- 1Department of Neuroradiology, Mayo Clinic, Rochester, MN, United States
- 2Nuffield Department of Primary Care Health Sciences and Department for Continuing Education (EBHC Program), Oxford University, Oxford, United Kingdom
- 3Faculty of Medicine, Al-Azhar University, Cairo, Egypt
- 4School of Medicine, American University of the Caribbean, Philipsburg, Sint Maarten
- 5Qena Faculty of Medicine, South Valley University, Qena, Egypt
- 6Medical Research Center, Kateb University, Kabul, Afghanistan
- 7Barry University, Miami, FL, United States
- 8Faculty of Physical Therapy, Sinai University, Cairo, Egypt
- 9Faculty of Physical Therapy, Cairo University, Giza, Egypt
- 10Department of Internal Medicine, NYC Health + Hospitals/Metropolitan, New York, NY, United States
- 11Faculty of Medicine, October 6 University, Giza, Egypt
- 12Department of Neurosurgery, Mayo Clinic Rochester, Rochester, MN, United States
Stroke is the second most common cause of global death following coronary artery disease. Time is crucial in managing stroke to reduce the rapidly progressing insult of the ischemic penumbra and the serious neurologic deficits that might follow it. Strokes are mainly either hemorrhagic or ischemic, with ischemic being the most common of all types of strokes. Thrombolytic therapy with recombinant tissue plasminogen activator and endovascular thrombectomy are the main types of management of acute ischemic stroke (AIS). In addition, there is a vital need for neuroprotection in the setting of AIS. Neuroprotective agents are important to investigate as they may reduce mortality, lessen disability, and improve quality of life after AIS. In our review, we will discuss the main types of management and the different modalities of neuroprotection, their mechanisms of action, and evidence of their effectiveness after ischemic stroke.
Introduction
The rapid development of neurologically related deficits is a potent indicator of acute ischemic stroke (AIS) (1). This happens as a result of the presence of an acute vascular etiology that lasts for at least 24 h and focally affects the central nervous system, inducing major disturbances to the related functions of the affected areas that may even end with mortality (1, 2). In general, stroke is the second most common cause of global death following coronary artery disease, and estimates show an annual incidence of 12.2 million (3). In addition, estimates show that it affects nearly 795,000 patients in the US (4). Reports also showed that stroke is the third most common contributor to disability and increased morbidities in approximately half of the stroke survivors over 65 years of age (4, 5).
Time is crucial in managing stroke to reduce the rapidly progressing insult of the ischemic penumbra and the severe neurologic deficits that might follow (6, 7). Hence, early management provides a window of opportunity for protecting against the development of severe complications and death, subsequently enhancing the prognosis (8). Furthermore, evidence concerning the management of stroke is changing quickly. Many studies are being published that report novel technical modalities and discoveries that can help improve the outcomes of stroke (9, 10). AIS has many management routes, including intravenous (IV) thrombolytic drugs such as recombinant tissue plasminogen activator (rtPA), control of body temperature and blood sugar, and blood pressure reduction of intracranial tension and neuroprotective agents (11). While IV thrombolytic agents help restore blood flow through an occluded vessel, neuroprotective agents are very important for maintaining the function of neurons surrounding dead brain tissue and, hence, limit after-stroke deficits (12).
There is rising interest in knowing more about neuroprotective agents, as shown in the currently ongoing studies listed in Table 1. These studies aim to investigate neuroprotective agents that can be beneficial to intervene against ischemic stroke and are the intended primary outcomes of these studies. In addition, data about neuroprotection approaches can now be easily accessed through multiple national and international registries that can help researchers globally. However, there is still no consensus about the unified management modalities to achieve the best prognosis. Currently, the US Food and Drug Administration (FDA) has not approved a specific treatment modality as neuroprotective therapy for ischemic stroke. This study aims to elaborate on the current gold standard management modalities of AIS. We will also discuss the latest reported evidence about the neuroprotective modalities as per evidence from studies in the literature.
Methods
The current literature review was conducted based on a comprehensive search strategy through various electronic databases, including EMBASE, Medline, and Cochrane library. No restrictions regarding the year of publication or country of the population were applied. However, we only included studies that were published in English. The search strategy was built on different relevant keywords obtained from relevant investigations and using the medical subject headings (MeSH). The formulated search strategy was modified based on the terms and conditions of each database to obtain the most relevant number of investigations. Finally, we conducted an additional manual search within the references of relevant articles and reviews to obtain any missed articles during the search strategy. We intended to include studies that reported neuroprotective agents that can be used for AIS patients.
Consequences of Blood Supply Disruption
After an ischemic stroke has occurred, various pathologic mechanisms occur, ultimately leading to brain tissue damage. Knowing how ischemia affects the brain is critical in assessing how to treat patients suffering from ischemic stroke. Once disruption of the blood supply has occurred, the cells of the brain die by several mechanisms. First, the diminished blood flow of the infarcted tissue leads to necrosis in adjacent penumbra tissue and decreased cellular metabolism (13). Second, when energy levels are depleted through the decreased generation of adenosine 5′-triphosphate, leading to sodium/potassium pump failure, the depolarization of the cell allows for calcium influx, resulting in cell apoptosis via the intrinsic pathway (14).
Evidence indicates that different factors that play a role in the level of ischemic brain tissue damage are neuronal excitotoxicity, peri-infarct depolarizations, local inflammation, and the accumulation of oxidative stress (15, 16). In this context, oxygen deprivation and blood flow loss lead to a near-immediate halt on neuroelectric activity and a loss of ion homeostasis (17–19). This ultimately lowers the energy stores by lowering adenosine triphosphate production (via mitochondrial dysfunction), leading to failure in the sodium-potassium pump. The cell's ion gradient is then compromised, leading to increased release of glutamate and aspartate at toxic concentrations (17–19). The increased glutamate release causes the activation of ionotropic receptors, leading to calcium influx and overload. This eventually leads to cellular damage, neuronal over-excitement, and ultimately cell death (20, 21).
Besides, free radical oxygen species (ROS) and calcium play a role in increasing levels of inflammatory cytokines, chemokines, and endothelial cell adhesion molecules, which assist in inflammatory cell migration and the upregulation of specific proinflammatory genes (15, 22, 23). When the sodium/potassium pump is impaired, as are glutamate and glucose transporters when free radicals are formed, mechanisms behind this are believed to be due to 4-hydroxynonenal production, lipid peroxidation, and their role in inducing apoptosis (24).
Another factor linked to brain cell death and a decreased patient prognosis following an ischemic stroke is increased cerebral edema (25). With the brain being in such a confined space, postinflammatory edema can lead to rapid brain tissue damage. In addition, various antiport channels have been shown to lead to cerebral edema after ischemic stroke. These channels include the sulfonylurea receptor 1–transient receptor potential melastatin 4 (SUR1-TRPM4) cation channel and the aquaporin-4 channel (26). Thus, the limitations of post-ischemic stroke edema could play a neuroprotective role (Figure 1).
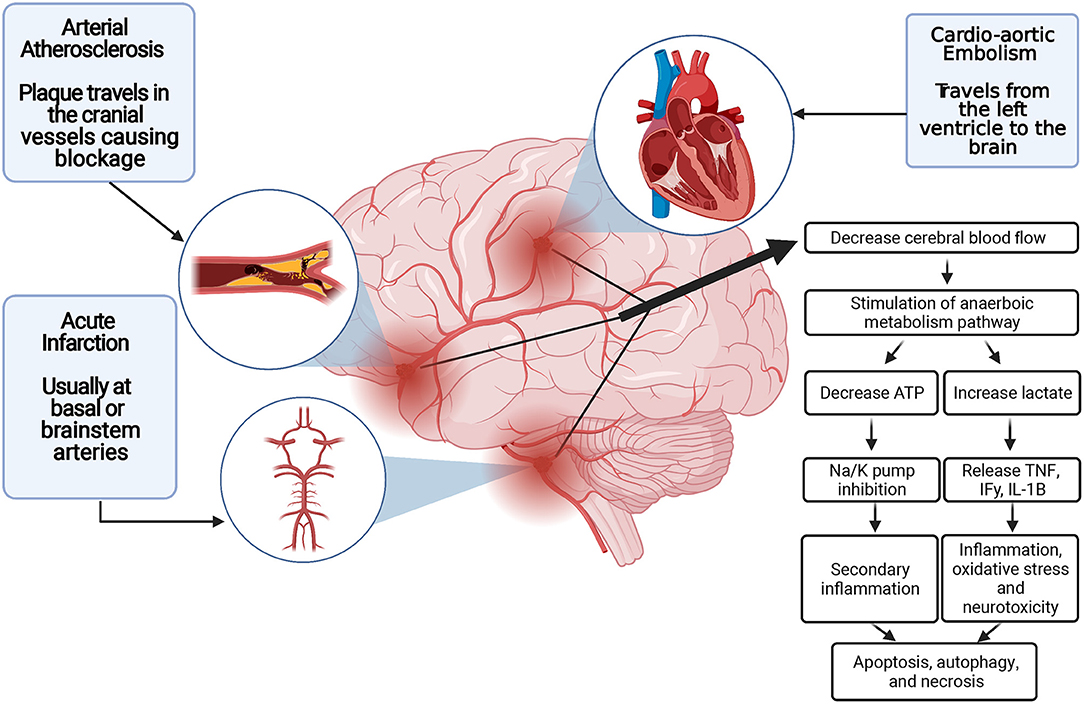
Figure 1. Blood supply disruption and ischemic stroke pathogenesis. ATP indicates adenosine triphosphatase; IFγ, Interferon gamma; IL-1B, interleukin 1B; K, potassium; Na, sodium; TNFα, tumor necrosis factor α.
Gold Standards for the Management of AIS
Recombinant Tissue Plasminogen Activator
The American Heart Association/American Stroke Association (AHA/ASA) guidelines have emphasized the efficacy of rtPA in the early management of AIS and a notable improvement in outcomes. rtPA helps recanalize the occluded blood vessels through the lysis of the blood clot and subsequently the reperfusion of brain tissue. The best results of rtPA can be obtained when administered intravenously within 3 h from the onset of symptoms of AIS (27, 28). In another study, a longer time window of 4.5 h was shown to be effective and safe in the management of AIS (29). In addition, several clinical trials (e.g., NINDS, ECASS, ECASS II, ATLANTIS, PROACT, and others) have studied the safety and effectiveness of using rtPA with a time window of 6 h (30, 31). However, some adverse effects were reported from the administration of rtPA in a 6-h time window. The most severe adverse effects included increased mortality and a high incidence of intracranial hemorrhage, which occurred less frequently in the 3-h or 4.5-h time windows (32). Also, rtPA is contraindicated in the presence of intracranial hemorrhage (33). Approximately 3 to 8% of patients with an ischemic stroke are eligible for IV thrombolytic therapy, with only 20 to 66% achieving complete recanalization. Furthermore, over 30% of patients who did achieve complete recanalization suffer from a neurologic injury due to re-occlusion (34, 35).
More recent clinical trials emphasized the importance of imaging-guided thrombolysis in patients with AIS, with a non-definite time of onset. Although this approach will enhance the efficacy of detecting thrombolysis candidates (36), some limitations, including far distance to the scanner, time spent in screening, and cost, might reduce this efficacy. Moreover, the eligibility for IV-tPA was evaluated by CT-P imaging in the Thrombolysis Guided by Perfusion Imaging up to 9 H after Onset of Stroke (EXTEND) clinical trial. The authors demonstrated that this approach could extend revascularization time to 24 h and the safety and efficacy of IV-tPA to 9 h (37).
More recent trials also investigated using a novel thrombolytic agent, tenecteplase, with high fibrinogen specificity, increasing its efficacy. Moreover, it has a long half-life and can be administered as a single bolus. This has been indicated in the Tenecteplase vs. Alteplase before Thrombectomy for Ischemic Stroke (EXTENT-IA-TNK) trial, indicating better functional outcomes and higher reperfusion rates than patients with AIS that were administered alteplase and were eligible for endovascular thrombectomy (EVT) (38, 39). Other studies also concluded that the efficacy and safety of tenecteplase and alteplase are similar when given to patients without large vessel occlusion (LVO) (40, 41). However, this modality is not FDA-approved and is not recommended by the AHA/ASA guidelines at the same level as alteplase.
Endovascular Thrombectomy
Treatment of ischemic stroke caused by LVO has been routinely managed using endovascular approaches (42–46). This approach consists of the interventionalist guiding a microcatheter from the groin or arm into the blood clot under X-ray guidance. However, as with thrombolytic pharmacologic therapy, many people are deemed ineligible for endovascular treatment. A study found that only 7 to 13% of patients with ischemic stroke were eligible candidates, with poor outcomes reported in up to 49% of participants and failure seen in 41% (42–47). Ineligibility is based on the timeframe, with large and small occlusions having a 6- and 24-h cutoff, respectively (48, 49). Another study showed the prior usage of rtPA before EVT has not shown substantial benefit in the outcomes of this procedure (50). Notably, the product of an EVT is not readily available due to its highly specialized nature with specific imaging equipment required to see cranial vessels (51).
Strategies to rescue ischemic stroke now include transduction blockage in downstream pathways. This includes the binding of glutamate to inhibit receptor stimulation and the antagonism of detrimental N-methyl-D-aspartate (NMDA) receptors selectively. These various mechanisms have all shown promise for ischemic stroke patients with minimal adverse effects (52).
Preclinical Models for Studying Neuroprotection
Neuroprotection research has found that testing works in animal models, but the outcomes in clinical trials have been disappointing. A rat stroke model (middle cerebral artery occlusion) revealed that even single whisker stimulation could induce complete protection of the rat cortex (53). Over 1,000 neuroprotective agents have been tested in preclinical stroke studies, with many showing promising success (54). Nearly 200 neuroprotective clinical trials have been performed, but only a tiny percentage of them have been successful (16, 54, 55). Sadly, substantial gaps in the literature still exist between animal studies and clinical trials on stroke. The aspects they can test help us understand the physiologic parameters of new drugs while giving our neuroprotective targets validity (56), although much is still lacking. For instance, it is known that the rodent most commonly used in preclinical stroke research cannot mimic the typical AIS patient (57).
Furthermore, some facets of ischemic stroke are not feasible in animal models, including preventative strategies (56). Although comorbidities such as diabetes and hypertension can be induced into the rodents being tested, the cost is high (58). Thus, most mice being tested are young and healthy males, although female mice account for a majority of stroke mortalities (59). These preclinical stroke model differences have led to a large gap between animal studies and clinical trials (56). With the utility of comorbidities and preclinical randomized control trials (RCTs) and studying the clinical setting, we may be able to close the gap leading to an increased understanding of AIS (56).
Neuroprotective Strategies and Agents
The main aim of the management of AIS is to restore the blood flow to the affected ischemic part of the brain. In contrast, the secondary goals include the modulation of any process that may aggravate the irreversible nerve cell damage induced by ischemia to the surrounding ischemic area and enhance the functional outcomes of the disease (56). Neuroprotection is studied based on understanding the pathophysiology of AIS to achieve these goals. Neuroprotection is the process that prevents the cellular injury of the brain in the setting of ischemia. This protection encompasses either interventional or pharmaceutical treatments (16). As a result, any pharmacologic or interventional technique that mitigates or prevents the harmful molecular and cellular activities that contribute to permanent cerebral ischemia is referred to as neuroprotection (16). Neuroprotective and collateral treatment in stroke tends to be more successful when delivered as soon as possible after the onset of symptoms. To minimize the time from stroke to needle, care should preferably begin before admission to the hospital (60). American Heart Association guidelines currently do not promote pharmacologic agents with putative neuroprotective actions, except for research purposes (33). Having adequate collateral blood flow to the brain has been shown to decrease infarct expansion leading to improved patient outcomes and prognosis in ischemic stroke patients (61–63). Thus, utilizing collateral blood flow to the ischemic area of the brain also presents physicians with another treatment option other than immediate clot thrombosis and recanalization (64).
Furthermore, studies show the importance of neuroprotection therapy together with reperfusion modalities. In this context, a recent systematic review showed that 5 out of 15 clinical trials indicated that patients treated with neuroprotection and reperfusion therapy had good clinical outcomes (65). These findings indicate the promising clinical future of using neuroprotection therapy for patients with AIS.
Therapeutic Hypothermia
Therapeutic hypothermia has proved its efficacy as a neuroprotective strategy with promising outcomes in protecting nerve injuries in humans. Therapeutic hypothermia as a neuroprotective strategy was shown to improve the prognosis of neurologic injuries in experimental animals and cardiac arrest patients (66). It works by many mechanisms to achieve neuroprotection. Reducing the basal metabolic rate through preserving glucose, PH, and ATP in the brain tissue is a critical mechanism for establishing therapeutic hypothermia's role as a neuroprotectant (67, 68). Other mechanisms include the inhibition of free radicals and nitric oxide generation (both play a role in nerve cell damage), modulation of the inflammatory processes that accompany and may aggravate the neuronal injuries, in addition to inhibition of nerve cell apoptosis and the protection against excitotoxic agents (as reported in some rat model studies) (69–71). Therapeutic hypothermia can be achieved through two different methods, either physical hypothermia or pharmacologic (drug-induced) hypothermia. Physical hypothermia can be obtained locally through a cooling helmet to get local and selective brain hypothermia (72) or generally through cooling blankets or ice pads and rapid infusion of cold saline (73).
On the other hand, many classes of drugs, such as diazepam, dopamine agonists, opioids, adenosine derivatives, and others, were reported to induce hypothermia (74, 75). However, therapeutic hypothermia has proved its efficacy as a neuroprotective treatment; it was found that therapeutic hypothermia in combination with other neuroprotectants with various mechanisms was more successful and gave better outcomes than administration of a single neuroprotective agent (16, 76). So it is advisable to apply therapeutic hypothermia in combination with other agents (77).
Ischemic Conditioning
Triggering mechanisms implicated in endogenous ischemic tolerance represents a very promising strategy to protect neurons against an ischemic insult (78). In this context, preconditioning, or the more clinically feasible postconditioning, with a brief period of ischemia or with a chemical challenge, is a practical approach to decrease neuronal death caused by a more severe ischemic episode (79, 80). These studies have found that neuronal cell death is minimized through preconditioning or postconditioning of a short period of ischemia or chemical challenge (79, 80). Meaning that once there has been a mild neurotransmitter stress (i.e., glutamate), the patient is left in a state tolerant of a more severe level of glutamate exposure.
Previous in vitro studies have established that preconditioning induced by glutamate or sublethal oxygen-glucose deprivation in cultured neurons is mediated by activation of NMDA receptors (81–83). It was also discovered that ischemic preconditioning increased γ-aminobutyric acid (GABA) production and release during lethal cerebral ischemia and its effects on glutamate neurotransmission (78, 84). Ischemia tolerance relies on conformational changes of both the presynaptic and postsynaptic GABA receptors causing a shift in the glutamate and GABA neurotransmitter balance (85). Importantly, different sodium-calcium exchanger isoforms have also been found to play a role in both precondtioning and postconditioning (86).
3K3A-Activated Protein C
Activated protein C (APC) is an anti-inflammatory and anticoagulant factor that was reported to have a neuroprotective role in ischemic stroke in many animal studies and a few human studies. It conducts its neuroprotection by inhibiting cell apoptosis through its action on endothelial protein C receptor and protease-activated receptor-1 (PAR-1) (87). The PAR-1 agonist (3K3A-APC) has shown promise in previous clinical trials in lowering neurologic injury and promoting vascular integrity. One study found that when 3K3A-APC was used in combination with rtPA, hemorrhage occurrence was reduced (88). It was also found that infarct size and an increased therapeutic window were seen when utilizing 3K3A-APC with rtPA in older female rodents suffering from acute high blood pressure. However, no additional benefit was seen if rtPA was not administered within the first 4 h after the onset of ischemia. More recent trials did appear to show the same lowered hemorrhage rates, although the authors stated experimental confirmation should be completed (89). However, human studies about 3K3A-APC brain cytoprotection in ischemic stroke patients are still deficient and need more evaluation and assessment before using this agent in humans safely. Hence, many efforts are directed to examine the effectiveness of the signaling-selective 3K3A-APC variant to upgrade its use from the preclinical to the clinical stage (90–92).
NXY-059
NXY-059 is an agent that reduces free radicals. Free radicals are involved in brain cell death in AIS (15, 22–24). Through its free radicals trapping property, NXY-059 was reported in a clinical trial (SAINT I) to have substantial neuroprotective efficacy when administered intravenously within 6 h after stroke onset, reducing the rate of 90-day disability (93). Contrastingly, the SAINT II clinical trial showed a 72-h IV infusion of NXY-059 in patients with acute stroke which was shown to be ineffective in improving stroke outcomes (94). After the confusing and controversial results from SAINT I and SAINT II, Antonic et al.'s (95) recent experiments about the efficacy of NXY-059 to reach a clear conclusion about its effectiveness have reported the failure of NXY-059 as a neuroprotective agent.
Nitric Oxide
Vascular nitric oxide concentrations are low in acute stroke and associated with poor outcomes, therefore, allowing the possibility that supplemental nitric oxide might be beneficial (96). Preclinical stroke studies found nitric oxide donors improved regional cerebral blood flow, and the lesion size was reduced if administered rapidly (22, 97). Therefore, a nitric oxide donor transdermal glyceryl trinitrate has been tried in the setting of acute stroke and randomized trials have shown that it was effective in improving multiple acute stroke hemodynamic parameters. These positive results included decreased central and peripheral blood pressure, decreased pulse pressure, and decreased blood pressure at 24 h while improving the augmentation index (96, 98–101). The timeframe of administration of transdermal glyceryl trinitrate has been shown to vary between different test studies, with some suggesting its utility within 4 h of stroke (101), while others say within 6 h (98, 102). A large study in the United Kingdom showed that the utility of prehospital transdermal glyceryl trinitrate in the prehospital setting did not improve patient outcomes with assumed stroke (103).
Magnesium Sulfate
Magnesium sulfate was documented to be a potent neuroprotectant, especially if given antenatally, where it considerably reduced the incidence of cerebral palsy and death (104). Since that study, many preclinical and clinical studies investigated its neuroprotection in ischemic stroke (105). Magnesium sulfate's neuroprotective effects come mainly from its antagonism to NMDA glutamate receptors, enhancing the blood flow to the ischemic areas and improving the metabolic recovery after ischemia (106–108). Many RCTs targeted the safety and probability of magnesium sulfate as one type of management in ischemic stroke (109–112). For example, the recent FAST-MAG trial showed the safety of prehospital application of magnesium sulfate therapy within 2 h from stroke onset. But it also revealed a slight improvement in stroke outcomes in the 90-day disability measured by scores on the modified Rankin scale (112).
NA-1 Delivery
When glutamate levels are elevated in the extracellular space, it leads to the activation of kainate glutamate, NMDA, and α-amino-3-hydroxy-5-methyl-4-isoxazole propionate acid receptors. This results in the influx of calcium which ultimately leads to damage of cells in ischemic infarct (113). Thus, blocking these receptors has been theorized as a possible treatment for ischemic stroke. However, the utility of the NMDA receptor antagonists on ischemic stroke has been shown to have a very tight timeframe for inducing positive effects. The receptors are significantly impaired before 2 h of ischemia onset, while reduced NMDA receptor density is observed in periods exceeding 2 h (114). Thus, the primary reason for failure in the clinical trials is due to immediate toxicity rather than the delayed recovery of the NMDA antagonist blockage mechanism (115).
The drug NA-1 has been shown to lessen the excitotoxic signaling of NMDA postsynaptic signaling. This is accomplished through targeting the 95 protein (116). Intervention studies before endovascular aneurysm surgery have shown that pretreatment of NA-1 before an operation decreased the frequency of iatrogenic stroke (ENACT trial) (117). This drug has also been tested in macaques known to have genetics similar to humans with positive behavioral findings and magnetic resonance imaging post-ischemic stroke (118). NMDA receptor stimulation, rather than inhibition, was influential in the subacute period following stroke, leading to plasticity and memory formation (119, 120). Despite the blockade of mechanisms of post-ischemic repair, the primary reason for the failure of clinical trials with NMDA antagonists was related to immediate toxicity rather than delayed recovery. The latest RCTs of EVTs performed in 2015 recognized critical points in the clinical trial design (faster revascularization and selection of patients with a limited core or presence of occlusion) and yielded promising results (42–46).
Statins
Statin therapy has been shown to have utility in both primary and secondary stroke prevention (121, 122). Furthermore, statins have also improved recovery times and survival 1-week post-AIS (123). However, after the onset of stroke, the discontinuation of statin therapy has been shown to worsen outcomes, whereas the initiation of statins has been shown to do the opposite (124). In NeuSTART, the administration of lovastatin (8 mg/kg) 3 days post-AIS was well tolerated (125). In the multicenter ASSORT RCT performed in Japan, Yoshimura et al. compared early (within 24 h) or delayed (on day 7 of AIS onset) administration of statin therapy in patients with AIS and dyslipidemia. The RCT had shown an insignificant difference and almost the same outcome in both early and delayed administration of statins (126).
Glyburide
Glyburide is a second-generation sulfonylurea used as an oral hypoglycemic drug in control of diabetes. It has been demonstrated that glyburide effectively blocks the SUR1-TRPM4 non-selective cation channels (127, 128). These channels are upregulated and reported to mediate cytotoxic brain swelling and subsequently brain cell oncotic necrosis in the setting of ischemia (129). Therefore, the blockade of these receptors can assist in nerve cell protection in cases of AIS. Results of preclinical animal studies emphasize the theory of glyburide's role as a neuroprotectant in AIS (127, 130). GAMES-RP, a double-blind, randomized phase II trial, reported the safety and well-tolerated use of glyburide in critically ill patients without substantial improvement of the disability or the need of craniotomy to alleviate brain edema compared to placebo (131, 132). An exploratory analysis of the GAMES-RP trial followed and showed the influential role of IV glyburide therapy in improving the survival rates in patients 70 years or younger with acute hemiplegic infarction (133). Further investigation in a phase III trial is warranted.
Insulin
Hyperglycemia is common when the infarct size is large in AIS patients (134). Conversely, persistent hyperglycemia following stroke was found to exacerbate the infarct size and subsequently give poor functional outcomes (133, 135). Additionally, Bruno et al. (136) reported in the NINDS rt-PA Stroke Trial that the presence of hyperglycemia in patients with stroke was associated with an increased risk of hemorrhagic events regardless of rtPA therapy. High blood glucose was also found to negatively impact the mortality rates in events of AIS (137). Hence, controlling blood glucose levels in stroke patients is crucial in improving the outcomes and protecting nerve cells from damage after exposure to ischemia. Insulin administration in cases of AIS was found to have beneficial neuroprotective effects. Reversal of the pathology produced by hyperglycemia [e.g., reducing the blood flow to the ischemic penumbra and increasing NMDA receptor-mediated nerve damage and glucose-induced inflammation and oxidative stress (138–140)] is one of the mechanisms by which insulin achieves its neuroprotection (141). In addition, insulin exerts its neuroprotective actions by blood glucose reduction and other mechanisms. These mechanisms include promoting glycogen synthesis, enhancing the functions of the neurotransmitters, and suppressing the process of neuronal apoptosis and necrosis, in addition to its antioxidant and anti-inflammatory effects (141, 142). Insulin is given through two different modes of administration, either standard or intensive modes. Standard insulin therapy involves subcutaneous insulin administration on a sliding scale every 6 h as needed to keep a blood glucose level between 80 and 179 mg/dL, while intensive insulin therapy is referred to as the continuous infusion of insulin intravenously as needed to keep the blood glucose levels between 80 and 130 mg/dL. Both methods are compared by the SHINE trial, a phase III RCT (143). SHINE examined the 90-day outcomes based on the modified Rankin scale score between the standard and the intensive insulin therapy. The trial concluded that there were no notable differences in the outcomes between the two groups (144), thereby concluding either method of administration can achieve the benefits of insulin therapy in stroke patients. Another route of insulin administration that has proved its efficacy on animals is intranasal insulin administration. Zhu et al. showed promising results from their experiment on animals, concluding that intranasal insulin could ameliorate neuronal dysfunction in mice with hemorrhagic stroke. Human studies are needed to check the efficacy of this method application, as it is recommended to use insulin therapy with any cases of AIS regardless of the glucose level (142).
Neu2000KWL
Neu2000KWL is a new multitarget drug designed to prevent excitotoxicity and oxygen toxicity by reducing NMDA receptor activity and the toxic effects of free radicals (145). Many ongoing trials are investigating the effectiveness of Neu2000KWL in protecting brain cells in cases of AIS. The ENIS I trial examines the safety and efficacy of Neu2000KWL in ischemic stroke (146). Once thromboembolism has occurred, nine consecutive infusions of Neu2000KWL at 12-h intervals are administered just before endovascular therapy with mechanical thrombectomy to follow (NCT02831088) (147). The trial is still ongoing and aims to investigate the safety of neuroprotection with Neu2000KWL and its effect on improving the outcomes when given before EVT. The outcomes of the trial were compared based on the modified Rankin scale at 3 months.
Another ongoing trial exploring the efficacy of Neu2000KWL as a neuroprotectant in patients with AIS is being processed in Beijing, China. The trial tests the outcomes of AIS after administration of Neu2000KWL infusion within 6 h from the onset of stroke symptoms followed by nine consecutive infusions at 12-h intervals. It will also compare the efficacy of different doses of the neuroprotective agent, either low, middle, or high. The outcomes of the study will be measured based on the National Institutes of Health Stroke Scale score of the involved patients. A score of 0 to 1 within 14 ± 2 days from the first injection represents a favorable outcome in those patients. Although the drug has not been approved yet by the US Food and Drug Administration to be used in cases of AIS, many reports see a promise in the efficacy of the drug as a neuroprotective medication.
Nimodipine
The calcium channel blocker nimodipine has also been studied for stroke patients due to its vasodilatory effects. Furthermore, nimodipine was shown to have the most potent vasodilation of all the calcium channel blockers, and this has shown utility in multiple cognitive treatments. However, the overall benefits were suboptimal despite the stroke size (148, 149). Interestingly, those who received their medication intravenously showed worse outcomes compared to those that received it by other means within a 12-h window (148, 149). Some of the benefits of nimodipine treatment include decreased hypertension-related intracranial vascular changes (150) and improved cognition (151–155). These benefits suggest nimodipine may play a neuroprotective role. When nimodipine was utilized early in subarachnoid hemorrhage patients, it decreased the neuronal damage induced by vasospasms (156). However, it has not been proven to reduce the incidence of angiographically documented delayed cerebral vasospasms (157–160).
Antioxidants and Antibodies
Oxidative stress is known to cause great insult to the brain in cases of ischemia. It is caused by oxygen and nutrient deprivation to brain cells, resulting in an imbalance between free radicals and the opposing antioxidant mechanisms (56, 161). Levels of antioxidants have been shown to correlate with the outcomes of stroke patients. Two naturally occurring and easily administered antioxidants that have been tested are uric acid and albumin. They have various mechanisms for why they work to reduce stroke risk, including removing harmful free oxygen radicals (162–164). The oxidative stress of free radicals is via the compounds superoxide, hydroxyl, nitric oxide, and peroxynitrite. The damage of these free radicals is further worsened by the reduction in scavenger enzymes in stroke due to energy depletion (165). Several antioxidant therapies are used in patients of AIS as neuroprotective agents to reduce the damage predisposed by the oxidative stress caused by increased free radical concentration (161). Edaravone is a free radical scavenger and a potent antioxidant against the peroxidation of the phosphatidylcholine liposomal membrane and reduction of ROS generation (166, 167). Many trials in Asian countries were first performed to check its safety and efficacy as a neuroprotectant (167). Its utilization as a neuroprotectant was first approved in Japan in 2001, and its administration in AIS is recommended by the Japanese guidelines in stroke management (168). In their systematic review of RCTs, Feng et al. (169) showed that the use of edaravone in patients with AIS resulted in substantial improvement in the neurologic functions as compared to placebo. Another recent large retrospective observational study suggested using edaravone in combination with endovascular reperfusion therapy to get the best outcomes of the intervention (170).
When brain ischemia occurs, ROS generation (edaravone inhibits this process) opens the mitochondrial permeability transition pore. Triggering of the mitochondrial permeability transition pore results in further release of ROS, a process called ROS-induced ROS release. This process is inhibited by cyclosporin A (167, 171). Cyclosporin A was shown to reduce the reperfusion-induced metabolic damage of brain nerve cells (172). Nighoghossian et al. demonstrated that IV cyclosporine A did not significantly reduce the infarct size when used in combination with thrombolytic therapy. However, it potently reduced the proximal cerebral artery occlusion resulting in successful recanalization (173). Due to the complementary role of both, edaravone and cyclosporine A can be used effectively in combination to achieve the best neuroprotection (167).
A multicentered trial on anti-CD49d (integrin alpha 4) antibodies has been completed to assess their ability to stop the migration of inflammatory cells (i.e., leukocytes) into the brain after the onset of ischemic stroke. This treatment would lower the generation of free radical damage mediated by the infiltrating peripheral leukocytes during the inflammatory response (174). Sadly, initial findings showed that only permanent stroke victims benefitted from the anti-CD49d treatment, whereas transient ischemic stroke patients did not (175). Recently, in an attempt to stop leukocyte adhesion and migration during stroke infarct, natalizumab (a monoclonal antibody against the leukocyte adhesion molecule α4 integrin) was tested in preclinical trials (175–177). The safety and efficacy of natalizumab on human participants were evaluated in the RCT ACTION. This trial showed no improvement in infarct size when administration occurred within a 9-h window of ischemia onset (178). However, there were improvements in the functional outcomes following its use, enhancing its role in neuroprotection (178).
Positive Modulators of GABA Receptors, Transaminase Inhibitors, or Transporter Blockers
The rapid and transient GABA elevation in the extracellular space during cerebral ischemia acts like most neurotransmitters (179, 180). Early in the reperfusion phase, disruption of GABA-mediated neurotransmission can lead to ongoing neuronal excitability and probably neuronal death (181, 182). Importantly, the different receptors of GABA have been shown to affect the brain differently, and utilizing the different traits of the glutamate and GABA receptors has helped reduce toxicity and increase efficiency (52).
There is a transient increase in ligands binding to inhibitory GABA-A receptors in the preconditioned in vivo hippocampus (183). Neuroprotection is abolished with weak antagonism of the GABA-A receptors and bicuculline as determined by viewing ischemic preconditioned rat hippocampal slices (184). Activation of GABA-B receptors has been suggested to support the role of hippocampal neuron neuroprotection (84). Simply put GABA-A receptors are associated with ischemic intolerance, whereas GABA-B receptors seem to be a component of neuroprotection (84, 183).
When endogenous GABA levels are pharmacologically increased, both GABA-A and GABA-B receptors are triggered, contributing to neuroprotection (185). Thus, GABA drugs that function as agonists or positive modulators of GABA receptors, GABA transaminase inhibitors, or GABA transporter blockers provide neuroprotection in the preclinical environment (186). When utilizing GABA in treating ischemic stroke, one will have to consider where the stroke occurred. The expression of both GABA-A and GABA-B receptors decreases in different brain regions of rodents subjected to transient ischemia (187).
Glial Cells as a Neuroprotection Target
The cells of the brain have been shown to play a role in the ischemic stroke response. Cells that have been shown to play a role include the microglia and the astrocytes. While they both release proinflammatory cytokines in ischemic stroke, the microglia also release toxic metabolites and enzymes (188, 189), whereas the astrocytes release neuroprotective factors (190). In addition, microglial cells and monocytes also play a role in ischemic stroke injury via increases in free radicals through elevated nitric oxide synthesis (22) (Figure 2).
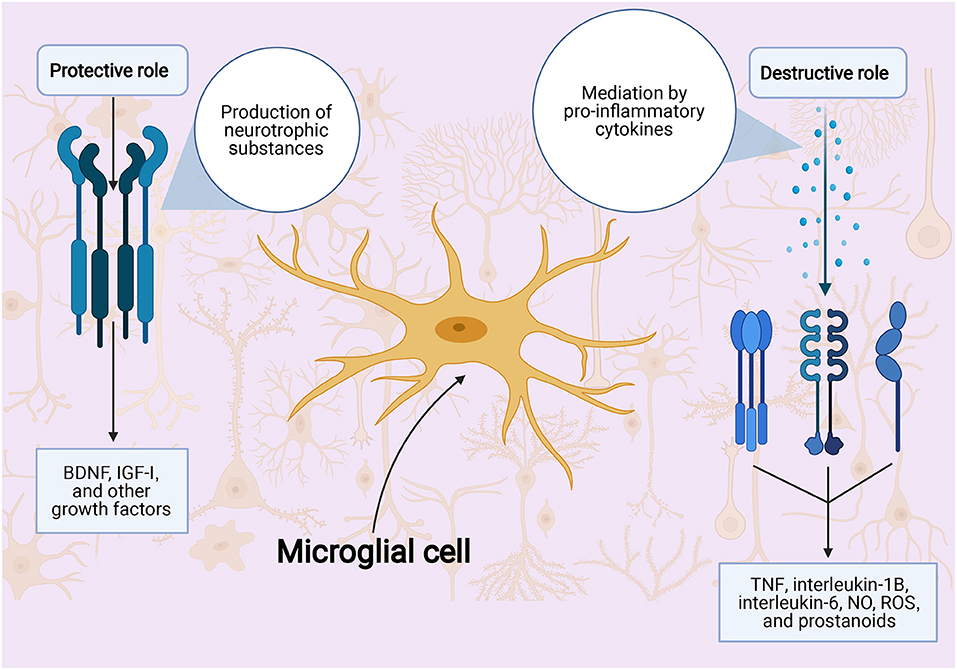
Figure 2. Role of microglial cells in ischemic stroke injury. BDNF indicates: NO, nitric oxide; ROS, reactive oxygen species; TNFα, tumor necrosis factor α.
The sphenopalatine ganglion is known to have multiple benefits when stimulated, including increased collateral blood flow to the brain and reduction in the size of infarct in ischemic stroke patients. A recent study found that it is safe to stimulate the sphenopalatine ganglion within the first 8 to 24 h of stroke symptoms. Although these are still only clinical trials, they show promise in benefit outcomes of stroke, but not in mortality (191). It has also been reported that upon sphenopalatine ganglion stimulation in vitro, there was normalized brain function with decreased blood-brain barrier dysfunction when applied 24 h after ischemic stroke (192). Changes caused by increased inflammatory markers can lead to changes in the endothelial cell layer, causing blood-brain barrier dysfunction (188) and more wound healing post-recovery due to infiltration of leukocytes from circulation (193–196). These findings suggest that cellular regulation and stimulation may be helpful in ischemic stroke patients even when outside the therapeutic window.
Stem Cells as a Neuroprotection Agent
The basis of using stem cells in the therapy of ischemic stroke patients is to replace the damaged neuronal tissue with new healthy cells. In recent years, experiments have proven stem cells to be effective in treating AIS while having very few adverse effects (197). The mechanism behind this includes anti-inflammation, antiapoptosis, antioxidative, blood-brain barrier protection, and the promotion of angiogenesis and neurogenesis (198, 199). In addition, stem cells have been shown to lend support to the at-risk tissues surrounding the infarct itself (200, 201). Thus, due to its relatively low cost and multiple delivery routes, the use of stem cell therapy in stroke patients should be investigated and utilized further.
Future Implications
We believe that our paper has adequately discussed the potential therapeutic and neuroprotective modalities for improving neurologic outcomes after AIS. We also believe that the currently ongoing trials about the various neuroprotective agents will add to the literature and lead to better evidence by indicating or inhibiting the previous and currently announced potential neuroprotective modalities. Future trials investigating the clinical outcomes of AIS patients using neuroprotection strategies/agents are encouraged. It is also expected that these trials will overcome the current limitations, regarding heterogeneity in reporting a standardized measure of functional and clinical outcomes of patients. Furthermore, future investigations should attempt to validate proven efficacious in vitro neuroprotective agents to make the best use of them.
Future research should also focus on the efficacy of adding neuroprotective treatment to endovascular treatment, as suggested that reperfusion together with neuroprotection is associated with enhanced outcomes after AIS. However, rapid reperfusion might be limited by inducing reperfusion injury. This has been attributed to increased ROS levels in previously ischemic tissues, secondary to reperfusion-related increases in oxygen levels. Therefore, it can be suggested that ROS-related neuroprotection modalities might be the ideal candidates to combine with reperfusion therapy and should be an area of focus for future investigations.
Moreover, stem cell-based treatment modalities are also promising treatments that will potentially influence the prevention of AIS and neuroprotection. Previous investigations conducted in preclinical models of AIS have been published, showing promising results (202, 203). Accordingly, many intentions have been declared to conduct similar human trials for adequate validation of these modalities, although the early findings are not appreciably promising (204).
Future preclinical investigations should also aim at enhancing the mechanism of action and targeting of neuroprotective agents. According to the Stroke Treatment Academic Industry Roundtable (STAIR) X, the term neurovascular unit (NVU) can be used to identify different brain parts that are at higher risk of ischemia. It has been shown that NVU includes various cell types, like endothelial cells, astrocytes, and neurons that are susceptible to ischemia (205). Accordingly, future research studies might concentrate on neuroprotective agents enhancing the prognosis of different NVU areas (206).
Conclusion
The management of AIS is time-dependent and needs urgent implementation to enhance prognosis. In the present literature review, we have discussed the advantages and complications of the most commonly described therapeutic modalities, in addition to the currently available neuroprotective agents and the roles they play in enhancing prognosis. Urgent approaches that can help stabilize the state of the patient can enhance the outcomes. Validating the results of the ongoing trials and the current in vitro-proven efficacious modalities is needed.
Author Contributions
SG, WB, RK, and DK contributed to the conception, design of the work, and revising it critically for important intellectual content. AR handled the reviewer comments and revised the manuscript accordingly. All authors contributed to the acquisition, interpretation of data, drafting the work, agreed to be accountable for all aspects of the work in ensuring that questions related to the accuracy or integrity of any part of the work are appropriately investigated, resolved, and approved the final version of this manuscript to be published.
Conflict of Interest
The authors declare that the research was conducted in the absence of any commercial or financial relationships that could be construed as a potential conflict of interest.
Publisher's Note
All claims expressed in this article are solely those of the authors and do not necessarily represent those of their affiliated organizations, or those of the publisher, the editors and the reviewers. Any product that may be evaluated in this article, or claim that may be made by its manufacturer, is not guaranteed or endorsed by the publisher.
Acknowledgments
The authors gratefully acknowledge the Scientific Publications staff at the Mayo Clinic for providing copyediting support.
References
1. Sacco RL, Kasner SE, Broderick JP, Caplan LR, Connors JJ, Culebras A, et al. An updated definition of stroke for the 21st century: a statement for healthcare professionals from the American heart association/American stroke association. Stroke. (2013) 44:2064–89. doi: 10.1161/STR.0b013e318296aeca
2. Hatano S. Experience from a multicentre stroke register: a preliminary report. Bull World Health Organ. (1976) 54:541–53.
3. Lopez AD, Mathers CD, Ezzati M, Jamison DT, Murray CJ. Global and regional burden of disease and risk factors, 2001: systematic analysis of population health data. Lancet. (2006) 367:1747–57. doi: 10.1016/S0140-6736(06)68770-9
4. Go AS, Mozaffarian D, Roger VL, Benjamin EJ, Berry JD, Borden WB, et al. Heart disease and stroke statistics−2013 update: a report from the American heart association. Circulation. (2013) 127:e6–245. doi: 10.1161/CIR.0b013e31828124ad
5. Feigin VL, Forouzanfar MH, Krishnamurthi R, Mensah GA, Connor M, Bennett DA, et al. Global and regional burden of stroke during 1990-2010: findings from the global burden of disease study 2010. Lancet. (2014) 383:245–54. doi: 10.1016/S0140-6736(13)61953-4
6. Heiss WD, Graf R. The ischemic penumbra. Curr Opin Neurol. (1994) 7:11–9. doi: 10.1097/00019052-199402000-00004
7. Ramos-Cabrer P, Campos F, Sobrino T, Castillo J. Targeting the ischemic penumbra. Stroke. (2011) 42:S7–11. doi: 10.1161/STROKEAHA.110.596684
8. Broussalis E, Killer M, McCoy M, Harrer A, Trinka E, Kraus J. Current therapies in ischemic stroke. part A recent developments in acute stroke treatment and in stroke prevention. Drug discovery today. (2012) 17:296–309. doi: 10.1016/j.drudis.2011.11.005
9. Schwamm LH, Ali SF, Reeves MJ, Smith EE, Saver JL, Messe S, et al. Temporal trends in patient characteristics and treatment with intravenous thrombolysis among acute ischemic stroke patients at get with the guidelines-stroke hospitals. Circ Cardiovasc Qual Outcomes. (2013) 6:543–9. doi: 10.1161/CIRCOUTCOMES.111.000095
10. Tanaka K, Matsumoto S, Yamada T, Nagano S, Takase KI, Hatano T, et al. Temporal trends in clinical characteristics and door-to-needle time in patients receiving intravenous tissue plasminogen activator: a retrospective study of 4 hospitals in Japan. J Stroke Cerebrovasc Dis. (2019) 28:104305. doi: 10.1016/j.jstrokecerebrovasdis.2019.104305
11. Adams HP, del Zoppo G, Alberts MJ, Bhatt DL, Brass L, Furlan A, et al. Guidelines for the early management of adults with ischemic stroke: a guideline from the American heart association/American stroke association stroke council, clinical cardiology council, cardiovascular radiology and intervention council, and the atherosclerotic peripheral vascular disease and quality of care outcomes in research interdisciplinary working groups: the American academy of neurology affirms the value of this guideline as an educational tool for neurologists. Stroke. (2007) 38:1655–711. doi: 10.1161/STROKEAHA.107.181486
12. Sutherland BA, Minnerup J, Balami JS, Arba F, Buchan AM, Kleinschnitz C. Neuroprotection for ischaemic stroke: translation from the bench to the bedside. Int J Stroke. (2012) 7:407–18. doi: 10.1111/j.1747-4949.2012.00770.x
13. Broughton BR, Reutens DC, Sobey CG. Apoptotic mechanisms after cerebral ischemia. Stroke. (2009) 40:e331–9. doi: 10.1161/STROKEAHA.108.531632
14. Lipton P. Ischemic cell death in brain neurons. Physiol Rev. (1999) 79:1431–568. doi: 10.1152/physrev.1999.79.4.1431
15. Dirnagl U, Iadecola C, Moskowitz MA. Pathobiology of ischaemic stroke: an integrated view. Trends Neurosci. (1999) 22:391–7. doi: 10.1016/S0166-2236(99)01401-0
16. Ginsberg MD. Neuroprotection for ischemic stroke: past, present and future. Neuropharmacology. (2008) 55:363–89. doi: 10.1016/j.neuropharm.2007.12.007
17. Benveniste H, Drejer J, Schousboe A, Diemer NH. Elevation of the extracellular concentrations of glutamate and aspartate in rat hippocampus during transient cerebral ischemia monitored by intracerebral microdialysis. J Neurochem. (1984) 43:1369–74. doi: 10.1111/j.1471-4159.1984.tb05396.x
18. Globus MYT, Busto R, Dietrich WD, Martinez E, Valdes I, Ginsberg MD. Effect of ischemia on the in vivo release of striatal dopamine, glutamate, and γ-aminobutyric acid studied by intracerebral microdialysis. J Neurochem. (1988) 51:1455–64. doi: 10.1111/j.1471-4159.1988.tb01111.x
19. Hillered L, Hallström A, Segersvärd S, Persson L, Ungerstedt U. Dynamics of extracellular metabolites in the striatum after middle cerebral artery occlusion in the rat monitored by intracerebral microdialysis. J Cereb Blood Flow Metab. (1989) 9:607–16. doi: 10.1038/jcbfm.1989.87
20. Rothman SM, Olney JW. Glutamate and the pathophysiology of hypoxic–ischemic brain damage. Ann Neurol. (1986) 19:105–11. doi: 10.1002/ana.410190202
21. Lai TW, Zhang S, Wang YT. Excitotoxicity and stroke: identifying novel targets for neuroprotection. Progress Neurobiol. (2014) 115:157–88. doi: 10.1016/j.pneurobio.2013.11.006
22. Willmot M, Gray L, Gibson C, Murphy S, Bath PM. A systematic review of nitric oxide donors and L-Arginine in experimental stroke; effects on infarct size and cerebral blood flow. Nitric Oxide. (2005) 12:141–9. doi: 10.1016/j.niox.2005.01.003
23. Ishida K, Berger M, Nadler J, Warner DS. Anesthetic neuroprotection: antecedents and an appraisal of preclinical and clinical data quality. Curr Pharm Design. (2014) 20:5751–65. doi: 10.2174/1381612820666140204111701
24. Mattson MP. Roles of the lipid peroxidation product 4-hydroxynonenal in obesity, the metabolic syndrome, and associated vascular and neurodegenerative disorders. Exp Gerontol. (2009) 44:625–33. doi: 10.1016/j.exger.2009.07.003
25. Stokum JA, Gerzanich V, Simard JM. Molecular pathophysiology of cerebral edema. J Cereb Blood Flow Metab. (2016) 36:513–38. doi: 10.1177/0271678X15617172
26. Aronowski J, Zhao X. Molecular pathophysiology of cerebral hemorrhage: secondary brain injury. Stroke. (2011) 42:1781–6. doi: 10.1161/STROKEAHA.110.596718
27. Powers WJ, Rabinstein AA, Ackerson T, Adeoye OM, Bambakidis NC, Becker K, et al. Guidelines for the early management of patients with acute ischemic stroke: 2019 update to the 2018 guidelines for the early management of acute ischemic stroke: a guideline for healthcare professionals from the American heart association/American stroke association. Stroke. (2019) 50:e344–418. doi: 10.1161/STR.0000000000000211
28. National Institute of Neurological Disorders and Stroke rt-PA Stroke Study Group. Tissue plasminogen activator for acute ischemic stroke. N Eng J Med. (1995) 333:1581–7. doi: 10.1056/NEJM199512143332401
29. Hacke W, Kaste M, Bluhmki E, Brozman M, Dávalos A, Guidetti D, et al. Thrombolysis with alteplase 3 to 45 hours after acute ischemic stroke. N Engl J Med. (2008) 359:1317–29. doi: 10.1056/NEJMoa0804656
30. Ringleb PA, Schellinger PD, Schranz C, Hacke W. Thrombolytic therapy within 3 to 6 hours after onset of ischemic stroke: useful or harmful? Stroke. (2002) 33:1437–41. doi: 10.1161/01.STR.0000015555.21285.DB
31. Lees KR, Bluhmki E, von Kummer R, Brott TG, Toni D, Grotta JC, et al. Time to treatment with intravenous alteplase and outcome in stroke: an updated pooled analysis of ecass, atlantis, ninds, and epithet trials. Lancet. (2010) 375:1695–703. doi: 10.1016/S0140-6736(10)60491-6
32. Sandercock P, Wardlaw JM, Lindley RI, Dennis M, Cohen G, Murray G, et al. The benefits and harms of intravenous thrombolysis with recombinant tissue plasminogen activator within 6 h of acute ischaemic stroke (the third international stroke trial [ist-3]): a randomised controlled trial. Lancet. (2012) 379:2352–63. doi: 10.1016/S0140-6736(12)60768-5
33. Jauch EC, Saver JL, Adams HP, Bruno A, Connors JJ, Demaerschalk BM, et al. Guidelines for the early management of patients with acute ischemic stroke: a guideline for healthcare professionals from the American heart association/American stroke association. Stroke. (2013) 44:870–947. doi: 10.1161/STR.0b013e318284056a
34. Ribo M, Alvarez-Sabín J, Montaner J, Romero F, Delgado P, Rubiera M, et al. Temporal profile of recanalization after intravenous tissue plasminogen activator: selecting patients for rescue reperfusion techniques. Stroke. (2006) 37:1000–4. doi: 10.1161/01.STR.0000206443.96112.d9
35. Heo JH, Lee KY, Kim SH, Kim DI. Immediate reocclusion following a successful thrombolysis in acute stroke: a pilot study. Neurology. (2003) 60:1684–7. doi: 10.1212/01.WNL.0000063323.23493.98
36. Ma H, Campbell BCV, Parsons MW, Churilov L, Levi CR, Hsu C, et al. Thrombolysis guided by perfusion imaging up to 9 hours after onset of stroke. N Engl J Med. (2019) 380:1795–803.
37. Campbell BCV, Ma H, Ringleb PA, Parsons MW, Churilov L, Bendszus M, et al. Extending thrombolysis to 4·5-9 h and wake-up stroke using perfusion imaging: a systematic review and meta-analysis of individual patient data. Lancet. (2019) 394:139–47. doi: 10.1016/S0140-6736(19)31053-0
38. Parsons M, Spratt N, Bivard A, Campbell B, Chung K, Miteff F, et al. A randomized trial of tenecteplase versus alteplase for acute ischemic stroke. N Engl J Med. (2012) 366:1099–107. doi: 10.1056/NEJMoa1109842
39. Campbell BCV, Mitchell PJ, Churilov L, Yassi N, Kleinig TJ, Dowling RJ, et al. Tenecteplase versus alteplase before thrombectomy for ischemic stroke. N Engl J Med. (2018) 378:1573–82. doi: 10.1056/NEJMoa1716405
40. Burgos AM, Saver JL. Evidence that tenecteplase is noninferior to alteplase for acute ischemic stroke: meta-analysis of 5 randomized trials. Stroke. (2019) 50:2156–62. doi: 10.1161/STROKEAHA.119.025080
41. Logallo N, Novotny V, Assmus J, Kvistad CE, Alteheld L, Rønning OM, et al. Tenecteplase versus alteplase for management of acute ischaemic stroke (nor-test): a phase 3, randomised, open-label, blinded endpoint trial. Lancet Neurol. (2017) 16:781–8. doi: 10.1016/S1474-4422(17)30253-3
42. Berkhemer OA, Fransen PS, Beumer D, Van Den Berg LA, Lingsma HF, Yoo AJ, et al. A randomized trial of intraarterial treatment for acute ischemic stroke. N Engl J Med. (2015) 372:11–20. doi: 10.1056/NEJMoa1411587
43. Goyal M, Demchuk AM, Menon BK, Eesa M, Rempel JL, Thornton J, et al. Randomized assessment of rapid endovascular treatment of ischemic stroke. N Engl J Med. (2015) 372:1019–30. doi: 10.1056/NEJMoa1414905
44. Campbell BV, Mitchell PJ, Kleinig TJ, Dewey HM, Churilov L, Yassi N, et al. Endovascular therapy for ischemic stroke with perfusion-imaging selection. N Engl J Med. (2015) 372:1009–18. doi: 10.1056/NEJMoa1414792
45. Saver JL, Goyal M, Bonafe A, Diener H-C, Levy EI, Pereira VM, et al. Stent-retriever thrombectomy after intravenous T-Pa Vs. T-Pa alone in stroke. N Engl J Med. (2015) 372:2285–95. doi: 10.1056/NEJMoa1415061
46. Jovin TG, Chamorro A, Cobo E, de Miquel MA, Molina CA, Rovira A, et al. Thrombectomy within 8 hours after symptom onset in ischemic stroke. N Engl J Med. (2015) 372:2296–306. doi: 10.1056/NEJMoa1503780
47. Chia NH, Leyden JM, Newbury J, Jannes J, Kleinig TJ. Determining the number of ischemic strokes potentially eligible for endovascular thrombectomy: a population-based study. Stroke. (2016) 47:1377–80. doi: 10.1161/STROKEAHA.116.013165
48. Nogueira RG, Jadhav AP, Haussen DC, Bonafe A, Budzik RF, Bhuva P, et al. Thrombectomy 6 to 24 hours after stroke with a mismatch between deficit and infarct. N Engl J Med. (2018) 378:11–21. doi: 10.1056/NEJMoa1706442
49. Albers GW, Marks MP, Kemp S, Christensen S, Tsai JP, Ortega-Gutierrez S, et al. Thrombectomy for stroke at 6 to 16 hours with selection by perfusion imaging. N Engl J Med. (2018) 378:708–18. doi: 10.1056/NEJMoa1713973
50. Imbarrato G, Bentley J, Gordhan A. Clinical outcomes of endovascular thrombectomy in tissue plasminogen activator versus non-tissue plasminogen activator patients at primary stroke care centers. J Neurosci Rural Pract. (2018) 9:240–4. doi: 10.4103/jnrp.jnrp_497_17
51. English JD, Yavagal DR, Gupta R, Janardhan V, Zaidat OO, Xavier AR, et al. Mechanical thrombectomy-ready comprehensive stroke center requirements and endovascular stroke systems of care: recommendations from the endovascular stroke standards committee of the society of vascular and interventional neurology (Svin). Interv Neurol. (2016) 4:138–50. doi: 10.1159/000442715
52. Amantea D, Bagetta G. Excitatory and inhibitory amino acid neurotransmitters in stroke: from neurotoxicity to ischemic tolerance. Curr Opin Pharmacol. (2017) 35:111–9. doi: 10.1016/j.coph.2017.07.014
53. Lay CC, Davis MF, Chen-Bee CH, Frostig RD. Mild sensory stimulation completely protects the adult rodent cortex from ischemic stroke. PLoS ONE. (2010) 5:e11270. doi: 10.1371/journal.pone.0011270
54. O'Collins VE, Macleod MR, Donnan GA, Horky LL, van der Worp BH, Howells DW. 1,026 Experimental treatments in acute stroke. Ann Neurol. (2006) 59:467–77. doi: 10.1002/ana.20741
55. Lee J-M, Zipfel GJ, Choi DW. The changing landscape of ischaemic brain injury mechanisms. Nature. (1999) 399:A7–14. doi: 10.1038/399a007
56. Neuhaus AA, Couch Y, Hadley G, Buchan AM. Neuroprotection in stroke: the importance of collaboration and reproducibility. Brain. (2017) 140:2079–92. doi: 10.1093/brain/awx126
57. Dirnagl U, Endres M. Found in Translation: Preclinical Stroke Research Predicts Human Pathophysiology, Clinical Phenotypes, and Therapeutic Outcomes. Stroke. (2014) 45:1510–8. doi: 10.1161/STROKEAHA.113.004075
58. McColl B, Howells D, Rothwell N, Denes A. Modeling risk factors and confounding effects in stroke. In: Dirnagl U, editor. Rodent Models of Stroke. Neuromethods, Vol 120. New York, NY: Humana Press (2016). p. 93–122. doi: 10.1007/978-1-4939-5620-3_9
59. Mozaffarian D, Benjamin EJ, Go AS, Arnett DK, Blaha MJ, Cushman M, et al. Heart disease and stroke statistics-−2015 update: a report from the American heart association. Circulation. (2015) 131:e29–e322. doi: 10.1161/CIR.0000000000000152
60. Audebert HJ, Saver JL, Starkman S, Lees KR, Endres M. Prehospital stroke care: new prospects for treatment and clinical research. Neurology. (2013) 81:501–8. doi: 10.1212/WNL.0b013e31829e0fdd
61. Guenego A, Mlynash M, Christensen S, Kemp S, Heit JJ, Lansberg MG, et al. Hypoperfusion ratio predicts infarct growth during transfer for thrombectomy. Ann Neurol. (2018) 84:616–20. doi: 10.1002/ana.25320
62. Jung S, Wiest R, Gralla J, McKinley R, Mattle H, Liebeskind D. Relevance of the cerebral collateral circulation in ischaemic stroke: time is brain, but collaterals set the pace. Swiss Med Wkly. (2017) 147:w14538. doi: 10.4414/smw.2017.14538
63. Liebeskind DS. Collateral circulation. Stroke. (2003) 34:2279–84. doi: 10.1161/01.STR.0000086465.41263.06
64. Shuaib A, Butcher K, Mohammad AA, Saqqur M, Liebeskind DS. Collateral blood vessels in acute ischaemic stroke: a potential therapeutic target. Lancet Neurol. (2011) 10:909–21. doi: 10.1016/S1474-4422(11)70195-8
65. Vos EM, Geraedts VJ, van der Lugt A, Dippel DWJ, Wermer MJH, Hofmeijer J, et al. Systematic review - combining neuroprotection with reperfusion in acute ischemic stroke. Front Neurol. (2022) 13:840892. doi: 10.3389/fneur.2022.840892
66. Hakim SM, Ammar MA, Reyad MS. Effect of therapeutic hypothermia on survival and neurological outcome in adults suffering cardiac arrest: a systematic review and meta-analysis. Minerva Anestesiol. (2018) 84:720–30. doi: 10.23736/S0375-9393.18.12164-X
67. Schaller B, Graf R. Cerebral ischemia and reperfusion: the pathophysiologic concept as a basis for clinical therapy. J Cereb Blood Flow Metab. (2004) 24:351–71. doi: 10.1097/00004647-200404000-00001
68. Kuffler DP. Neuroprotection by hypothermia plus alkalinization of dorsal root ganglia neurons through ischemia. Ann N Y Acad Sci. (2010) 1199:158–63. doi: 10.1111/j.1749-6632.2009.05358.x
69. Gao XY, Huang JO, Hu YF, Gu Y, Zhu SZ, Huang KB, et al. Combination of mild hypothermia with neuroprotectants has greater neuroprotective effects during oxygen-glucose deprivation and reoxygenation-mediated neuronal injury. Sci Rep. (2014) 4:7091. doi: 10.1038/srep07091
70. Nilupul Perera M, Ma HK, Arakawa S, Howells DW, Markus R, Rowe CC, et al. Inflammation following stroke. J Clin Neurosci. (2006) 13:1–8. doi: 10.1016/j.jocn.2005.07.005
71. Ishikawa T, Marsala M. Hypothermia prevents biphasic glutamate release and corresponding neuronal degeneration after transient spinal cord ischemia in the rat. Cell Mol Neurobiol. (1999) 19:199–208. doi: 10.1023/A:1006973026514
72. Wang H, Olivero W, Lanzino G, Elkins W, Rose J, Honings D, et al. Rapid and selective cerebral hypothermia achieved using a cooling helmet. J Neurosurg. (2004) 100:272–7. doi: 10.3171/jns.2004.100.2.0272
73. Ikeda K, Ikeda T, Taniuchi H, Suda S. Comparison of whole-body cooling and selective head cooling on changes in urinary 8-hydroxy-2-deoxyguanosine levels in patients with global brain ischemia undergoing mild hypothermia therapy. Med Sci Monit Int Med J Exp Clin Res. (2012) 18:Cr409–14. doi: 10.12659/MSM.883208
74. Liu K, Khan H, Geng X, Zhang J, Ding Y. Pharmacological hypothermia: a potential for future stroke therapy? Neurol Res. (2016) 38:478–90. doi: 10.1080/01616412.2016.1187826
75. Neugebauer H, Schneider H, Bösel J, Hobohm C, Poli S, Kollmar R, et al. Outcomes of hypothermia in addition to decompressive hemicraniectomy in treatment of malignant middle cerebral artery stroke: a randomized clinical trial. JAMA Neurol. (2019) 76:571–9. doi: 10.1001/jamaneurol.2018.4822
76. Seyedsaadat SM, Marasco SF, Daly DJ, McEgan R, Anderson J, Rodgers S, et al. Selective brain hypothermia: feasibility and safety study of a novel method in five patients. Perfusion. (2020) 35:96–103. doi: 10.1177/0267659119853950
77. Sun YJ, Zhang ZY, Fan B, Li GY. Neuroprotection by therapeutic hypothermia. Front Neurosci. (2019) 13:586. doi: 10.3389/fnins.2019.00586
78. Wang Y, Reis C, Applegate R, Stier G, Martin R, Zhang JH. Ischemic conditioning-induced endogenous brain protection: applications pre-, per- or post-stroke. Exp Neurol. (2015) 272:26–40. doi: 10.1016/j.expneurol.2015.04.009
79. Pignataro G, Scorziello A, Di Renzo G, Annunziato L. Post-ischemic brain damage: effect of ischemic preconditioning and postconditioning and identification of potential candidates for stroke therapy. FEBS J. (2009) 276:46–57. doi: 10.1111/j.1742-4658.2008.06769.x
80. Constantino LC, Tasca CI, Boeck CR. The role of nmda receptors in the development of brain resistance through pre- and postconditioning. Aging Dis. (2014) 5:430–41. doi: 10.14336/AD.2014.0500430
81. Grabb MC, Choi DW. Ischemic tolerance in murine cortical cell culture: critical role for Nmda receptors. J neurosci. (1999) 19:1657–62. doi: 10.1523/JNEUROSCI.19-05-01657.1999
82. Mabuchi T, Kitagawa K, Kuwabara K, Takasawa K, Ohtsuki T, Xia Z, et al. Phosphorylation of camp response element-binding protein in hippocampal neurons as a protective response after exposure to glutamate in vitro and ischemia in vivo. J Neurosci. (2001) 21:9204–13. doi: 10.1523/JNEUROSCI.21-23-09204.2001
83. Lin CH, Chen PS, Gean PW. Glutamate preconditioning prevents neuronal death induced by combined oxygen-glucose deprivation in cultured cortical neurons. Eur J Pharmacol. (2008) 589:85–93. doi: 10.1016/j.ejphar.2008.05.047
84. Dave KR, Lange-Asschenfeldt C, Raval AP, Prado R, Busto R, Saul I, et al. Ischemic preconditioning ameliorates excitotoxicity by shifting glutamate/gamma-aminobutyric acid release and biosynthesis. J Neurosci Res. (2005) 82:665–73. doi: 10.1002/jnr.20674
85. Obrenovitch TP. Molecular physiology of preconditioning-induced brain tolerance to ischemia. Physiol Rev. (2008) 88:211–47. doi: 10.1152/physrev.00039.2006
86. Cuomo O, Vinciguerra A, Cerullo P, Anzilotti S, Brancaccio P, Bilo L, et al. Ionic homeostasis in brain conditioning. Front Neurosci. (2015) 9:277. doi: 10.3389/fnins.2015.00277
87. Cheng T, Liu D, Griffin JH, Fernández JA, Castellino F, Rosen ED, et al. Activated Protein C Blocks P53-mediated apoptosis in ischemic human brain endothelium and is neuroprotective. Nat Med. (2003) 9:338–42. doi: 10.1038/nm826
88. Wang Y, Zhang Z, Chow N, Davis TP, Griffin JH, Chopp M, et al. An activated protein c analog with reduced anticoagulant activity extends the therapeutic window of tissue plasminogen activator for ischemic stroke in rodents. Stroke. (2012) 43:2444–9. doi: 10.1161/STROKEAHA.112.658997
89. Lyden P, Pryor KE, Coffey CS, Cudkowicz M, Conwit R, Jadhav A, et al. Final results of the rhapsody trial: a multi-center, phase 2 trial using a continual reassessment method to determine the safety and tolerability of 3k3a-Apc, a recombinant variant of human activated protein C, in combination with tissue plasminogen activator, mechanical thrombectomy or both in moderate to severe acute ischemic stroke. Ann Neurol. (2019) 85:125–36. doi: 10.1002/ana.25383
90. Zlokovic BV, Griffin JH. Cytoprotective protein c pathways and implications for stroke and neurological disorders. Trends Neurosci. (2011) 34:198–209. doi: 10.1016/j.tins.2011.01.005
91. Griffin JH, Fernández JA, Lyden PD, Zlokovic BV. Activated Protein C promotes neuroprotection: mechanisms and translation to the clinic. Thromb Res. (2016) 141 Suppl 2:S62–4. doi: 10.1016/S0049-3848(16)30368-1
92. Griffin JH, Mosnier LO, Fernández JA, Zlokovic BV. 2016 scientific sessions sol sherry distinguished lecturer in thrombosis: thrombotic stroke: neuroprotective therapy by recombinant-activated protein C. Arterioscler Thromb Vasc Biol. (2016) 36:2143–51. doi: 10.1161/ATVBAHA.116.308038
93. Lees KR, Zivin JA, Ashwood T, Davalos A, Davis SM, Diener HC, et al. Nxy-059 for acute ischemic stroke. N Engl J Med. (2006) 354:588–600. doi: 10.1056/NEJMoa052980
94. Shuaib A, Lees KR, Lyden P, Grotta J, Davalos A, Davis SM, et al. Nxy-059 for the treatment of acute ischemic stroke. N Engl J Med. (2007) 357:562–71. doi: 10.1056/NEJMoa070240
95. Antonic A, Dottori M, Macleod MR, Donnan GA, Howells DW. Nxy-059, a failed stroke neuroprotectant, offers no protection to stem cell-derived human neurons. J Stroke Cerebrovasc Dis. (2018) 27:2158–65. doi: 10.1016/j.jstrokecerebrovasdis.2018.03.015
96. Rashid P, Weaver C, Leonardi-Bee J, Bath F, Fletcher S, Bath P. The effects of transdermal glyceryl trinitrate, a nitric oxide donor, on blood pressure, cerebral and cardiac hemodynamics, and plasma nitric oxide levels in acute stroke. J Stroke Cerebrovasc Dis. (2003) 12:143–51. doi: 10.1016/S1052-3057(03)00037-5
97. Maniskas ME, Roberts JM, Trueman R, Learoyd AE, Gorman A, Fraser JF, et al. Intra-arterial nitroglycerin as directed acute treatment in experimental ischemic stroke. J Neurointerv Surg. (2018) 10:29–33. doi: 10.1136/neurintsurg-2016-012793
98. Bath PM, Houlton A, Woodhouse L, Sprigg N, Wardlaw J, Pocock S. Statistical analysis plan for the ‘efficacy of nitric oxide in stroke'(enos) trial. Int J Stroke. (2014) 9:372–4. doi: 10.1111/ijs.12235
99. Bath PM, Pathansali R, Iddenden R, Bath FJ. The effect of transdermal glyceryl trinitrate, a nitric oxide donor, on blood pressure and platelet function in acute stroke. Cerebrovasc Dis. (2001) 11:265–72. doi: 10.1159/000047649
100. Willmot M, Ghadami A, Whysall B, Clarke W, Wardlaw J, Bath PM. Transdermal glyceryl trinitrate lowers blood pressure and maintains cerebral blood flow in recent stroke. Hypertension. (2006) 47:1209–15. doi: 10.1161/01.HYP.0000223024.02939.1e
101. Ankolekar S, Fuller M, Cross I, Renton C, Cox P, Sprigg N, et al. Feasibility of an ambulance-based stroke trial, and safety of glyceryl trinitrate in ultra-acute stroke: the rapid intervention with glyceryl trinitrate in hypertensive stroke trial (Right, Isrctn66434824). Stroke. (2013) 44:3120–8. doi: 10.1161/STROKEAHA.113.001301
102. Woodhouse L, Scutt P, Krishnan K, Berge E, Gommans J, Ntaios G, et al. Effect of hyperacute administration (within 6 hours) of transdermal glyceryl trinitrate, a nitric oxide donor, on outcome after stroke: subgroup analysis of the efficacy of nitric oxide in stroke (enos) trial. Stroke. (2015) 46:3194–201. doi: 10.1161/STROKEAHA.115.009647
103. Bath PM, Scutt P, Anderson CS, Appleton JP, Berge E, Cala L, et al. Prehospital transdermal glyceryl trinitrate in patients with ultra-acute presumed stroke (right-2): an ambulance-based, randomised, sham-controlled, blinded, phase 3 trial. Lancet. (2019) 393:1009–20. doi: 10.1016/S0140-6736(19)30194-1111
104. Crowther CA, Middleton PF, Voysey M, Askie L, Duley L, Pryde PG, et al. Assessing the neuroprotective benefits for babies of antenatal magnesium sulphate: an individual participant data meta-analysis. PLoS Med. (2017) 14:e1002398. doi: 10.1371/journal.pmed.1002398
105. Westermaier T, Stetter C, Kunze E, Willner N, Raslan F, Vince GH, et al. Magnesium treatment for neuroprotection in ischemic diseases of the brain. Exp Transl Stroke Med. (2013) 5:6. doi: 10.1186/2040-7378-5-6
106. McDonald JW, Silverstein FS, Johnston MV. Magnesium reduces N-Methyl-D-aspartate (Nmda)-mediated brain injury in perinatal rats. Neurosci Lett. (1990) 109:234–8. doi: 10.1016/0304-3940(90)90569-U
107. Chi OZ, Pollak P, Weiss HR. Effects of magnesium sulfate and nifedipine on regional cerebral blood flow during middle cerebral artery ligation in the rat. Arch Int Pharmacodyn Ther. (1990) 304:196–205.
108. Schanne FA, Gupta RK, Stanton PK. 31p-Nmr study of transient ischemia in rat hippocampal slices in vitro. Biochim Biophys Acta. (1993) 1158:257–63. doi: 10.1016/0304-4165(93)90023-2
109. Afshari D, Moradian N, Rezaei M. Evaluation of the intravenous magnesium sulfate effect in clinical improvement of patients with acute ischemic stroke. Clin Neurol Neurosurg. (2013) 115:400–4. doi: 10.1016/j.clineuro.2012.06.001
110. Muir KW, Lees KR, Ford I, Davis S. Magnesium for acute stroke (intravenous magnesium efficacy in stroke trial): randomised controlled trial. Lancet. (2004) 363:439–45. doi: 10.1016/S0140-6736(04)15490-1
111. Mousavi S, J Z, Saadatnia M. Magnesium sulfate in acute stroke: a randomized double-blind clinical trial. J Res Med Sci. (2004) 9:7–10.
112. Saver JL, Starkman S, Eckstein M, Stratton SJ, Pratt FD, Hamilton S, et al. Prehospital use of magnesium sulfate as neuroprotection in acute stroke. N Engl J Med. (2015) 372:528–36. doi: 10.1056/NEJMoa1408827
113. Lipton SA, Rosenberg PA. Excitatory amino acids as a final common pathway for neurologic disorders. N Engl J Med. (1994) 330:613–22. doi: 10.1056/NEJM199403033300907
114. Carletti R, Ratti E, Gaviraghi G, Bowery NG. Comparative receptor autoradiography of ex vivo and in vitro [3h]Dizocilpine binding in mouse brain after middle cerebral artery occlusion. Neuropharmacology. (1994) 33:43–53. doi: 10.1016/0028-3908(94)90095-7
115. Muir KW. Glutamate-based therapeutic approaches: clinical trials with nmda antagonists. Curr Opin Pharmacol. (2006) 6:53–60. doi: 10.1016/j.coph.2005.12.002
116. Tymianski M. Emerging mechanisms of disrupted cellular signaling in brain ischemia. Nat Neurosci. (2011) 14:1369–73. doi: 10.1038/nn.2951
117. Hill MD, Martin RH, Mikulis D, Wong JH, Silver FL, Terbrugge KG, et al. Safety and efficacy of na-1 in patients with iatrogenic stroke after endovascular aneurysm repair (enact): a phase 2, randomised, double-blind, placebo-controlled trial. Lancet Neurol. (2012) 11:942–50. doi: 10.1016/S1474-4422(12)70225-9
118. Cook DJ, Teves L, Tymianski M. Treatment of stroke with a Psd-95 Inhibitor in the gyrencephalic primate brain. Nature. (2012) 483:213–7. doi: 10.1038/nature10841
119. Biegon A, Fry PA, Paden CM, Alexandrovich A, Tsenter J, Shohami E. Dynamic changes in N-Methyl-D-aspartate receptors after closed head injury in mice: implications for treatment of neurological and cognitive deficits. Proc Natl Acad Sci U S A. (2004) 101:5117–22. doi: 10.1073/pnas.0305741101
120. Dhawan J, Benveniste H, Luo Z, Nawrocky M, Smith SD, Biegon A, et al. New look at glutamate and ischemia: nmda agonist improves long-term functional outcome in a rat model of stroke. Future Neurol. (2011) 6:823–34. doi: 10.2217/fnl.11.55
121. Baigent C, Blackwell L, Emberson J, Holland LE, Reith C, Bhala N, et al. Efficacy and safety of more intensive lowering of Ldl cholesterol: a meta-analysis of data from 170,000 participants in 26 randomised trials. Lancet. (2010) 376:1670–81. doi: 10.1016/S0140-6736(10)61350-5
122. Naci H, Brugts J, Ades T. Comparative tolerability and harms of individual statins: a study-level network meta-analysis of 246 955 participants from 135 randomized, controlled trials. Circ Cardiovasc Qual Outcomes. (2013) 6:390–9. doi: 10.1161/CIRCOUTCOMES.111.000071
123. NC D, Callaly EL, Duggan J, Merwick Á, Hannon N, Sheehan Ó, et al. Association between acute statin therapy, survival, and improved functional outcome after ischemic stroke: the north dublin population stroke study. Stroke. (2011) 42:1021–9. doi: 10.1161/STROKEAHA.110.596734
124. Flint AC, Kamel H, Navi BB, Rao VA, Faigeles BS, Conell C, et al. Statin use during ischemic stroke hospitalization is strongly associated with improved poststroke survival. Stroke. (2012) 43:147–54. doi: 10.1161/STROKEAHA.111.627729
125. Elkind MS, Sacco RL, Macarthur RB, Peerschke E, Neils G, Andrews H, et al. High-dose lovastatin for acute ischemic stroke: results of the phase i dose escalation neuroprotection with statin therapy for acute recovery trial (Neustart). Cerebrovasc Dis. (2009) 28:266–75. doi: 10.1159/000228709
126. Yoshimura S, Uchida K, Daimon T, Takashima R, Kimura K, Morimoto T. Randomized controlled trial of early versus delayed statin therapy in patients with acute ischemic stroke: assort trial (administration of statin on acute ischemic stroke patient). Stroke. (2017) 48:3057–63. doi: 10.1161/STROKEAHA.117.017623
127. Simard JM, Chen M, Tarasov KV, Bhatta S, Ivanova S, Melnitchenko L, et al. Newly expressed sur1-regulated Nc(Ca-Atp) channel mediates cerebral edema after ischemic stroke. Nat Med. (2006) 12:433–40. doi: 10.1038/nm1390
128. Khanna A, Walcott BP, Kahle KT, Simard JM. Effect of glibenclamide on the prevention of secondary brain injury following ischemic stroke in humans. Neurosurg Focus. (2014) 36:E11. doi: 10.3171/2013.10.FOCUS13404
129. Mehta RI, Tosun C, Ivanova S, Tsymbalyuk N, Famakin BM, Kwon MS, et al. Sur1-Trpm4 cation channel expression in human cerebral infarcts. J Neuropathol Exp Neurol. (2015) 74:835–49. doi: 10.1097/NEN.0000000000000223
130. Simard JM, Tsymbalyuk N, Tsymbalyuk O, Ivanova S, Yurovsky V, Gerzanich V. Glibenclamide is superior to decompressive craniectomy in a rat model of malignant stroke. Stroke. (2010) 41:531–7. doi: 10.1161/STROKEAHA.109.572644
131. Sheth KN, Elm JJ, Beslow LA, Sze GK, Kimberly WT. Glyburide advantage in malignant edema and stroke (Games-Rp) trial: rationale and design. Neurocrit Care. (2016) 24:132–9. doi: 10.1007/s12028-015-0189-7
132. Sheth KN, Elm JJ, Molyneaux BJ, Hinson H, Beslow LA, Sze GK, et al. Safety and efficacy of intravenous glyburide on brain swelling after large hemispheric infarction (Games-Rp): a randomised, double-blind, placebo-controlled phase 2 trial. Lancet Neurol. (2016) 15:1160–9. doi: 10.1016/S1474-4422(16)30196-X
133. Baird TA, Parsons MW, Phan T, Butcher KS, Desmond PM, Tress BM, et al. Persistent poststroke hyperglycemia is independently associated with infarct expansion and worse clinical outcome. Stroke. (2003) 34:2208–14. doi: 10.1161/01.STR.0000085087.41330.FF
134. Els T, Klisch J, Orszagh M, Hetzel A, Schulte-Mönting J, Schumacher M, et al. Hyperglycemia in patients with focal cerebral ischemia after intravenous thrombolysis: influence on clinical outcome and infarct size. Cerebrovasc Dis. (2002) 13:89–94. doi: 10.1159/000047756
135. Yong M, Kaste M. Dynamic of hyperglycemia as a predictor of stroke outcome in the ecass-Ii trial. Stroke. (2008) 39:2749–55. doi: 10.1161/STROKEAHA.108.514307
136. Bruno A, Levine SR, Frankel MR, Brott TG, Lin Y, Tilley BC, et al. Admission glucose level and clinical outcomes in the ninds Rt-Pa stroke trial. Neurology. (2002) 59:669–74. doi: 10.1212/WNL.59.5.669
137. Mi D, Wang P, Yang B, Pu Y, Yang Z, Liu L. Correlation of hyperglycemia with mortality after acute ischemic stroke. Ther Adv Neurol Disord. (2018) 11:1756285617731686. doi: 10.1177/1756285617731686
138. Duckrow RB, Beard DC, Brennan RW. Regional cerebral blood flow decreases during hyperglycemia. Ann Neurol. (1985) 17:267–72. doi: 10.1002/ana.410170308
139. Li PA, Shuaib A, Miyashita H, He QP, Siesjö BK, Warner DS. Hyperglycemia enhances extracellular glutamate accumulation in rats subjected to forebrain ischemia. Stroke. (2000) 31:183–92. doi: 10.1161/01.STR.31.1.183
140. Mohanty P, Hamouda W, Garg R, Aljada A, Ghanim H, Dandona P. Glucose challenge stimulates reactive oxygen species (Ros) generation by leucocytes. J Clin Endocrinol Metab. (2000) 85:2970–3. doi: 10.1210/jcem.85.8.6854
141. Garg R, Chaudhuri A, Munschauer F, Dandona P. Hyperglycemia, insulin, and acute ischemic stroke: a mechanistic justification for a trial of insulin infusion therapy. Stroke. (2006) 37:267–73. doi: 10.1161/01.STR.0000195175.29487.30
142. Yu LY, Pei Y. Insulin neuroprotection and the mechanisms. Chin Med J. (2015) 128:976–81. doi: 10.4103/0366-6999.154323
143. Bruno A, Durkalski VL, Hall CE, Juneja R, Barsan WG, Janis S, et al. The Stroke hyperglycemia insulin network effort (shine) trial protocol: a randomized, blinded, efficacy trial of standard vs. intensive hyperglycemia management in acute stroke. Int J Stroke. (2014) 9:246–51. doi: 10.1111/ijs.12045
144. Johnston KC, Bruno A, Pauls Q, Hall CE, Barrett KM, Barsan W, et al. Intensive Vs standard treatment of hyperglycemia and functional outcome in patients with acute ischemic stroke: the shine randomized clinical trial. JAMA. (2019) 322:326–35. doi: 10.1001/jama.2019.9346
145. Okada H, Matsuda Y, Chung J, Webster Crowley R, Lopes DK. Editorial: perspective in current neuroprotection strategies. Neurosurg Focus. (2017) 42:E5. doi: 10.3171/2017.1.FOCUS1728
146. Hong JM, Choi MH, Sohn SI, Hwang YH, Ahn SH, Lee YB, et al. Safety and optimal neuroprotection of neu2000 in acute ischemic stroke with recanalization: study protocol for a randomized, double-blinded, placebo-controlled, phase-Ii trial. Trials. (2018) 19:375. doi: 10.1186/s13063-018-2746-9
147. Pharma GNT. A Phase Ii, Double-Blind, Randomized, Placebo-Controlled, Multi-Center Study to Assess Efficacy and Safety of Neu2000kwl in Patients with Acute Ischemic Stroke Receiving Endovascular Therapy. Clinical trial registration. clinicaltrials.gov. (2017) 2017/07/11/. Report No.: NCT02831088.
148. Zhang J, Liu J, Li D, Zhang C, Liu M. Calcium antagonists for acute ischemic stroke. Cochrane Database Syst Rev. (2019) 2:Cd001928. doi: 10.1002/14651858.CD001928.pub3
149. Infeld B, Davis SM, Donnan GA, Yasaka M, Lichtenstein M, Mitchell PJ, et al. Nimodipine and perfusion changes after stroke. Stroke. (1999) 30:1417–23. doi: 10.1161/01.STR.30.7.1417
150. Sabbatini M, Tomassoni D, Amenta F. Hypertensive brain damage: comparative evaluation of protective effect of treatment with dihydropyridine derivatives in spontaneously hypertensive rats. Mech Ageing Dev. (2001) 122:2085–105. doi: 10.1016/S0047-6374(01)00318-9
151. Deyo RA, Straube KT, Disterhoft JF. Nimodipine facilitates associative learning in aging rabbits. Science. (1989) 243:809–11. doi: 10.1126/science.2916127
152. Nomura M. Effect of nimodipine on brightness discrimination learning test in wistar kyoto and spontaneously hypertensive rats. Arzneimittelforschung. (1988) 38:1282–6.
153. Scriabine A, Schuurman T, Traber J. Pharmacological basis for the use of nimodipine in central nervous system disorders. FASEB J. (1989) 3:1799–806. doi: 10.1096/fasebj.3.7.2565839
154. Levy A, Kong RM, Stillman MJ, Shukitt-Hale B, Kadar T, Rauch TM, et al. Nimodipine improves spatial working memory and elevates hippocampal acetylcholine in young rats. Pharmacol Biochem Behav. (1991) 39:781–6. doi: 10.1016/0091-3057(91)90164-W
155. Meneses A, Terrón JA, Ibarra M, Hong E. Effects of nimodipine on learning in normotensive and spontaneously hypertensive rats. Behav Brain Res. (1997) 85:121–5. doi: 10.1016/S0166-4328(97)87580-8
156. Tomassoni D, Lanari A, Silvestrelli G, Traini E, Amenta F. Nimodipine and its use in cerebrovascular disease: evidence from recent preclinical and controlled clinical studies. Clin Experiment Hypertension. (2008) 30(8):744–66. doi: 10.1080/10641960802580232
157. Pickard J, Murray G, Illingworth R, Shaw M, Teasdale G, Foy P, et al. Effect of oral nimodipine on cerebral infarction and outcome after subarachnoid haemorrhage: british aneurysm nimodipine trial. Br Med J. (1989) 298:636–42. doi: 10.1136/bmj.298.6674.636
158. Philippon J, Grob R, Dagreou F, Guggiari M, Rivierez M, Viars P. Prevention of vasospasm in subarachnoid haemorrhage. a controlled study with nimodipine. Acta Neurochir. (1986) 82:110–4. doi: 10.1007/BF01456369
159. Gilsbach JM. Nimodipine in the prevention of ischaemic deficits after aneurysmal subarachnoid haemorrhage. Prev Treatment Delayed Ischaemic Dysfunction Patient Subarachnoid Haemorrhage. (1988):41–50. doi: 10.1007/978-3-7091-9014-2_7
160. Allen GS, Ahn HS, Preziosi TJ, Battye R, Boone SC, Chou SN, et al. Cerebral arterial spasm–a controlled trial of nimodipine in patients with subarachnoid hemorrhage. N Engl J Med. (1983) 308:619–24. doi: 10.1056/NEJM198303173081103
161. Lalkovičová M, Danielisová V. Neuroprotection and antioxidants. Neural Reg Res. (2016) 11:865–74. doi: 10.4103/1673-5374.184447
162. Ginsberg MD, Palesch YY, Hill MD, Martin RH, Moy CS, Barsan WG, et al. High-dose albumin treatment for acute ischaemic stroke (alias) part 2: a randomised, double-blind, phase 3, placebo-controlled trial. Lancet Neurol. (2013) 12:1049–58. doi: 10.1016/S1474-4422(13)70223-0
163. Bang OY. Advances in biomarker for stroke patients: from marker to regulator. Prec Future Med. (2017) 1:32–42. doi: 10.23838/pfm.2017.00052
164. Chamorro Á, Amaro S, Castellanos M, Segura T, Arenillas J, Martí-Fábregas J, et al. Safety and efficacy of uric acid in patients with acute stroke (urico-ictus): a randomised, double-blind phase 2b/3 trial. Lancet Neurol. (2014) 13:453–60. doi: 10.1016/S1474-4422(14)70054-7
165. Love S. Oxidative stress in brain ischemia. Brain Pathol. (1999) 9:119–31. doi: 10.1111/j.1750-3639.1999.tb00214.x
166. Chamorro Á, Dirnagl U, Urra X, Planas AM. Neuroprotection in acute stroke: targeting excitotoxicity, oxidative and nitrosative stress, and inflammation. Lancet Neurol. (2016) 15:869–81. doi: 10.1016/S1474-4422(16)00114-9
167. Matsumoto S, Murozono M, Kanazawa M, Nara T, Ozawa T, Watanabe Y. Edaravone and cyclosporine a as neuroprotective agents for acute ischemic stroke. Acute Med Surg. (2018) 5:213–21. doi: 10.1002/ams2.343
168. Ishihara H, Suzuki M. Japanese Guidelines for the Management of Stroke 2015: Overview of the Chapter on Subarachnoid Hemorrhage. Nihon Rinsho. (2016) 74:677–80.
169. Feng S, Yang Q, Liu M, Li W, Yuan W, Zhang S, et al. Edaravone for acute ischaemic stroke. Cochrane Database Systemat Rev. (2011) (12):Cd007230. doi: 10.1002/14651858.CD007230.pub2
170. Enomoto M, Endo A, Yatsushige H, Fushimi K, Otomo Y. Clinical effects of early edaravone use in acute ischemic stroke patients treated by endovascular reperfusion therapy. Stroke. (2019) 50:652–8. doi: 10.1161/STROKEAHA.118.023815
171. Forsse A, Nielsen TH, Nygaard KH, Nordström CH, Gramsbergen JB, Poulsen FR. Cyclosporin a ameliorates cerebral oxidative metabolism and infarct size in the endothelin-1 rat model of transient cerebral ischaemia. Sci Rep. (2019) 9:3702. doi: 10.1038/s41598-019-40245-x
172. Nighoghossian N, Ovize M, Mewton N, Ong E, Cho TH. Cyclosporine a, a potential therapy of ischemic reperfusion injury. a common history for heart and brain. Cerebrovasc Dis. (2016) 42:309–18. doi: 10.1159/000446850
173. Nighoghossian N, Berthezène Y, Mechtouff L, Derex L, Cho TH, Ritzenthaler T, et al. Cyclosporine in acute ischemic stroke. Neurology. (2015) 84:2216–23. doi: 10.1212/WNL.0000000000001639
174. Stoll G, Jander S, Schroeter M. Inflammation and glial responses in ischemic brain lesions. Prog Neurobiol. (1998) 56:149–71.
175. Llovera G, Hofmann K, Roth S, Salas-Pérdomo A, Ferrer-Ferrer M, Perego C, et al. Results of a preclinical randomized controlled multicenter trial (prct): anti-Cd49d treatment for acute brain ischemia. Science Transl Med. (2015) 7:299ra121. doi: 10.1126/scitranslmed.aaa9853
176. Langhauser F, Kraft P, Göb E, Leinweber J, Schuhmann MK, Lorenz K, et al. Blocking of α4 integrin does not protect from acute ischemic stroke in mice. Stroke. (2014) 45:1799–806. doi: 10.1161/STROKEAHA.114.005000
177. Becker K, Kindrick D, Relton J, Harlan J, Winn R. Antibody to the Alpha4 integrin decreases infarct size in transient focal cerebral ischemia in rats. Stroke. (2001) 32:206–11. doi: 10.1161/01.STR.32.1.206
178. Elkins J, Veltkamp R, Montaner J, Johnston SC, Singhal AB, Becker K, et al. Safety and efficacy of natalizumab in patients with acute ischaemic stroke (action): a randomised, placebo-controlled, double-blind phase 2 trial. Lancet Neurol. (2017) 16:217–26. doi: 10.1016/S1474-4422(16)30357-X
179. Globus MY, Busto R, Martinez E, Valdés I, Dietrich WD, Ginsberg MD. Comparative effect of transient global ischemia on extracellular levels of glutamate, glycine, and gamma-aminobutyric acid in vulnerable and nonvulnerable brain regions in the rat. J Neurochem. (1991) 57:470–8. doi: 10.1111/j.1471-4159.1991.tb03775.x
180. Phillis JW, O'Regan MH. Characterization of modes of release of amino acids in the ischemic/reperfused rat cerebral cortex. Neurochem Int. (2003) 43:461–7. doi: 10.1016/S0197-0186(03)00035-4
181. Schwartz-Bloom RD, McDonough KJ, Chase PJ, Chadwick LE, Inglefield JR, Levin ED. Long-term neuroprotection by benzodiazepine: full versus partial agonists after transient cerebral ischemia in the gerbil. J Cerebr Blood Flow Metab. (1998) 18:548–58. doi: 10.1097/00004647-199805000-00010
182. Mele M, Ribeiro L, Inácio AR, Wieloch T, Duarte CB. Gabaa receptor dephosphorylation followed by internalization is coupled to neuronal death in in vitro ischemia. Neurobiol Dis. (2014) 65:220–32. doi: 10.1016/j.nbd.2014.01.019
183. Sommer C, Fahrner A, Kiessling M. Postischemic neuroprotection in the ischemia-tolerant state gerbil hippocampus is associated with increased ligand binding to inhibitory gaba a receptors. Acta Neuropathol. (2003) 105:197–202. doi: 10.1007/s00401-002-0632-7
184. DeFazio RA, Raval AP, Lin HW, Dave KR, Della-Morte D, Perez-Pinzon MA. Gaba synapses mediate neuroprotection after ischemic and εpkc preconditioning in rat hippocampal slice cultures. J Cerebr Blood Flow Metab. (2009) 29:375–84. doi: 10.1038/jcbfm.2008.126
185. Costa C, Leone G, Saulle E, Pisani F, Bernardi G, Calabresi P. Coactivation of Gabaa and gabab receptor results in neuroprotection during in vitro ischemia. Stroke. (2004) 35:596–600. doi: 10.1161/01.STR.0000113691.32026.06
186. Schwartz-Bloom RD, Sah R. Gamma-aminobutyric acid(a) neurotransmission and cerebral ischemia. J Neurochem. (2001) 77:353–71. doi: 10.1046/j.1471-4159.2001.00274.x
187. Huang L, Li Q, Wen R, Yu Z, Li N, Ma L, et al. Rho-kinase inhibitor prevents acute injury against transient focal cerebral ischemia by enhancing the expression and function of gaba receptors in rats. Eur J Pharmacol. (2017) 797:134–42. doi: 10.1016/j.ejphar.2017.01.021
188. del Zoppo GJ, Milner R, Mabuchi T, Hung S, Wang X, Berg GI, et al. Microglial activation and matrix protease generation during focal cerebral ischemia. Stroke. (2007) 38:646–51. doi: 10.1161/01.STR.0000254477.34231.cb
189. O'Bryant Z, Vann KT, Xiong ZG. Translational strategies for neuroprotection in ischemic stroke–focusing on acid-sensing ion channel 1a. Transl Stroke Res. (2014) 5:59–68. doi: 10.1007/s12975-013-0319-5
190. Mergenthaler P, Dirnagl U, Meisel A. Pathophysiology of stroke: lessons from animal models. Metab Brain Dis. (2004) 19:151–67. doi: 10.1023/B:MEBR.0000043966.46964.e6
191. Bornstein NM, Saver JL, Diener HC, Gorelick PB, Shuaib A, Solberg Y, et al. An Injectable implant to stimulate the sphenopalatine ganglion for treatment of acute ischaemic stroke up to 24 h from onset (impact-24b): an international, randomised, double-blind, sham-controlled, pivotal trial. Lancet. (2019) 394:219–29. doi: 10.1016/S0140-6736(19)31192-4
192. Levi H, Schoknecht K, Prager O, Chassidim Y, Weissberg I, Serlin Y, et al. Stimulation of the sphenopalatine ganglion induces reperfusion and blood-brain barrier protection in the photothrombotic stroke model. PLoS ONE. (2012) 7:e39636. doi: 10.1371/journal.pone.0039636
193. Gelderblom M, Leypoldt F, Steinbach K, Behrens D, Choe C-U, Siler DA, et al. Temporal and spatial dynamics of cerebral immune cell accumulation in stroke. Stroke. (2009) 40:1849–57. doi: 10.1161/STROKEAHA.108.534503
195. Hudome S, Palmer C, Roberts RL, Mauger D, Housman C, Towfighi J. The role of neutrophils in the production of hypoxic-ischemic brain injury in the neonatal rat. Pediatr Res. (1997) 41:607–16. doi: 10.1203/00006450-199705000-00002
196. Matsuo Y, Onodera H, Shiga Y, Nakamura M, Ninomiya M, Kihara T, et al. Correlation between myeloperoxidase-quantified neutrophil accumulation and ischemic brain injury in the rat. Effects Neutrophil Depletion Stroke. (1994) 25:1469–75. doi: 10.1161/01.STR.25.7.1469
197. Napoli E, Borlongan CV. Recent advances in stem cell-based therapeutics for stroke. Transl Stroke Res. (2016) 7:452–7. doi: 10.1007/s12975-016-0490-6
198. Gu N, Rao C, Tian Y, Di Z, Liu Z, Chang M, et al. Anti-inflammatory and antiapoptotic effects of mesenchymal stem cells transplantation in rat brain with cerebral ischemia. J Stroke Cerebrovasc Dis. (2014) 23:2598–606. doi: 10.1016/j.jstrokecerebrovasdis.2014.05.032
199. Wei L, Fraser JL, Lu ZY, Hu X, Yu SP. Transplantation of hypoxia preconditioned bone marrow mesenchymal stem cells enhances angiogenesis and neurogenesis after cerebral ischemia in rats. Neurobiol Dis. (2012) 46:635–45. doi: 10.1016/j.nbd.2012.03.002
200. Banerjee S, Williamson DA, Habib N, Chataway J. The potential benefit of stem cell therapy after stroke: an update. Vasc Health Risk Manag. (2012) 8:569–80. doi: 10.2147/VHRM.S25745
201. Chen L, Qiu R, Li L, He D, Lv H, Wu X, et al. The role of exogenous neural stem cells transplantation in cerebral ischemic stroke. J Biomed Nanotechnol. (2014) 10:3219–30. doi: 10.1166/jbn.2014.2018
202. Hao L, Zou Z, Tian H, Zhang Y, Zhou H, Liu L. Stem cell-based therapies for ischemic stroke. Biomed Res Int. (2014) 2014:468748. doi: 10.1155/2014/468748
203. Bang OY. Clinical trials of adult stem cell therapy in patients with ischemic stroke. J Clin Neurol. (2016) 12:14–20. doi: 10.3988/jcn.2016.12.1.14
204. Wang Q, Duan F, Wang MX, Wang XD, Liu P, Ma LZ. Effect of stem cell-based therapy for ischemic stroke treatment: a meta-analysis. Clin Neurol Neurosurg. (2016) 146:1–11. doi: 10.1016/j.clineuro.2016.04.011
205. Savitz SI, Baron JC, Fisher M. Stroke treatment academic industry roundtable x: brain cytoprotection therapies in the reperfusion era. Stroke. (2019) 50:1026–31. doi: 10.1161/STROKEAHA.118.023927
Keywords: acute ischemic stroke, neuroprotection, ischemia, therapy, management
Citation: Ghozy S, Reda A, Varney J, Elhawary AS, Shah J, Murry K, Sobeeh MG, Nayak SS, Azzam AY, Brinjikji W, Kadirvel R and Kallmes DF (2022) Neuroprotection in Acute Ischemic Stroke: A Battle Against the Biology of Nature. Front. Neurol. 13:870141. doi: 10.3389/fneur.2022.870141
Received: 06 February 2022; Accepted: 21 April 2022;
Published: 31 May 2022.
Edited by:
Eduardo Candelario-Jalil, University of Florida, United StatesReviewed by:
Ziping Han, Capital Medical University, ChinaDi Wu, Capital Medical University, China
Ido Van Den Wijngaard, Haaglanden Medical Center, Netherlands
Copyright © 2022 Ghozy, Reda, Varney, Elhawary, Shah, Murry, Sobeeh, Nayak, Azzam, Brinjikji, Kadirvel and Kallmes. This is an open-access article distributed under the terms of the Creative Commons Attribution License (CC BY). The use, distribution or reproduction in other forums is permitted, provided the original author(s) and the copyright owner(s) are credited and that the original publication in this journal is cited, in accordance with accepted academic practice. No use, distribution or reproduction is permitted which does not comply with these terms.
*Correspondence: Jaffer Shah, jaffer.shah@kateb.edu.af
†ORCID: Sherief Ghozy orcid.org/0000-0001-5629-3023
Abdullah Reda orcid.org/0000-0002-2180-0029
Joseph Varney orcid.org/0000-0003-1797-8016
Kimberly Murry orcid.org/0000-0001-8522-4086
Mohammed Gomaa Sobeeh orcid.org/0000-0001-7201-8793
‡These authors have contributed equally to this work