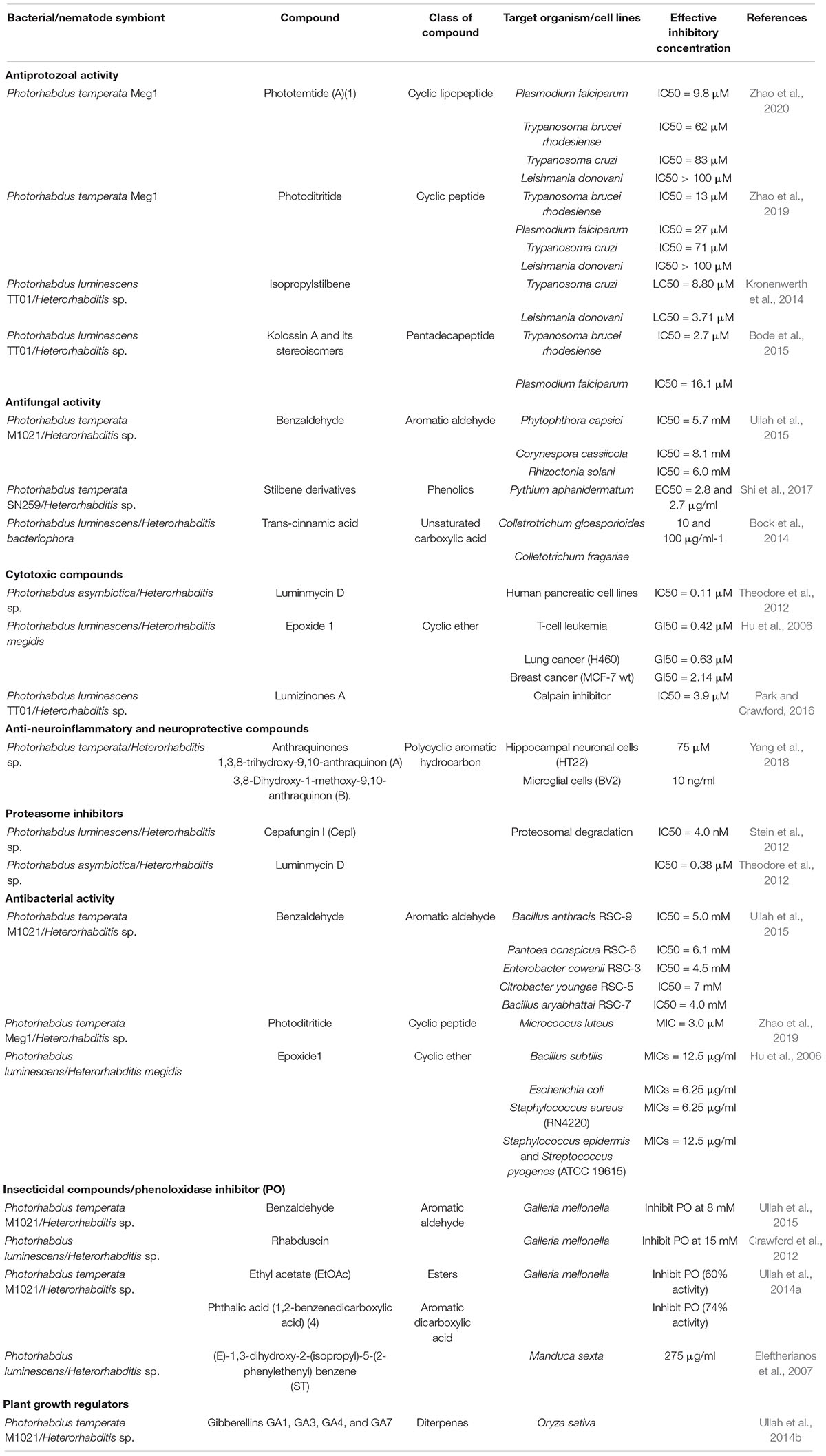
Table 1. List of bioactive compounds isolated from different species of Heterorhabditis and Photorhabdus.
Photorhabdus as Source of Antifungal Compounds
Many fungal strains cause huge losses to crops, such as cereals and vegetables, and pose a serious threat to food security across the globe (Savary et al., 2006). Postharvest losses to vegetables and fruits due to many fungal pathogens lead to rotten crops and mycotoxin production to harm animals as well as humans (Saremi and Okhovvat, 2006; Bai et al., 2013). Huge loss to the crop has been caused by Fusarium oxysporum and Pythium aphanidermatum due to postharvest decay (Saremi and Okhovvat, 2006). Studies have reported antifungal activity of different compounds produced by entomopathogenic nematodes (Cimen et al., 2021). Some antifungal compounds produced by Photorhabdus species are listed below.
Benzaldehyde (4)
Photorhabdus Temperata M1021 Produces Benzaldehyde as an Insecticidal, Antimicrobial, and Antioxidant Compound.
Antimicrobial activity was assessed by MIC values ranging from 6 to 10 mM for bacterial strains and 6–10 mM for fungal strains, i.e., Phytophthora capsici (IC50 = 5.7 mM), Corynespora cassiicola (IC50 = 8.1 mM), and Rhizoctonia solani (IC50 = 6.0 mM) (Ullah et al., 2015). Studies claim that the interaction of benzaldehyde with the cell surface triggers cell membrane disintegration and intracellular constituent release, which leads to cell death (Cheng et al., 2009).
Stilbene Derivatives (5–9)
These classes of compounds are phenolics. It has been reported that seven derivatives of stilbene, i.e., 3-hydroxy-2-isopropyl-5-phenethylphenyl carbamate,2-(1-hydroxypropan-2-yl)-5-[(E)-2-phenylethenyl]benzene-1,3-diol, 2-(1-hydroxypropan-2-yl)-5-[2-phenylethyl]benzene-1,3-diol, 2-ethyl-5-(2-phenylethyl) benzene-1,3-diol, 2-isopropyl-5-[2-phenylethyl]benzene-1, 3-diol, 2-isopropyl-5- [(E)-2-phenylethenyl]benzene-1,3-diol, and 2-ethyl-5-[(E)-2-phenylethenyl]benzene-1,3-diol showed antifungal activity against four phytopathogenic fungi, such as Rhizoctonia solani Kuhn, Pythium aphanidermatum, Fusarium oxysporum, and Exserohilum turcicum (Paul et al., 1981; Fuchs et al., 2013). Out of these seven derivatives, 3-hydroxy-2-isopropyl-5-phenethylphenyl carbamate strongly inhibits Pythium aphanidermatum mycelium at EC50 = 2.8 and 2.7 μg/ml, respectively. The presence of acyl amino group in the former and isopropyl group in the latter contributed to inhibitory activity of these compounds.
Trans-Cinnamic Acid
This class of compound is a small molecule possessing antibiotic properties and food preservative (US patent doc numbers 6036986; 6042861) (Si et al., 2006; Wong et al., 2008; Chen et al., 2011; Hakkim et al., 2012). It has been reported that TCA is a necessary precursor for biosynthesis of the antibiotic stilbene (Williams et al., 2005; Eleftherianos et al., 2007; Chalabaev et al., 2008). Studies have established that TCA produced by Photorhabdus luminescens shows antimycotic activity against two important plant pathogens belonging to two fungal genera Colletotrichum and Fusicladium. In vitro studies have confirmed the toxicity of TCA against Colletotrichum gloeosporioides, Colletotrichum acutatum, Colletotrichum fragariae, and Fusicladium effusum (Bock et al., 2014). Recently, researchers have discovered phenylalanine ammonia-lyase (PAL) gene from Photorhabdus luminescens DSM 3368, which has enhanced the value of this strain for the production of TCA at commercial level (Zhang et al., 2021; Figure 4 and Table 1).
Photorhabdus as Source of Cytotoxic Compounds
Cancer, the uncontrolled division of cells, claims more than 8 million lives annually and is the second leading cause of deaths globally (Tarver, 2012; Bray et al., 2013). Major cancer types reported amounts to 1.61 million (lung cancer), 1.38 million (breast cancer), and 1.23 million (colorectal cancers) (Ferlay et al., 2010). Among men, prostate, lung, colorectal, liver, and stomach cancer, whereas among women breast, lung, colorectal, thyroid, and cervical cancer are very common (World Health Organization [WHO], 2021). Pancreatic cancer is reported as highly lethal (Kamisawa et al., 2016). The hallmark features of cancer includes cancer cell ability to induce angiogenesis, evade apoptosis, replicate limitlessly, insensitivity to antigrowth signals, self-sufficient production of growth signals, tissue invasion, and metastasis (Hanahan and Weinberg, 2011; Merdad et al., 2014; Pérez, 2014; Snellenberg et al., 2014; Courtnay et al., 2015; Jiang et al., 2015; Mar et al., 2015; Frink et al., 2016).
Glidobactins (11–15)
This novel compound belongs to the luminmycin metabolite family and is produced by Photorhabdus asymbiotica in laboratory culture. Photorhabdus asymbiotica has a unique, biphasic lifestyle as both a symbiotic and pathogenic bacterium. Genomic analysis revealed several synthetic gene clusters capable of producing secondary metabolites. Photorhabdus asymbiotica can produce cytotoxic derivatives, such as glidobactin A, luminmycin D, and luminmycin A. These compounds show cytotoxicity against pancreatic cells (IC50 = 0.11 μM) and inhibit proteasome (IC50 = 0.38 μM) (Theodore et al., 2012). Sequencing and annotation of the Photorhabdus asymbiotica (ATCC43949) genome have also been reported (Wilkinson et al., 2009). Recently, studies have reported cepafungin 1 and (GLNPs) glidobactin A produced by Photorhabuds laumondii as potent anticancer agents (Zhao et al., 2021).
Epoxide 1
This novel compound epoxide 1, is also known as 2-isopropyl-5-(3-phenyl-oxiranyl)-benzene-1,3-diol. It was isolated from Galleria mellonella larvae infected with Photorhabdus luminescens C9-Heterorhabditis megidis 90 symbiont complexes (tripartite interaction, insect-nematode, and bacterium). Epoxide 1 has been derived from 2-isopropyl-5-(2-phenylethenyl)-benzene-1,3-diol (Hu et al., 1997, 1998). Epoxide 1 was active against Bacillus subtilis, Escherichia coli, Streptococcus pyogenes, and a drug-resistant, clinical strain of Staphylococcus aureus (RN4220) with minimum inhibitory concentrations in the range of 6.25–12.5 μg/ml. Epoxide 1 was cytotoxic against human cancer cell lines, MCF-7 wt, H460, and Jurkat, with GI (50) of 2.14, 0.63, and 0.42 μM, respectively, but was less toxic on normal, mouse splenic lymphocytes with a GI (50) of 45.00 μM (Hu et al., 2006).
Lumizinones A (16)
Lumizinones are produced exclusively from the pathogenic form, a phenotypic variant of Photorhabdus, which is associated with insect pathogenesis and nematode development. Photorhabdus. This compound inhibits calpain protease activity at IC50 = 3.9 μM (Park and Crawford, 2016). Calpain is a heterodimer having two subunits, i.e., regulatory subunit (30 kDa) and catalytic subunit (80 kDa). It belongs to the intracellular cysteine proteases family, Ca2+-dependent, and distributed in the cytoplasm of cells and tissues in eukaryotes (Sato and Kawashima, 2001; Goll et al., 2003; Park and Crawford, 2016). Calpains are associated with cancer like schwannomas and meningiomas, renal cell carcinoma, colorectal adenocarcinoma, squamous carcinomas of the skin, prostate cancer, endometrial cancer, uterine sarcomas and carcinosarcomas, uterine cervical, neoplasiamelanoma, gastric cancer, laryngeal, colorectal, and pancreatic cancer (Kimura et al., 1998; Braun et al., 1999; Yoshikawa et al., 2000; Mamoune et al., 2003; Reichrath et al., 2003; Rios-Doria et al., 2003; Lakshmikuttyamma et al., 2004; Frances et al., 2007; Lee et al., 2007, 2008; Moretti et al., 2009; Fong et al., 2010; Salehin et al., 2010; Moreno et al., 2011; Figure 5 and Table 1).
Photorhabdus as Source of Antineuroinflammatory and Neuroprotective Compounds
Anthraquinones (17–18)
These quinones are derived from anthracene and are widely used in cosmetics, food, dyes, and pharmaceuticals. Over the years, many anthraquinone derivatives have been identified from bacteria, plants, insects, and fungi having a wide array of bioactivity like anticancer, laxation, neuroprotective, antimalaria, and anti-inflammatory effects (Bonadonna et al., 1969; Monks et al., 1992; Van Gorkom et al., 2002; Pankewitz et al., 2007; Wang et al., 2007; Batista et al., 2009; Kim et al., 2009; Gessler et al., 2013; Lim et al., 2017; Wu et al., 2017).
Recently, Photorhabdus temperata has been reported as a source of anti-neuroinflammatory and neuroprotective drug leads, such as 1, 3, 8-trihydroxy-9, 10-anthraquinone, and 3, 8-dihydroxy-1-methoxy-9, 10-anthraquinone. An earlier compound has shown significant protection of hippocampal neuronal cells (HT22) at 75 μM in mouse against glutamate-induced cell death (5 mM) caused via lipid peroxidation, Ca2+ influx, and inhibiting reactive oxygen species production. Both the compounds have also been reported to suppress neuroinflammation induced by interferon γ in microglial cells (BV2) of mouse at a concentration of 10 ng/ml through reduction of interleukin-6, TNF (tumor necrosis factor-α), and nitric oxide (Yang et al., 2018; Figure 6 and Table 1).
Photorhabdus as Source of Proteasome Inhibitor Compounds
Proteasome is a proteolytic complex having hollow cylinder and single torus protein described by the Harris group in 1970 (Harris, 1971). It is responsible for ubiquitinated protein degradation and has been a ray of hope to stop proliferation of malignant cells and cancer/multiple myeloma (Voorhees et al., 2003; Shah and Orlowski, 2009; Crawford et al., 2011). The function of proteosome is associated with ATP-dependent degradation of intracellular proteins, specifically having a polyubiquitin chain (Ciehanover et al., 1978; Ciechanover, 1998). Proteosome inhibitors are short peptides having a covalently bonded group of atoms called pharmacophore, which binds to proteosome at its catalytic sites and inhibits proteasome function (Kisselev et al., 2012). It is useful in treating multiple myeloma (MM), characterized by accumulation of pathological clonal plasma cells in bone marrow (BM) and a huge amount of monoclonal immunoglobulin (Ig) (Kyle and Rajkumar, 2009). Photorhabdus luminescens has emerged as a new source of this remarkable compound as explained below.
Cepafungin I
It is a 12-member macrolactam ring system that is linked with a fatty acid tail, terminally branched, and having additional methyl moiety. This compound has been reported to be produced by Photorhabdus luminescens. This compound is similar to one of the strongest proteasome inhibitors Glidobactin A (GlbA) (Oka et al., 1988; Shoji et al., 1990; Groll et al., 2008).
Luminmycin D
Photorhabdus asymbiotica-produced compound luminmycin D is also a potent proteasome inhibitor at IC50 value equal to 0.38 μM (Theodore et al., 2012).
Photorhabdus as Source of Antibacterial Compounds
Benzaldehyde (4)
This is a simplest aromatic aldehyde made up of benzene ring and formyl components. Photorhabdus temperata M1021 has been reported to produce this compound (Ullah et al., 2015). This compound shows antimicrobial properties against Bacillus anthracis RSC-9 (IC50 = 5.0 mM), Pantoea conspicua RSC-6 (IC50 = 6.1 mM), Enterobacter cowanii RSC-3 (IC50 = 4.5 mM), Citrobacter youngae RSC-5 (IC50 = 7 mM), and Bacillus aryabhattai RSC-7 (IC50 = mM).
Photoditritide (2)
This compound has been reported from Photorhabdus temperata Meg1. This compound shows antimicrobial potential against bacterium Micrococcus luteus at an MIC value equal to 3.0 μM (Zhao et al., 2019).
Epoxide1
This compound has been isolated from Photorhabdus luminescens. Studies have reported that this compound (2-isopropyl-5-(3-phenyl-oxiranyl)-benzene-1, 3-diol) is active against Escherichia coli, Streptococcus pyogenes, Bacillus subtilis, and Staphylococcus aureus (RN4220) at an inhibitory concentration ranging from 6.25 to 12.5 μg/ml (Hu et al., 2006; Figures 3, 4 and Table 1).
Photorhabdus as a Source of Insecticidal Compounds and Phenoloxidase Inhibition
Benzaldehyde (4)
This is an insecticidal compound produced by Photorhabdus temperata M1021. Studies have shown that it caused 100% mortality in G. mellonella at 8 mM concentration. It is toxic to insects and inhibited PO activity from 15 to 80% at different concentrations (Ullah et al., 2015).
Rhabduscin (19)
This class of molecule is an amidoglycosyl- and vinyl-isonitrile-functionalized tyrosine derivative produced by Photorhabdus luminescens. This compound targets the innate immune system of the insect through a key component called phenoloxidase in G. mellonella and has emerged as a potential lead for the formation of insecticides (Crawford et al., 2012).
Phurealipids
This compound is a simple urea compound produced by Photorhabdus luminescens. Studies have shown that it inhibits juvenile hormone epoxide hydrolase (JHEH), which is an important enzyme in the growth and development of insects. This makes it a more suitable chemical with insecticidal properties (Nollmann et al., 2015b).
(E)-1,3-Dihydroxy-2-(Isopropyl)-5(2-Phenylethenyl) Benzene (ST)
This is a small molecule having antibiotic properties and is produced by Photorhabdus luminescens in both in vivo as well as in vitro conditions. Cinnamic acid is a precursor of ST and is catalyzed by an enzyme encoded by stlA gene, which is a Photorhabdus gene (Eleftherianos et al., 2007). Studies have shown that this compound shows a wide range of antimicrobial activity and defends dead insects from microbe invasion (Hu and Webster, 2000; Williams et al., 2005).
3,5-Dihydroxy4-Isopropylstilbene
This class of compound has been isolated from Photorhabdus luminescens in dead G. mellonella larvae. This compound minimizes the competition between different microbes by inhibiting the growth of a wide range of bacterium (Li et al., 1995; Hu et al., 1998). This hypothesis is well supported in in vitro studies, but in vivo shreds of evidence are very less and have been questioned by Dutky (1959, 1974), Paul et al. (1981), Akhurst (1982), Li et al. (1995), Forst and Nealson (1996) and Jarosz (1996).
Phthalic Acid (20)
This compound was isolated from Photorhabdus temperata and has shown reliable insecticidal activity against G. mellonella (Figure 7 and Table 1).
Mode of Action of Insecticidal Compounds
Phospholipase A2 (PLA2) plays a key role in imparting immunity to insects as it releases arachidonic acid (AA) via its catalytic activity from phospholipids (Burke and Dennis, 2009). AA acts as precursor molecule in synthesis of eicosanoid. Eicosanoids are oxygenated C20 polyunsaturated fatty acids. These eicosanoids are grouped into three main categories, namely, prostaglandins (PGs), epoxyeicosatrienoic acids (EETs), and leukotrienes (LTs) (Stanley, 2014). Cyclooxygenase, monooxygenases, and lipoxygenase oxygenate AA into PGs, EETs, and LTs, respectively (Stanley and Kim, 2019). However, in the case of terrestrial insects, linoleic acid (LA) is present, which becomes converted into AA with the help of elongase and desaturase enzymes (Hasan et al., 2019). Furthermore, PGs and LTs, in turn, activate phenol oxidase (PO) via formation of proPo and antimicrobial gene expression (AMP) (Shrestha and Kim, 2008; Shrestha et al., 2011). This PO catalyzes the process of melanization, which is a crucial event that leads to insect mortality (Castillo et al., 2011). Bioactive compounds rhabduscin have the ability to destruct the host’s epithelium and inhibit the catalytic activity of PLA2 (Figure 2).
Photorhabdus as Source of Plant Growth-Regulator Compounds
Gibberellins
Gibberellins represent a group of tetracyclic compounds, diterpenoid compounds consisting of four isoprene units having an ent-gibberellane ring skeleton. It plays an essential role in germination, stem elongation, flowering, dormancy, sex expression, induction of enzymes, and senescence of fruit and leaf. This compound has been reported to be produced by Photorhabdus temperata M1021, a symbiont of entomopathogenic nematodes. Various bioactive GAs reported from Photorhabdus temperata M1021 through GC/MS-SIM analyses are GA1, GA3, GA4, GA7, GA9, GA12, and GA20 (Ullah et al., 2014b). GAs initiate cellular totipotency, seed germination, and plant growth (Großelindemann et al., 1992; Hedden and Kamiya, 1997; Table 1).
Other Compounds
Polyketide Pigments
These pigments are secondary metabolites having carbonyl and methylene groups, or have precursors with this group. Studies have reported these compounds from Photorhabdus luminescens strain TT01 and linked these compounds with antibiotic activity (Ffrench-Constant et al., 2003).
Photorhabdicins—R-Type Pyocins (21)
Bacteriocin is a protein, which is produced by one species of bacteria to inhibit some other strain. Studies have shown that Photorhabdus aeruginosa produces R-type pyocins having modified tail fibers, which strongly bind to bacterial surfaces and advocate antibacterial activity (Eleftherianos, 2009). Photorhabdicins are compounds having structures similar to R-type pyocins, have been reported from K122 and W14 strains of Photorhabdus, and possess a vital role against microbes (Ffrench-Constant et al., 2003) (Figure 8).
Lumicins—S-Type Pyocins
This is another type of bacteriocin reported to be produced by the W14 strain of Photorhabdus luminescens (Sharma et al., 2002). It carries many killer proteins to counter the effect of many other bacteria strains (Sharma et al., 2002).
Carbapenem Antibiotics
It is a class of antibiotics produced via biosynthetic pathways in cephamycins, penicillins, and cephalosporins (Williamson et al., 1985; Bycroft et al., 1988). It was reported that Photorhabdus luminescens TT01 possess a group of eight genes (cpmH to cpmA), which are responsible for carbapenem-like antibiotic production. This compound was found effective against Escherichia coli, Enterobacter cloacae, and Klebsiella pneumonia (Derzelle et al., 2002).
Anthraquinone Metabolites
Many Anthraquinone (AQ) pigments have been reported from Photorhabdus (Richardson et al., 1988; Li et al., 1995). Two gene clusters (plu4186-plu4194) have been identified for the production of type II PKS, which is predicted to form an AQ heptaketide backbone (Duchaud et al., 2003). These compounds possess antibiotic potential (Richardson et al., 1988; Sztaricskai et al., 1992; Li et al., 1995; Brachmann et al., 2007). Two more anthraquinone derivatives, i.e., 1,3-dimethoxy-8-hydroxy-9,10-anthraquinone (major) and 3,8-dimethoxy-1-hydroxy9,10-anthraquinone (minor) have been reported from Photorhabdus luminescens (Li et al., 1995).
Hydroxystilbene Compounds
Among the hydroxystilbene family, 1,3-dihydroxy-2-(isopropyl)-5-(2-phenylethenyl) benzene (ST) is an important molecule. This is a small multifunctional compound produced by only one bacterium, i.e., Photorhabdus luminescens, and shows antibacterial properties (Akhurst, 1982; Richardson et al., 1988; Li et al., 1995; Williams et al., 2005). ST epoxide, another related compound having powerful antibiotic potential, has also been reported from Photorhabdus species (Hu et al., 2006). stlA gene is responsible for the production of stilbene antibiotic molecules (Williams et al., 2005). Reports of antibiotic, 3,5-dihydroxy-4-isopropylstilbene from Photorhabdus luminescens are also available (Li et al., 1995).
Cinnamic Acid
This compound is a precursor of the antibiotic 3,5-dihydroxy-4-isopropylstilbene (ST) (Williams et al., 2005; Eleftherianos et al., 2007). Antioxidant as well as antibacterial activities of this compound have been reported (Korkina, 2007). Photorhabdus luminescens studies have linked Hca enzyme with ST synthesis and CA utilization (Chalabaev et al., 2008).
Photobactin
This compound is a catechol-type siderophore produced by Photorhabdus luminescens having the structure 2-(2,3-dihydroxyphenyl)-5-methyl-4,5-dihydro-oxazole-4-carboxylic acid [4-(2,3-dihydroxybenzoylamino)-butyl]-amide (Ciche et al., 2003). Purified photobactin also shows antibiotic activity (Paul et al., 1981; Akhurst, 1982; Richardson et al., 1988).
The bacteria of the genus Photorhabdus is also a source of many compounds having unknown functions or multifunctions. Studies have reported new pentapeptides known as GameXPeptides A (22) from Photorhabdus luminescens TTO1. These peptides are of unknown nature (Nollmann et al., 2015a). In Photorhabdus asymbiotica PB68.1, a library of photohexapeptides (23) has been generated after activating phpS, which is a silent gene. The photohexapeptide compound belongs to the rare linear D-/L-peptide family, which also includes feglymycin, kolossin (A), and gramicidin A (Zhao and Bode, 2019; Figure 8 and Table 1).
Discussion and Conclusion
Natural products (NPs) possess enough structural complexity and scaffold diversity. Historically, NPs and their analogs have contributed a lot to the pharmacology sector. Inspite of this, NPs also possess challenges for drug discovery like technical barrier to isolation, characterization, screening, and optimization, which has a decline in their pursuit in the pharmaceutical industry since 1990s (Atanasov et al., 2021). Evolution has structurally optimized NPs to serve peculiar biological function, such as interaction (inter- and intraspecific competitions) and defense mechanisms, which emphasize their relevance in combating many diseases (Atanasov et al., 2015). Bioactive compound-rich NP pool covers a broad chemical space compared with a library of small synthetic molecules (Lachance et al., 2012). A wide array of these natural products produced by natural processes plays a vital role in symbiotic associations. Entomopathogenic nematodes possess a huge diversity and indicate a huge scope of research for natural products. The genus has attracted research interest in the past decade because it has emerged as a new group of biocontrol agents against pathogens of crop plants and as a new source of bioactive natural products. This article includes screening reports of bioactive compounds, which require further studies before clinical trials. Considering the current crisis in antibiotic resistance, the discovery of novel antibiotics is of great importance, and this association of Heterorhabditis and Photorhabdus might play a wider role in human survival in the twenty-first century.
However, there are still many aspects remaining to be studied. First, the genome sequencing of these bacteria revealed the presence of several predicted gene clusters. The exact roles of these are still unknown and need thorough investigation. So far, the main effort in this area has been devoted to isolation and structural determination. Second, the mining of these gene clusters with media manipulation and epigenetic approach for new natural products is still in its infant stage. Future research should set out to identify new selection markers, powerful promoters, and innovative approaches to tackle the low gene expression level and often extremely poor product yield. Finally, more efforts are needed in the studies of application as new biocontrol agents. A key factor for the success of this biological approach is the discovery of new microbial strains that can produce potent natural products with novel chemistry and modes of action.
Author Contributions
All authors listed have made a substantial, direct, and intellectual contribution to the work, and approved it for publication.
Funding
This work was supported by the Council of Scientific and Industrial Research (CSIR), New Delhi (Grant Nos. HCP-007 and MLP-4011) and SERB, New Delhi (Grant No. EMR/2016/002584).
Conflict of Interest
The authors declare that the research was conducted in the absence of any commercial or financial relationships that could be construed as a potential conflict of interest.
Publisher’s Note
All claims expressed in this article are solely those of the authors and do not necessarily represent those of their affiliated organizations, or those of the publisher, the editors and the reviewers. Any product that may be evaluated in this article, or claim that may be made by its manufacturer, is not guaranteed or endorsed by the publisher.
Acknowledgments
RP is thankful to Rusa/Fits/Purse grants and Dr. Seema Langer, HoD, Department of Zoology, University of Jammu, for constant support and encouragement. PG, Senior Scientist, is thankful to Department of Horticulture, RVSKVV, for encouragement and support for research work.
References
Abd-Elgawad, M. M. M. (2021). Photorhabdus spp.: an overview of the beneficial aspects of Mutualistic bacteria of insecticidal nematodes. Plants 10, 1660–1673. doi: 10.3390/plants10081660
Akhurst, R. J. (1982). Antibiotic activity of Xenorhabdus spp., bacteria symbiotically associated with insect pathogenic nematodes of the families Heterorhabditidae and Steinernematidae. J. Gen. Microbiol. 128, 3061–3065. doi: 10.1099/00221287-128-12-3061
Atanasov, A. G., Waltenberger, B., Pferschy-Wenzig, E. M., Linder, T., Wawrosch, C., Uhrin, P., et al. (2015). Discovery and resupply of pharmacologically active plant-derived natural products: a review. Biotechnol. Adv. 33, 1582–1614. doi: 10.1016/j.biotechadv.2015.08.001
Atanasov, A. G., Zotchev, S. B., Dirsch, V. M., and Supuran, C. T. (2021). Natural products in drug discovery: advances and opportunities. Nat. Rev. Drug Discov. 20, 200–216. doi: 10.1038/s41573-020-00114-z
Bai, Y. B., Zhang, A. L., Tang, J. J., and Gao, J. M. (2013). Synthesis and antifungal activity of 2-chloromethyl-1 H-benzimidazole derivatives against phytopathogenic fungi in vitro. J. Agric. Food Chem. 61, 2789–2795. doi: 10.1021/jf3053934
Baker, W., Van den, B. A., Camon, E., Hingamp, P., Sterk, P., Stoesser, G., et al. (2000). The EMBL nucleotide sequence database. Nucleic Acids Res. 28, 19–23. doi: 10.1093/nar/29.1.17
Baneth, G., and Solano, G. L. (2020). “Protozoal and protozoa like infections,” in Clinical Small Animal Internal Medicine, ed. D. S. Bruyette (Hoboken, NJ: Willey), 1003–1021. doi: 10.1002/9781119501237.ch110
Batista, R., De Jesus Silva Júnior, A., and De Oliveira, A. B. (2009). Plant-derived antimalarial agents: new leads and efficient phytomedicines. Part II. Non-alkaloidal natural products. Molecules 14, 3037–3072. doi: 10.3390/molecules14083037
Bock, C., Shapiro-Ilan, D., Wedge, D. E., and Cantrell, C. L. (2014). Identification of the antifungal compound, trans-cinnamic acid, produced by Photorhabdus luminescens, a potential biopesticide against pecan scab. J. Pest. Sci. 87, 1–9. doi: 10.1007/s10340-013-0519-5
Bode, H. B., Brachmann, A. O., Jadhav, K. B., Seyfarth, L., Dauth, C., Fuchs, S. W., et al. (2015). Structure elucidation and activity of kolossin a, the D-/L-Pentadecapeptide product of a giant nonribosomal peptide synthetase. Angew. Chem. Int. Ed. 54, 10352–10355. doi: 10.1002/anie.201502835
Bonadonna, G., Monfardini, S., De Lena, M., and Fossati-Bellani, F. (1969). Clinical evaluation of adriamycin, a new antitumour antibiotic. Br. Med. J. 3:5669. doi: 10.1136/bmj.3.5669.503
Bonnington, L. S., Tanaka, J., Higa, T., Kimura, J., Yoshimura, Y., Nakao, Y., et al. (1997). Cupolamide A: a cytotoxic cyclic heptapeptide from two samples of the sponge Theonella cupola. J. Org. Chem. 62, 7765–7767. doi: 10.1021/jo9710285
Brachmann, A. O., Joyce, S. A., Jenke-Kodama, H., Schwär, G., Clarke, D. J., and Bode, H. B. (2007). A type II polyketide synthase is responsible for anthraquinone biosynthesis in Photorhabdus luminescence. Chem. Biochem. 8, 1721–1728. doi: 10.1002/cbic.200700300
Braun, C., Engel, M., Seifert, M., Theisinger, B., Seitz, G., Zang, K. D., et al. (1999). Expression of calpain I messenger RNA in human renal cell carcinoma: correlation with lymph node metastasis and histological type. Int. J. Cancer 84, 6–9. doi: 10.1002/(sici)1097-0215(19990219)84:1<6::aid-ijc2>3.0.co;2-t
Bray, F., Ren, J. S., Masuyer, E., and Ferlay, J. (2013). Global estimates of cancer prevalence for 27 sites in the adult population in 2008. Int. J. Cancer 132, 1133–1145. doi: 10.1002/ijc.27711
Brusotti, G., Cesari, I., Dentamaro, A., Caccialanza, G., and Massolini, G. (2014). Isolation and characterization of bioactive compounds from plant resources: the role of analysis in the ethnopharmacological approach. J. Pharm. Biomed. Anal. 87, 218–228. doi: 10.1016/j.jpba.2013.03.007
Burke, J. E., and Dennis, E. A. (2009). Phospholipase A2 structure/function, mechanism, and signaling1. J. Lipid Res. 50, S237–S242. doi: 10.1194/jlr.R800033-JLR200
Bycroft, B. W., Maslen, C., Box, S. J., Brown, A., and Tyler, J. W. (1988). The biosynthetic implications of acetate and glutamate incorporation into (3r, 5r)-careapenam-3-carboxylic acid and (5r)-carbapen-2-em-3-carboxylic acid by serratia sp. J. Antibiot. 41, 1231–1242. doi: 10.7164/antibiotics.41.1231
Cabeza, L., Peña, M., Martínez, R., Mesas, C., Galisteo, M., Perazzoli, G., et al. (2021). Anemoniasulcata and its symbiont symbiodinium as a source of anti-tumor and anti-oxoxidant compounds for colon cancer therapy: a preliminary in vitro study. Biology 10:134. doi: 10.3390/biology10020134
Carmichael, W. W. (1992). Cyanobacteria secondary metabolites-the cyanotoxins. J. Appl. Bacteriol. 72, 445–459. doi: 10.1111/j.1365-2672.1992.tb01858.x
Castillo, J. C., Reynolds, S. E., and Eleftherianos, I. (2011). Insect immune responses to nematode parasites. Trends Parasitol. 27, 537–547. doi: 10.1016/j.pt.2011.09.001
Cha, J. W., Park, J. S., Sim, T., Nam, S. J., Kwon, H. C., Del Valle, J. R., et al. (2012). Structure assignment of lucentamycin E and revision of the olefin geometries of the marine-derived lucentamycins. J. Nat. Prod. 75, 1648–1651. doi: 10.1021/np3003854
Chalabaev, S., Turlin, E., Bay, S., Ganneau, C., Brito-Fravallo, E., Charles, J. F., et al. (2008). Cinnamic acid, an autoinducer of its own biosynthesis, is processed via Hca enzymes in Photorhabdus luminescens. Appl. Environ. Microbiol. 74, 1717–1725. doi: 10.1128/AEM.02589-07
Chamika, W. A. S., Ho, T. C., Roy, V. C., Kiddane, A. T., Park, J. S., Kim, G. D., et al. (2021). In vitro characterization of bioactive compounds extracted from sea urchin Stomopneustes variolaris using green and conventional techniques. Food Chem. 36, 129866–129872. doi: 10.1016/j.foodchem.2021.129866
Chen, Y. L., Huang, S. T., Sun, F. M., Chiang, Y. L., Chiang, C. J., Tsai, C. M., et al. (2011). Transformation of cinnamic acid from trans-to cis-form raises a notable bactericidal and synergistic activity against multiple-drug resistant Mycobacterium tuberculosis. Eur. J. Pharm. Sci. 43, 188–194. doi: 10.1016/j.ejps.2011.04.012
Cheng, S. S., Liu, J. Y., Huang, C. G., Hsui, Y. R., Chen, W. J., and Chang, S. T. (2009). Insecticidal activities of leaf essential oils from Cinnamomum osmophloeum against three mosquito species. Bioresour. Technol. 100, 457–464. doi: 10.1016/j.biortech.2008.02.030
Chitwood, D. J. (1999). Biochemistry and function of nematode steroids. Crit. Rev. Biochem. Mol. Biol. 34, 273–284. doi: 10.1080/10409239991209309
Ciche, T. A., and Ensign, J. C. (2003). For the insect pathogen Photorhabdus luminescens, which end of a nematode is out? Appl. Environ. Microbiol. 69, 1890–1897. doi: 10.1128/AEM.69.4.1890-1897.2003
Ciche, T. A., Bintrim, S. B., Horswill, A. R., and Ensign, J. C. (2001). A phosphopantetheinyltransferase homolog is essential for Photorhabdus luminescens to support growth and reproduction of the entomopathogenic nematode Heterorhabditis bacteriophora. J. Bacteriol. 183, 3117–3126. doi: 10.1128/JB.183.10.3117-3126.2001
Ciche, T. A., Blackburn, M., Carney, J. R., and Ensign, J. C. (2003). Photobactin: a catechol siderophore produced by Photorhabdus luminescens, an entomopathogen mutually associated with Heterorhabditis bacteriophora NC1 nematodes. Appl. Environ. Microbiol. 69, 4706–4713. doi: 10.1128/AEM.69.8.4706-4713.2003
Ciechanover, A. (1998). The ubiquitin–proteasome pathway: on protein death and cell life. EMBO. J. 17, 7151–7160. doi: 10.1093/emboj/17.24.7151
Ciehanover, A., Hod, Y., and Hershko, A. (1978). A heat-stable polypeptide component of an ATP-dependent proteolytic system from reticulocytes. Biochem. Biophys. Res. Commun. 81, 1100–1115. doi: 10.1016/0006-291X(78)91249-4
Cimen, H., Touray, M., Gulsen, S. H., Erincik, O., Wenski, S. L., Bode, H. B., et al. (2021). Antifungal activity of different Xenorhabdus and Photorhabdus species against various fungal phytopathogens and identification of the antifungal compounds from X. szentirmaii. App. Microbiol. Biotech. 105, 5517–5528. doi: 10.1007/s00253-021-11435-3
Coates, A. R., Halls, G., and Hu, Y. (2011). Novel classes of antibiotics or more of the same? Br. J. Pharmacol. 163, 184–194. doi: 10.1111/j.1476-5381.2011.01250.x
Coates, A., Hu, Y., Bax, R., and Page, C. (2002). The future challenges facing the development of new antimicrobial drugs. Nat. Rev. Drug Discov. 1, 895–910. doi: 10.1038/nrd940
Courtnay, R., Ngo, D. C., Malik, N., Ververis, K., Tortorella, S. M., and Karagiannis, T. C. (2015). Cancer metabolism and the Warburg effect: the role of HIF-1 and PI3K. Mol. Biol. Rep. 42, 841–851. doi: 10.1007/s11033-015-3858-x
Crawford, J. M., Portmann, C., Zhang, X., Roeffaers, M. B., and Clardy, J. (2012). Small molecule perimeter defense in entomopathogenic bacteria. Proc. Natl. Acad. Sci. U S A. 109, 10821–10826. doi: 10.1073/pnas.1201160109
Crawford, L. J., Walker, B., and Irvine, A. E. (2011). Proteasome inhibitors in cancer therapy. Cell Commun. Signal. 5, 101–110. doi: 10.1007/s12079-011-0121-7
Daborn, P. J., Waterfield, N., Blight, M. A., and Ffrench-Constant, R. H. (2001). Measuring virulence factor expression by the pathogenic bacterium Photorhabdus luminescens in culture and during insect infection. J. Bacteriol. 183, 5834–5839. doi: 10.1128/JB.183.20.5834-5839.2001
Derzelle, S., Duchaud, E., Kunst, F., Danchin, A., and Bertin, P. (2002). Identification, characterization, and regulation of a cluster of genes involved in carbapenem biosynthesis in Photorhabdus luminescens. Appl. Environ. Microbiol. 68, 3780–3789. doi: 10.1128/AEM.68.8.3780-3789.2002
Du, Q. S., Xie, N. Z., and Huang, R. B. (2015). Recent development of peptide drugs and advance on theory and methodology of peptide inhibitor design. Med. Chem. 11, 235–247. doi: 10.2174/1573406411666141229163355
Duchaud, E., Rusniok, C., Frangeul, L., Buchrieser, C., Givaudan, A., Taourit, S., et al. (2003). The genome sequence of the entomopathogenic bacterium Photorhabdus luminescens. Nat. Biotechnol. 21, 1307–1313. doi: 10.1038/nbt886
Dutky, S. R. (1974). “Nematode parasites,” in Proceedings of the Summer Institute on Biological Control of Plant Insects and Diseases, eds F. G. Maxwell and F. A. Harris (Jackson, MS: University Press of Mississippi), 576–590.
Ebada, S. S., Edrada, R. A., Lin, W., and Proksch, P. (2008). Methods for isolation, purification and structural elucidation of bioactive secondary metabolites from marine invertebrates. Nat. Protoc. 3, 1820–1831. doi: 10.1038/nprot.2008.182
Eleftherianos, I. G. (2009). Novel antibiotic compounds produced by the insect pathogenic bacterium Photorhabdus. Anti-Infect. Drug Discov. 4, 81–89. doi: 10.2174/157489109788490280
Eleftherianos, I., Boundy, S., Joyce, S. A., Aslam, S., Marshall, J. W., Cox, R. J., et al. (2007). An antibiotic produced by an insect-pathogenic bacterium suppresses host defenses through phenoloxidase inhibition. Proc. Natl. Acad. Sci. U S A. 104, 2419–2424. doi: 10.1073/pnas.0610525104
Ferlay, J., Shin, H. R., Bray, F., Forman, D., Mathers, C., and Parkin, D. M. (2010). Estimates of worldwide burden of cancer in 2008: globocan 2008. Int. J. Cancer 127, 2893–2917. doi: 10.1002/ijc.25516
Ferrer, J. L., Austin, M. B., Stewart, C. Jr., and Noel, J. P. (2008). Structure and function of enzymes involved in the biosynthesis of phenylpropanoids. Plant Physiol. Biochem. 46, 356–370. doi: 10.1016/j.plaphy.2007.12.009
Ffrench-Constant, R., Waterfield, N., Daborn, P., Joyce, S., Bennett, H., Au, C., et al. (2003). Photorhabdus: toward a functional genomic analysis of a symbiont and pathogen. FEMS Microbiol. Rev. 26, 433–456. doi: 10.1111/j.1574-6976.2003.tb00625.x
Fong, P. Y., Fesinmeyer, M. D., White, E., Farin, F. M., Srinouanprachanh, S., Afsharinejad, Z., et al. (2010). Association of diabetes susceptibility gene calpain-10 with pancreatic cancer among smokers. J. Gastrointest. Cancer 41, 203–208. doi: 10.1007/s12029-010-9130-7
Forst, S., and Nealson, K. (1996). Molecular biology of the symbiotic pathogenic bacteria Xenorhabdus spp. and Photorhabdus spp. Microbiol. Rev. 60, 21–43. doi: 10.1128/mr.60.1.21-43.1996
Forst, S., Dowds, B., Boemare, N., and Stackebrandt, E. (1997). Xenorhabdus and Photorhabdus spp.: bugs that kill bugs. Annu. Rev. Microbiol. 51, 47–72. doi: 10.1146/annurev.micro.51.1.47
Frances, C. P., Conde, M. C., Saez, M. E., Diez, S. F., Rey, C. M., Ramírez, A. J. A., et al. (2007). Identification of a protective haplogenotype within CAPN10 gene influencing colorectal cancer susceptibility. J. Gastroenterol. Hepatol. 22, 2298–2302. doi: 10.1111/j.1440-1746.2007.04843.x
Frink, R. E., Peyton, M., Schiller, J. H., Gazdar, A. F., Shay, J. W., and Minna, J. D. (2016). Telomerase inhibitor imetelstat has preclinical activity across the spectrum of non-small cell lung cancer oncogenotypes in a telomere length dependent manner. Oncotarget 7, 31639–31651. doi: 10.18632/oncotarget.9335
Fuchs, S. W., Bozhüyük, K. A., Kresovic, D., Grundmann, F., Dill, V., Brachmann, A. O., et al. (2013). Formation of 1, 3-cyclohexanediones and resorcinols catalyzed by a widely occuringketosynthase. Angew. Chem. Int. Ed. 52, 4108–4112. doi: 10.1002/anie.201210116
Garcia, H. H., Gonzalez, A. E., and Gilman, R. H. (2003). Diagnosis, treatment and control of Taeniasoliumcysticercosis. Curr. Opin. Infect. Dis. 16, 411–419. doi: 10.1097/00001432-200310000-200310007
Gerrard, J., Waterfield, N., and Vohra, R. (2004). Human infection with Photorhabdus asymbiotica: an emerging bacterial pathogen. Microb. Infect. 6, 229–237. doi: 10.1016/j.micinf.2003.10.018
Gessler, N. N., Egorova, A. S., and Belozerskaya, T. A. (2013). Fungal anthraquinones. Appl. Biochem. Microbiol. 49, 85–99. doi: 10.1134/S000368381302004X
Goll, D. E., Thompson, V. F., Li, H., Wei, W. E. I., and Cong, J. (2003). The calpain system. Physiol. Rev. 83, 731–801. doi: 10.1152/physrev.00029.2002
Groll, M., Schellenberg, B., Bachmann, A. S., Archer, C. R., Huber, R., Powell, T. K., et al. (2008). A plant pathogen virulence factor inhibits the eukaryotic proteasome by a novel mechanism. Nature 452, 755–758. doi: 10.1038/nature06782
Großelindemann, E., Lewis, M. J., Hedden, P., and Graebe, J. E. (1992). Gibberellin biosynthesis from gibberellin A 12-aldehyde in a cell-free system from germinating barley (Hordeumvulgare L., cv. Himalaya) embryos. Planta 188, 252–257. doi: 10.1007/BF00216821
Hakkim, F. L., Essa, M. M., Arivazhagan, G., Guizani, N., and Hyuk, S. (2012). Evaluation of food protective property of five natural products using fresh-cut apple slice model. Pak. J. Biol. Sci. 15, 10–18. doi: 10.3923/pjbs.2012.10.18
Hanahan, D., and Weinberg, R. A. (2011). Hallmarks of cancer: the next generation. Cell 144, 646–674. doi: 10.1016/j.cell.2011.02.013
Hapeshi, A., Healey, J. R. J., Mulley, G., and Waterfield, N. R. (2020). Temperature restriction in Entomopathogenic bacteria. Front. Microbiol. 11:548800. doi: 10.3389/fmicb.2020.548800
Harris, J. R. (1971). The proteins released from intact erythrocyte ‘ghosts’ at low ionic strength. Biochem. J. 122, 38–40. doi: 10.1042/bj1220038P
Hasan, M. A., Ahmed, S., Mollah, M. M. I., Lee, D., and Kim, Y. (2019). Variation in pathogenicity of different strains of Xenorhabdus nematophila; differential immunosuppressive activities and secondary metabolite production. J. Invertebr. Pathol. 166:107221. doi: 10.1016/j.jip.2019.107221
Hedden, P., and Kamiya, Y. (1997). Gibberellin biosynthesis: enzymes, genes and their regulation. Annu. Rev. Plant Biol. 48, 431–460. doi: 10.1146/annurev.arplant.48.1.431n
Hu, K., and Webster, J. M. (2000). Antibiotic production in relation to bacterial growth and nematode development in Photorhabdus–Heterorhabditis infected Galleria mellonella larvae. FEMS Microbiol. Lett. 189, 219–223. doi: 10.1111/j.1574-6968.2000.tb09234
Hu, K., Li, J., and Webster, J. M. (1997). Quantitative analysis of a bacteria-derived antibiotic in nematode-infected insects using HPLC–UV and TLC–UV methods. J. Chromatogr. B. Biomed. Appl. 703, 177–183. doi: 10.1016/S0378-4347(97)00398-8
Hu, K., Li, J., Li, B., Webster, J. M., and Chen, G. (2006). A novel antimicrobial epoxide isolated from larval Galleria mellonella infected by the nematode symbiont, Photorhabdus luminescens (Enterobacteriaceae). Bioorg. Med. Chem. 14, 4677–4681. doi: 10.1016/j.bmc.2006.01.025
Hu, K., Li, J., Wang, W., Wu, H., Lin, H., and Webster, J. M. (1998). Comparison of metabolites produced in vitro and in vivo by Photorhabdus luminescens, a bacterial symbiont of the entomopathogenic nematode Heterorhabditis megidis. Can. J. Microbiol. 44, 2–10. doi: 10.1139/w98-098
Jarosz, J. (1996). Ecology of anti-microbials produced by bacterial associates of Steinernema carpocapsae and Heterorhabditis bacteriophora. Parasitology 112, 545–552. doi: 10.1017/S0031182000066129
Jiang, W. G., Sanders, A. J., Katoh, M., Ungefroren, H., Gieseler, F., Prince, M., et al. (2015). Tissue invasion and metastasis: molecular, biological and clinical perspectives. Sem. Cancer Biol. 35, S244–S275. doi: 10.1016/j.semcancer.2015.03.008
Joyce, S. A., Brachmann, A. O., Glazer, I., Lango, L., Schwär, G., Clarke, D. J., et al. (2008). Bacterial biosynthesis of a multipotentstilbene. Angew. Chem. Int. Ed. 47, 1942–1945. doi: 10.1002/anie.200705148
Kamisawa, T., Wood, L. D., Itoi, T., and Takaori, K. (2016). Pancreatic cancer. Lancet 388, 2008–2020. doi: 10.1016/S0140-6736(16)00141-0
Kim, S. H., Jang, S. D., Lee, K. Y., Sung, S. H., and Kim, Y. C. (2009). Chemical constituents isolated from Polygala japonica leaves and their inhibitory effect on nitric oxide production in vitro. J. Enzyme. Inhib. Med. Chem. 24, 230–233. doi: 10.1080/14756360802051362
Kimura, Y., Koga, H., Araki, N., Mugita, N., Fujita, N., Takeshima, H., et al. (1998). The involvement of calpain-independent proteolysis of the tumor suppressor NF2 (merlin) in schwannomas and meningiomas. Nat. Med. 4, 915–922. doi: 10.1038/nm0898-915
Kisselev, A. F., Van der Linden, W. A., and Overkleeft, H. S. (2012). Proteasome inhibitors: an expanding army attacking a unique target. Chem. Biol. 19, 99–115. doi: 10.1016/j.chembiol.2012.01.003
Korkina, L. G. (2007). Phenylpropanoids as naturally occurring antioxidants: from plant defense to human health. Cell. Mol. Biol. 53, 15–25.
Kronenwerth, M., Brachmann, A. O., Kaiser, M., and Bode, H. B. (2014). Bioactive derivatives of isopropylstilbene from mutasynthesis and chemical synthesis. Chem. Bio. Chem. 15, 2689–2691. doi: 10.1002/cbic.201402447
Kyle, R. A., and Rajkumar, S. V. (2009). Treatment of multiple myeloma: a comprehensive review. Clin. Lymphoma Myeloma Leuk. 9, 278–288. doi: 10.3816/CLM.2009.n.056
Lachance, H., Wetzel, S., Kumar, K., and Waldmann, H. (2012). Charting, navigating, and populating natural product chemical space for drug discovery. J. Med. Chem. 55, 5989–6001. doi: 10.1021/jm300288g
Lakshmikuttyamma, A., Selvakumar, P., Kanthan, R., Kanthan, S. C., and Sharma, R. K. (2004). Overexpression of m-calpain in human colorectal adenocarcinomas. Cancer Epidemiol. Biomark. Prev. 13, 1604–1609.
Lee, S. J., Choi, Y. L., Lee, E. J., Kim, B. G., Bae, D. S., Ahn, G. H., et al. (2007). Increased expression of calpain 6 in uterine sarcomas and carcinosarcomas: an immunohistochemical analysis. Int. J. Gynecol. Cancer 17, 248–253. doi: 10.1111/j.1525-1438.2006.00756.x
Lee, S. J., Kim, B. G., Choi, Y. L., and Lee, J. W. (2008). Increased expression of calpain 6 during the progression of uterine cervical neoplasia: immunohistochemical analysis. Oncol. Rep. 19, 859–863. doi: 10.3892/or.19.4.859
Lee, S. M., Kim, M. S., Hayat, F., and Shin, D. (2019). Recent advances in the discovery of novel antiprotozoal agents. Molecules 24, 3886–3893. doi: 10.3390/molecules24213886
Li, J., Chen, G., Wu, H., and Webster, J. M. (1995). Identic/cation of two pigments and a hydroxystilbene antibiotic from Photorhabdus luminescens. Appl. Environ. Microbiol. 61, 4329–4333. doi: 10.1128/aem.61.12.4329-4333.1995
Lim, W., Yang, C., Bazer, F. W., and Song, G. (2017). Chrysophanol induces apoptosis of choriocarcinoma through regulation of ROS and the AKT and ERK1/2 pathways. J. Cell. Physiol. 232, 31–39. doi: 10.1002/jcp.25423
Lulamba, T. E., Green, E., and Serepa-Dlamini, M. H. (2021). Photorhabdus sp. ETL antimicrobial properties and characterization of its secondary metabolites by gas chromatography–mass spectrometry. Life 11, 787–792. doi: 10.3390/life11080787
Machado, R. A. R., Wüthrich, D., Kuhnert, P., Arce, C. C. M., Thönen, L., Ruiz, C., et al. (2018). Whole-genome-based revisit of Photorhabdus phylogeny: proposal for the elevation of most Photorhabdus subspecies to the species level and description of one novel species Photorhabdus bodei sp. nov., and one novel subspecies Photorhabdus laumondii subsp. clarkei subsp. Nov. Int. J. Syst. Evol. Microbiol. 68, 2664–2681. doi: 10.1099/ijsem.0.002820
Mamoune, A., Luo, J. H., Lauffenburger, D. A., and Wells, A. (2003). Calpain-2 as a target for limiting prostate cancer invasion. Cancer Res. 63, 4632–4640.
Mar, A. C., Chu, C. H., Lee, H. J., Chien, C. W., Cheng, J. J., Yang, S. H., et al. (2015). Interleukin-1 receptor type 2 acts with c-Fos to enhance the expression of interleukin-6 and vascular endothelial growth factor A in colon cancer cells and induce angiogenesis. J. Biol. Chem. 290, 22212–22224. doi: 10.1074/jbc.M115.644823
Marokhazi, J., Waterfield, N., LeGoff, G., Feil, E., Stabler, R., Hinds, J., et al. (2003). Using a DNA microarray to investigate the distribution of insect virulence factors in strains of Photorhabdus bacteria. J. Bacteriol. 185, 4648–4656. doi: 10.1128/JB.185.15.4648-4656.2003
Mayefis, D., and Widiastuti, S. (2021). Antioxidant activity of methanol extracts natuna marine sponge (porifera) with dpphmethode. Asian J. Pharm. Clin. Res. 80, 109–112. doi: 10.22159/ajpcr.2021.v14i4.40091
Méndez, C., and Salas, J. A. (2001). Altering the glycosylation pattern of bioactive compounds. Trends Biotechnol. 19, 449–456. doi: 10.1016/S0167-7799(01)01765-6
Merdad, A., Karim, S., Schulten, H. J., Dallol, A., Buhmeida, A., Al-Thubaity, F., et al. (2014). Expression of matrix metalloproteinases (MMPs) in primary human breast cancer: MMP-9 as a potential biomarker for cancer invasion and metastasis. Anticancer. Res. 34, 1355–1366.
Monks, T. J., Hanzlik, R. P., Cohen, G. M., Ross, D., and Graham, D. G. (1992). Quinone chemistry and toxicity. Toxicol. Appl. Pharmacol. 112, 2–16. doi: 10.1016/0041-008X(92)90273-U
Moreno, L. R., Abrante, A., Esteban, F., González, M. M. A., Delgado, R. M., Sáez, M. E., et al. (2011). Calpain 10 gene and laryngeal cancer: a survival analysis. Head Neck 33, 72–76. doi: 10.1002/hed.21404
Moretti, D., Del Bello, B., Cosci, E., Biagioli, M., Miracco, C., and Maellaro, E. (2009). Novel variants of muscle calpain 3 identified in human melanoma cells: cisplatin-induced changes in vitro and differential expression in melanocytic lesions. Carcinogenesis 30, 960–967. doi: 10.1093/carcin/bgp098
Nollmann, F. I., Heinrich, A. K., Brachmann, A. O., Morisseau, C., Mukherjee, K., and Casanova Torres, et al. (2015b). A photorhabdus natural product inhibits insect juvenile hormone epoxide hydrolase. Chem. Bio. Chem. 16, 766–771. doi: 10.1002/cbic.201402650
Nollmann, F. I., Dauth, C., Mulley, G., Kegler, C., Kaiser, M., Waterfield, N. R., et al. (2015a). Insect-specific production of new GameXPeptides in Photorhabdus luminescens TTO1, widespread natural products in entomopathogenic bacteria. Chem. Bio. Chem. 16, 205–208. doi: 10.1002/cbic.201402603
Ntie-Kang, F., and Svozil, D. (2020). An enumeration of natural products from microbial, marine and terrestrial sources. Phys. Sci. Rev. 5, 2021–2026. doi: 10.1515/psr-2018-0121
Oka, M., Yaginuma, K., Numata, K., Konishi, M., Oki, T., and Kawaguchi, H. (1988). Glidobactins a, b and c, new antitumor antibiotics. J. Antibiot. 41, 1331–1337. doi: 10.7164/antibiotics.41.1338
Pankewitz, F., Zöllmer, A., Gräser, Y., and Hilker, M. (2007). Anthraquinones as defensive compounds in eggs of Galerucini leaf beetles: biosynthesis by the beetles? Arch. Insect Biochem. Physiol. 66, 98–108. doi: 10.1002/arch.20215
Park, H. B., and Crawford, J. M. (2016). Pyrazinone protease inhibitor metabolites from Photorhabdus luminescens. J. Antibiot. 69:8. doi: 10.1038/ja.2016.79
Paul, V. J., Frautschy, S., Fenical, W., and Nealson, K. H. (1981). Antibiotics in microbial ecology: isolation and structure assignment of several new antibacterial compounds for the insect symbiotic bacteria Xenorhabdusspp. J. Chem. Ecol. 7, 589–597. doi: 10.1007/BF00987707
Peel, M. M., Alfredson, D. A., Gerrard, J. G., Davis, J. M., Robson, J. M., McDougall, R. J., et al. (1999). Isolation, identification, and molecular characterization of strains of Photorhabdus luminescens from infected humans in Australia. J. Clin. Microbiol. 37, 3647–3653. doi: 10.1128/JCM.37.11.3647-3653.1999
Pérez, M. A. (2014). Cancer Stem Cells, Reason of the Failure of Conventional Cancer Treatments. Barcelona: Autonomous University of Barcelona, Department of Cell Biology, Physiology and Immunology.
Plowe, C. V. (2005). Antimalarial drug resistance in Africa: strategies for monitoring and deterrence. Curr. Top. Microbiol. Immunol. 295, 55–79. doi: 10.1007/3-540-29088-5_3
Powers, J. H. (2004). Antimicrobial drug development–the past, the present, and the future. Clin. Microbiol. Infect. 10, 23–31. doi: 10.1111/j.1465-0691.2004.1007.x
Pozio, E. (2007). World distribution of Trichinella spp. infections in animals and humans. Vet. Parasitol. 149, 3–21. doi: 10.1016/j.vetpar.2007.07.002
Ramesh, C., Tulasi, B. R., Raju, M., Thakur, N., and Dufossé, L. (2021). Marine natural products from tunicates and their associated microbes. Mar. Drugs 19:308. doi: 10.3390/md19060308
Reichrath, J., Welter, C., Mitschele, T., Classen, U., Meineke, V., Tilgen, W., et al. (2003). Different expression patterns of calpainisozymes 1 and 2 (CAPN1 and 2) in squamous cell carcinomas (SCC) and basal cell carcinomas (BCC) of human skin. J. Pathol. 199, 509–516. doi: 10.1002/path.1308
Renslo, A. R., and McKerrow, J. H. (2006). Drug discovery and development for neglected parasitic diseases. Nat. Chem. Biol. 2, 701–710. doi: 10.1038/nchembio837m
Riccio, G., Nuzzo, G., Zazo, G., Coppola, D., Senese, G., Romano, L., et al. (2021). Bioactivity screening of antarctic sponges reveals anticancer activity and potential cell death via ferroptosis by mycalols. Mar. Drugs 19:459. doi: 10.3390/md19080459
Richardson, W. H., Schmidt, T. M., and Nealson, K. H. (1988). Identification of an anthraquinone pigment and a hydroxystilbene antibiotic from Xenorhabdus luminescens. Appl. Environ. Microbiol. 54, 1602–1605. doi: 10.1128/aem.54.6.1602-1605.1988
Rios-Doria, J., Day, K. C., Kuefer, R., Rashid, M. G., Chinnaiyan, A. M., Rubin, M. A., et al. (2003). The role of calpain in the proteolytic cleavage of E-cadherin in prostate and mammary epithelial cells. J. Biol. Chem. 278, 1372–1379. doi: 10.1074/jbc.M208772200
Saito, K., Konno, A., Ishii, H., Saito, H., Nishida, F., Abe, T., et al. (2001). Nodularin-Har: a new nodularin from Nodularia. J. Nat. Prod. 64, 139–141. doi: 10.1021/np000299z
Salehin, D., Fromberg, I., Haugk, C., Dohmen, B., Georg, T., Bohle, R. M., et al. (2010). Immunhistochemical analysis for expression of calpain 1, calpain 2 and calpastatin in endometrial cancer. Anticancer. Res. 30, 2837–2843.
Saremi, H., and Okhovvat, S. M. (2006). Mycotoxin producing Fusarium species associated with plant disease on potato, wheat, corn and animal diseases in northwest Iran. Commun. Agric. Appl. Biol. Sci. 71, 1175–1185.
Sato, K., and Kawashima, S. (2001). Calpain function in the modulation of signal transduction molecules. Biol. Chem. 382, 743–751. doi: 10.1515/bchm.2001.382.5.743
Savary, S., Teng, P. S., Willocquet, L., and Nutter, F. W. Jr. (2006). Quantification and modeling of crop losses: a review of purposes. Annu. Rev. Phytopathol. 44, 89–112. doi: 10.1146/annurev.phyto.44.070505.143342
Shah, J. J., and Orlowski, R. Z. (2009). Proteasome inhibitors in the treatment of multiple myeloma. Leukemia 23, 1964–1979. doi: 10.1038/leu.2009.173
Sharma, S., Waterfield, N., Bowen, D., Rocheleau, T., Holland, L., James, R., et al. (2002). The lumicins: novel bacteriocins from Photorhabdus luminescens with similarity to the uropathogenic-specific protein (USP) from uropathogenic Escherichia coli. FEMS Microbiol. Lett. 214, 241–249. doi: 10.1111/j.1574-6968.2002.tb11354.x
Shen, B. (2015). A new golden age of natural products drug discovery. Cell 163, 1297–1300. doi: 10.1016/j.cell.2015.11.031
Shi, D., An, R., Zhang, W., Zhang, G., and Yu, Z. (2017). Stilbene derivatives from Photorhabdus temperata SN259 and their antifungal activities against phytopathogenic fungi. J. Agric. Food Chem. 65, 60–65. doi: 10.1021/acs.jafc.6b04303
Shoji, J. I., Hinoo, H., Kato, T., Hattori, T., Hirooka, K., Tawara, K., et al. (1990). Isolation of cepafungins I, II and III from Pseudomonas species. J. Antibiot. 43, 783–787. doi: 10.7164/antibiotics.43.783
Shrestha, S., and Kim, Y. (2008). Eicosanoids mediate prophenoloxidase release from oenocytoids in the beet armyworm Spodoptera exigua. Insect. Biochem. Mol. Biol. 38, 99–112. doi: 10.1016/j.ibmb.2007.09.013
Shrestha, S., Stanley, D., and Kim, Y. (2011). PGE2 induces oenocytoid cell lysis via a G protein-coupled receptor in the beet armyworm, Spodoptera exigua. J. Insect Physiol. 57, 1568–1576. doi: 10.1016/j.jinsphys.2011.08.010
Si, W., Gong, J., Tsao, R., Zhou, T., Yu, H., Poppe, C., et al. (2006). Antimicrobial activity of essential oils and structurally related synthetic food additives towards selected pathogenic and beneficial gut bacteria. J. Appl. Microbiol. 100, 296–3505. doi: 10.1111/j.1365-2672.2005.02789.x
Sibiya, A., Jeyavani, J., Sivakamavalli, J., Ravi, C., Divya, M., and Vaseeharan, B. (2021). Bioactive compounds from various types of sea urchin and their therapeutic effects—a review. Reg. Stud. Mar. Sci. 44, 101760–101768. doi: 10.1016/j.rsma.2021.101760
Silva, C. P., Waterfield, N. R., Daborn, P. J., Dean, P., Chilver, T., Au, C. P., et al. (2002). Bacterial infection of a model insect: Photorhabdus luminescens and Manducasexta. Cell. Microbiol. 4, 329–339. doi: 10.1046/j.1462-5822.2002.00194.x
Singh, R. K., Ranjan, A., Singh, M., and Srivastava, A. K. (2021). Anticancer natural product from marine invertebrates. Evol. Diversity Source Anticancer Mol. 2, 255–266. doi: 10.1016/B978-0-12-821710-8.00011-4
Sivaramakrishnan, S., and Razia, M. (2021). “Recent advances and future prospect,” in Entomopathogenic Nematodes and Their Symbiotic Bacteria, eds S. Sivaramakrishnan and Razia, M. (New York, NY: Springer), 159–161. doi: 10.1007/978-1-0716-1445-7_12
Snellenberg, S., Cillessen, S. A., Van Criekinge, W., Bosch, L., Meijer, C. J., Snijders, P. J., et al. (2014). Methylation-mediated repression of PRDM14 contributes to apoptosis evasion in HPV-positive cancers. Carcinogenesis 35, 2611–2618. doi: 10.1093/carcin/bgu197
Solomon, H. K., and William, W. W. (2003). In Bioactive Food Components, 2 Edn. New York, NY: Charles Scribner’s Sons.
Stanley, D. W. (2014). Eicosanoids in Invertebrate Signal Transduction Systems. Princeton NJ: Princeton University Press, doi: 10.1515/9781400865055
Stanley, D., and Kim, Y. (2019). Prostaglandins and other eicosanoids in insects: biosynthesis and biological actions. Front. Physiol. 9:1927. doi: 10.3389/fphys.2018.01927
Stein, M. L., Beck, P., Kaiser, M., Dudler, R., Becker, C. F., and Groll, M. (2012). One-shot NMR analysis of microbial secretions identifies highly potent proteasome inhibitor. Proc. Natl. Acad. Sci. U S A. 109, 18367–18371. doi: 10.1073/pnas.1211423109
Steketee, R. W., Wirima, J. J., Slutsker, L., Breman, J. G., and Heymann, D. L. (1996). Comparability of treatment groups and risk factors for parasitemia at the first antenatal clinic visit in a study of malaria treatment and prevention in pregnancy in rural Malawi. Am. J. Trop. Med. Hyg. 55, 17–23. doi: 10.4269/ajtmh.1996.55.17
Stock, S. P., Kusakabe, A., and Orozco, R. A. (2017). Secondary metabolites produced by Heterorhabditis symbionts and their application in agriculture: what we know and what to do next. J. Nematol. 49, 373–383.
Sztaricskai, F., Dinya, Z., Batta, G. Y., Szallas, E., Szentirmai, A., and Fodor, A. (1992). Anthraquinones produced by enterobacters and nematodes. Acta Chim. Hung. 129, 697–707.
Tarver, T. (2012). Cancer facts & figures 2012. American Cancer Society (ACS). American Cancer Soc. 2012:66. doi: 10.1080/15398285.2012.701177
Theodore, C. M., King, J. B., You, J., and Cichewicz, R. H. (2012). Production of cytotoxic glidobactins/luminmycins by Photorhabdus asymbiotica in liquid media and live crickets. J. Nat. Prod. 75, 2007–2011. doi: 10.1021/np300623x
Tsvetkov, V., Todorov, D., Hinkov, A., Velkova, L., Dolashki, A., et al. (2021). Effect of extracts of some species from Phylum Mollusca against the replication of Human Alphaherpesviruses types. Bulg. Chem. Commun. 53, 66–72.
Uhlemann, A. C., and Krishna, S. (2005). Antimalarial multi-drug resistance in Asia: mechanisms and assessment. Curr. Top. Microbiol. Immunol. 295, 39–53. doi: 10.1007/3-540-29088-5_2
Ullah, I., Khan, A. L., Ali, L., Khan, A. R., Waqas, M., Hussain, J., et al. (2015). Benzaldehyde as an insecticidal, antimicrobial, and antioxidant compound produced by Photorhabdus temperata M1021. J. Microbiol. 53, 127–133. doi: 10.1007/s12275-015-4632-4
Ullah, I., Khan, A. L., Ali, L., Khan, A. R., Waqas, M., Lee, I. J., et al. (2014a). An insecticidal compound produced by an insect-pathogenic bacterium suppresses host defenses through phenoloxidase inhibition. Molecules 19, 20913–20928. doi: 10.3390/molecules191220913
Ullah, I., Khan, A. R., Jung, B. K., Khan, A. L., Lee, I. J., and Shin, J. H. (2014b). Gibberellins synthesized by the entomopathogenic bacterium, Photorhabdus temperata M1021 as one of the factors of rice plant growth promotion. J. Plant Interact. 9, 775–782. doi: 10.1080/17429145.2014.942956
Van Gorkom, B. A. P., Timmer-Bosscha, H., De Jong, S., Van der Kolk, D. M., Kleibeuker, J. H., and De Vries, E. G. E. (2002). Cytotoxicity of rhein, the active metabolite of sennoside laxatives, is reduced by multidrug resistance-associated protein 1. Br. J. Cancer 86, 1494–1500. doi: 10.1038/sj.bjc.6600255
Voorhees, P. M., Dees, E. C., O’Neil, B., and Orlowski, R. Z. (2003). The proteasome as a target for cancer therapy. Clin. Cancer Res. 9, 6316–6325.
Wang, C., Zhang, D., Ma, H., and Liu, J. (2007). Neuroprotective effects of emodin-8-O-β-d-glucoside in vivo and in vitro. Eur. J. Pharmacol. 577, 58–63. doi: 10.1016/j.ejphar.2007.08.033
Waterfield, N. R., Ciche, T., and Clarke, D. (2009). Photorhabdus and a host of hosts. Annual Rev. Microbiol. 63, 557–574. doi: 10.1146/annurev.micro.091208.073507
Weinke, T., Trautmann, M., Held, T., Weber, G., Eichenlaub, D., Fleischer, K., et al. (1991). Neuropsychiatric side effects after the use of mefloquine. Am. J. Trop. Med. Hyg. 45, 86–91. doi: 10.4269/ajtmh.1991.45.86
Wewengkang, D. S., Rotinsulu, H., Sumilat, D. A., Oda, T., Yamazaki, H., Ukai, K., et al. (2021). Three bioactive compounds against colony formation of chinese hamster V79 cells from an indonesian ascidian Didemnum sp. Chem. Nat. Compd. 57, 592–593. doi: 10.1007/s10600-021-03427-6
Wilkinson, P., Waterfield, N. R., Crossman, L., Corton, C., Sanchez-Contreras, M., Vlisidou, I., et al. (2009). Comparative genomics of the emerging human pathogen Photorhabdus asymbiotica with the insect pathogen Photorhabdus luminescens. BMC Genomics 10:309. doi: 10.1186/1471-2164-10-302
Williams, J. S., Thomas, M., and Clarke, D. J. (2005). The gene stlA encodes a phenylalanine ammonia-lyase that is involved in the production of a stilbene antibiotic in Photorhabdus luminescens TT01. Microbiology 151, 2543–2550. doi: 10.1099/mic.0.28136-0
Williamson, J. M., Inamine, E., Wilson, K. E., Douglas, A. W., Liesch, J. M., and Albers-Schönberg, G. (1985). Biosynthesis of the beta-lactam antibiotic, thienamycin, by Streptomyces cattleya. J. Biol. Chem. 260, 4637–4647. doi: 10.1016/S0021-9258(18)89118-9
Wong, S. Y., Grant, I. R., Friedman, M., Elliott, C. T., and Situ, C. (2008). Antibacterial activities of naturally occurring compounds against Mycobacterium avium sub sp. paratuberculosis. Appl. Environ. Microbiol. 74, 5986–5990. doi: 10.1128/AEM.00981-08
Wu, Y., Jin, F., Wang, Y., Li, F., Ren, Z., and Wang, Y. (2017). In vitro and in vivo inhibitory effects of 6-hydroxyrubiadin on lipopolysaccharide-induced inflammation. Immunopharmacol. Immunotoxicol. 39, 107–116. doi: 10.1080/08923973.2017.1295053
Yang, E. J., Kim, S. H., Lee, K. Y., and Song, K. S. (2018). Neuroprotective and anti-neuroinflammatory activities of anthraquinones isolated from Photorhabdus temperata culture broth. J. Microbiol. Biotechnol. 28, 12–21. doi: 10.4014/jmb.1708.08067
Yin, J., Zhu, H., Xia, L., Ding, X., Hoffmann, T., Hoffmann, M., et al. (2015). A new recombineering system for Photorhabdus and Xenorhabdus. Nucleic Acids Res. 43:e36.
Yoshikawa, Y., Mukai, H., Hino, F., Asada, K., and Kato, I. (2000). Isolation of two novel genes, down regulated in gastric cancer. Jpn. J. Cancer Res. 91, 449–463. doi: 10.1111/j.1349-7006.2000.tb00967.x
Zhang, F., Ren, J., and Zhan, J. (2021). Identification and characterization of an efficient phenylalanine ammonia-lyase from Photorhabdus luminescens. Appl. Biochem. Biotech. 193, 1099–1115. doi: 10.1007/s12010-020-03477-6
Zhao, L., and Bode, H. B. (2019). Production of a photohexapeptide library from entomopathogenic Photorhabdus asymbiotica PB68. Org. Biomol. Chem. 17, 7858–7862. doi: 10.1039/C9OB01489F
Zhao, L., Awori, R. M., Kaiser, M., Groß, J., Opatz, T., and Bode, H. B. (2019). Structure, biosynthesis, and bioactivity of photoditritide from Photorhabdus temperata Meg1. J. Nat. Prod. 82, 3499–3503. doi: 10.1021/acs.jnatprod.9b00932
Zhao, L., Le Chapelain, C., Brachmann, A. O., Kaiser, M., Groll, M., and Bode, H. B. (2021). Activation, structure, biosynthesis and bioactivity of glidobactin-like proteasome inhibitors from Photorhabdus laumondii. Chem. Bio. Chem. 22, 1582–1588. doi: 10.1002/cbic.202100014
Keywords: Heterorhabditis, Photorhabdus, nematodes, antimicrobial, antiprotozoal, anti-inflammatory and anticancer
Citation: Parihar RD, Dhiman U, Bhushan A, Gupta PK and Gupta P (2022) Heterorhabditis and Photorhabdus Symbiosis: A Natural Mine of Bioactive Compounds. Front. Microbiol. 13:790339. doi: 10.3389/fmicb.2022.790339
Received: 06 October 2021; Accepted: 02 February 2022;
Published: 29 March 2022.
Edited by:
Louis S. Tisa, University of New Hampshire, United StatesReviewed by:
Piyush Baindara, University of Missouri, United StatesSumit Vashisth, Dr. Yashwant Singh Parmar University of Horticulture and Forestry, India
Javad Karimi, Ferdowsi University of Mashhad, Iran
Davoud Mohammadi, Azarbaijan Shahid Madani University, Iran
Vincent Gray, University of the Witwatersrand, South Africa
Rosalba Salgado-Morales, Universidad Autónoma del Estado de Morelos, Mexico
Copyright © 2022 Parihar, Dhiman, Bhushan, Gupta and Gupta. This is an open-access article distributed under the terms of the Creative Commons Attribution License (CC BY). The use, distribution or reproduction in other forums is permitted, provided the original author(s) and the copyright owner(s) are credited and that the original publication in this journal is cited, in accordance with accepted academic practice. No use, distribution or reproduction is permitted which does not comply with these terms.
*Correspondence: Prasoon Gupta, guptap@iiim.ac.in
†These authors have contributed equally to this work