- 1Department of Neurosurgery, The Affiliated Hospital of Southwest Medical University, Luzhou, China
- 2Laboratory of Neurological Diseases and Brain Function, The Affiliated Hospital of Southwest Medical University, Luzhou, China
- 3Institute of Epigenetics and Brain Science, Southwest Medical University, Luzhou, China
- 4Academician (Expert) Workstation of Sichuan Province, The Affiliated Hospital of Southwest Medical University, Luzhou, China
- 5Sichuan Clinical Research Center for Neurosurgery, The Affiliated Hospital of Southwest Medical University, Luzhou, China
A wide plethora of intervention procedures, tissue plasminogen activators, mechanical thrombectomy, and several neuroprotective drugs were reported in stroke research over the last decennium. However, against this vivid background of newly emerging pieces of evidence, there is little to no advancement in the overall functional outcomes. With the advancement of epigenetic tools and technologies associated with intervention medicine, stroke research has entered a new fertile. The stroke involves an overabundance of inflammatory responses arising in part due to the body’s immune response to brain injury. Neuroinflammation contributes to significant neuronal cell death and the development of functional impairment and even death in stroke patients. Recent studies have demonstrated that epigenetics plays a key role in post-stroke conditions, leading to inflammatory responses and alteration of the microenvironment within the injured tissue. In this review, we summarize the progress of epigenetics which provides an overview of recent advancements on the emerging key role of secondary brain injury in stroke. We also discuss potential epigenetic therapies related to clinical practice.
Introduction
Stroke is one of the main leading causes of death and the first leading cause of disability worldwide (Avan et al., 2019; Collaborators, 2019). Hemorrhagic stroke, including intracerebral hemorrhage (ICH) and subarachnoid hemorrhage (SAH), happens when a blood vessel in the brain bursts or when brain tissue starts to bleed. On the other hand, ischemic stroke (IS) directly results from the disruption of blood supply to the brain and constitutes approximately 85% of all known cases of stroke. After the stroke, injured brain parenchyma initiates biochemical cascades, which include energy failure, ionic pump failure, oxidative damage, cell death, and inflammation, eventually leading to irreversible brain damage (Iglesias-Rey et al., 2022). Additionally, patients surviving stroke may suffer from functional disabilities that might require temporary or lifelong assistance (Aslanyan et al., 2003). Thus, understanding stroke at the molecular level will help researchers to produce key therapeutic strategies to minimize secondary injuries and promotion of neuroprotection associated with stroke (Saini et al., 2021).
Over the past few decades, researchers have advanced in our understanding of the epigenetic mechanisms involved in the central nervous system (CNS) and its role in neuropsychiatric disorders (Szyf, 2015). These epigenetic-related findings also offer the important translational potential for stroke research. Thus, fully understanding the role of epigenetic regulators in the stroke process is crucial to harness the potential of epigenetic therapies. Here, we review three epigenetic mechanisms involved in secondary brain injuries post-stoke: histone modification, DNA-methylation, and RNA modifications. We also discuss the relevant clinical treatment targeting epigenetics and summarize future advancements in this field.
Etiology
The mechanism and pathophysiology involved in ischemic stroke and hemorrhagic stroke are quite different but with some overlap. Two major mechanisms responsible for acute ischemic stroke (AIS) are thromboembolism and hemodynamic failure. Embolism, more precisely cardio-embolism, has been demonstrated to produce 20 to 30% of all ischemic strokes (Kolominsky-Rabas et al., 2001; Kamel and Healey, 2017). Risk factors associated with cardio-embolism include atrial fibrillation (Kamel et al., 2016), systolic heart failure (Go et al., 2001), acute myocardial infarction (Putaala and Nieminen, 2018), patent foramen ovale (Gottdiener et al., 1983), aortic arch atheroma (Witt et al., 2006), prosthetic heart valves (Cannegieter et al., 1994) and infective endocarditis (Kim and Kim, 2018). Large vessel atherosclerosis (LVA) is another main contributor to ischemic stroke. LVA accounts for nearly 15 to 20% of all ischemic strokes. In addition, small vessel occlusion is also a culprit that can be diagnosed in approximately 25% of patients with ischemic stroke (Grau et al., 2001). Hemorrhagic stroke, on the other hand, has a well-established relationship with traumatic brain injury (TBI) (Chen et al., 2011), cerebral aneurysm (Nieuwkamp et al., 2009), anti-thrombolytic therapy (Puy et al., 2022), hypertension (Wan et al., 2022), and other cerebrovascular diseases.
Pathophysiology
Under injured conditions, several molecules can gain access to the cytoplasm of the cell and leak from the dying cells into the extracellular environment. These spilled substances such as DNA are not only manifested as changes in expression but also in their own structures, these changes will gather a series of complex secondary pathophysiological processes (Eser Ocak et al., 2020; Gamdzyk et al., 2020). The pathophysiology involving stroke is quite complex and involves various cascade processes, which include: loss of cellular homeostasis, energy failure, metabolic acidosis, increased intracellular Ca2+ levels, free-radical mediated toxicity, generation of arachidonic acid products, cytokine-mediated cytotoxicity, complement activation, apoptosis, autophagy, disruption of the blood-brain barrier (BBB), activation of glial cells and infiltration of leukocytes (Lu et al., 2021; Qiu et al., 2021; Ye et al., 2021; Peng et al., 2022). The mechanism involved in both ischemic and hemorrhagic stroke produces significant cerebral hypoperfusion leading to an increase in anaerobic metabolism and eventually, lactic acidosis which in turn sequentially causes astrocyte demise and an increase in neuroinflammatory cytokines.
Neuroinflammation has been recognized as one of the main culprits in promoting further insults in post-stroke conditions, however, they also play a beneficial role in functional recovery (Bourhy et al., 2022). Similarly, a decrease in cerebral hypo-perfusion can also produce malfunction of the ionic pump causing potassium ions (K+) efflux and sodium, calcium (Na+ and Ca2+, respectively) influx into the neuronal cells and adenosine triphosphate (ATP) depletion causing excitotoxicity, edema, and eventually led to necrosis (Zhang et al., 2021; Zhong et al., 2022). Red blood cell lysis can further cause oxidative damage which in turn further supports necrosis (Figure 1).
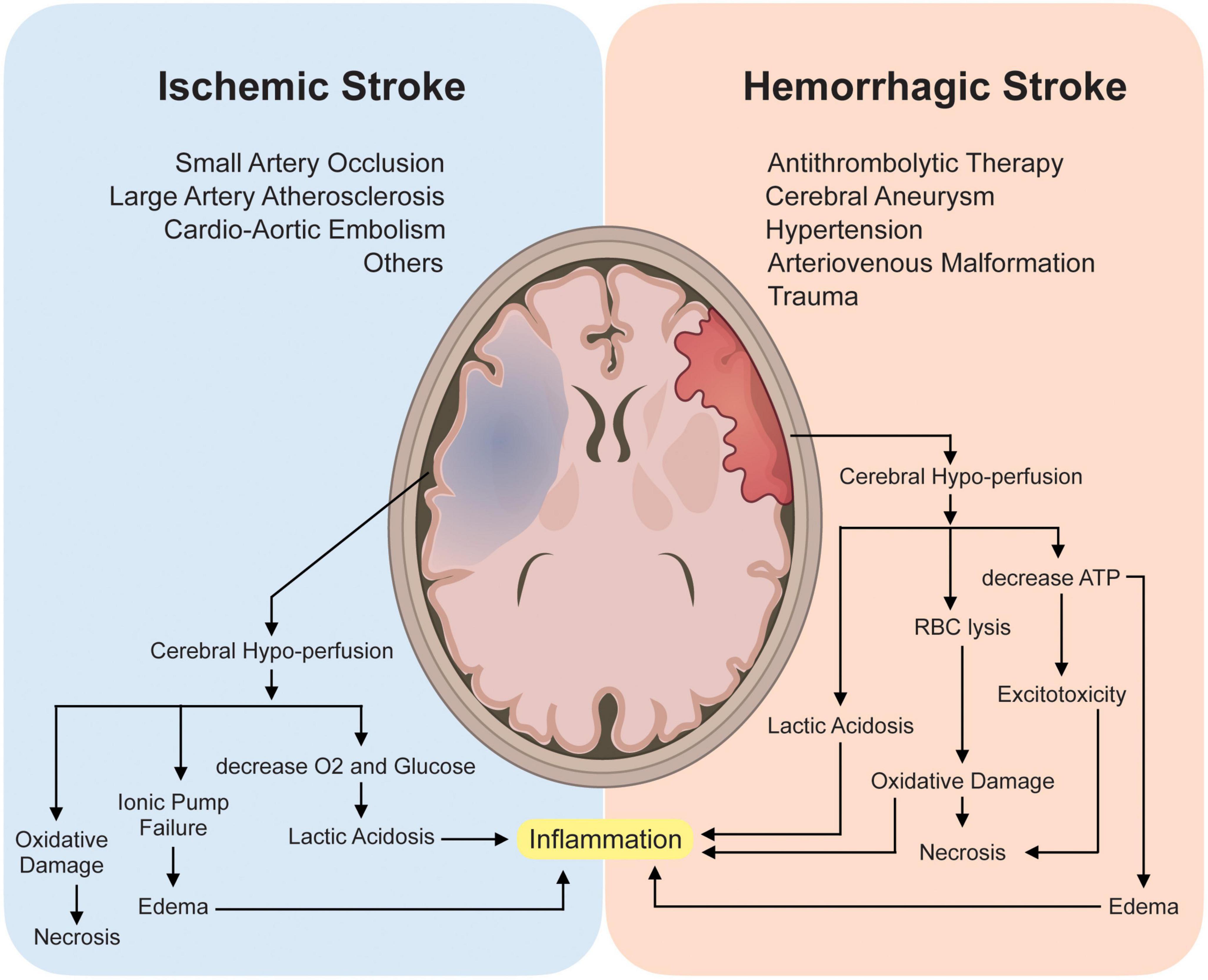
Figure 1. Pathophysiology and Mechanism involved in Ischemic and Hemorrhagic stroke. Briefly, mechanisms involved in both ischemic and hemorrhagic stroke involve cerebral hypo-perfusion leading to oxygen (O2) deprivation causing an increase in anaerobic metabolism and eventually lactic acidosis which sequentially causes astrocyte demise and an increase in neuroinflammatory cytokines thus promoting neuroinflammation. Subsequently, cerebral hypo-perfusion can also cause malfunction of the ionic pump causing potassium ions (K+) efflux, sodium and calcium (Na+ and Ca2+ respectively) influx into the neuronal cells and adenosine triphosphate (ATP) depletion causing excitotoxicity, edema, and eventually led to necrosis. Red blood cell lysis can further cause oxidative damage which further supports necrosis.
Epigenetics in research frontline
Epigenetics is defined as the branch of biology which studies the causal interactions between genes and their products which bring the phenotype into being. Epigenetic variation, a phenomenon that alters genome modifications without affecting DNA sequence, can affect the development of individuals (Freedman et al., 2022), cancer evolution (Nam et al., 2021), neurodegenerative disease (Corces et al., 2020), and mental disorder (Havdahl et al., 2021). In particular, dynamic epigenetic states regulate immune response and inflammation under pathological conditions (Liotti et al., 2022). Recent epigenetic studies have been demonstrated to play a key role in post-stroke conditions leading to inflammatory responses and alteration of the microenvironment within the injured tissue (Zhao et al., 2016). The current understanding and development of epigenetic tools have given the researchers a more reliable method of competitive differentiation of normal versus diseased conditions at the molecular level (Ng et al., 2018). Contemporary studies in the field of epigenetics involve Histone modification, DNA-methylation, and RNA modifications, and their association with both pre and post-stroke conditions (Figure 2).
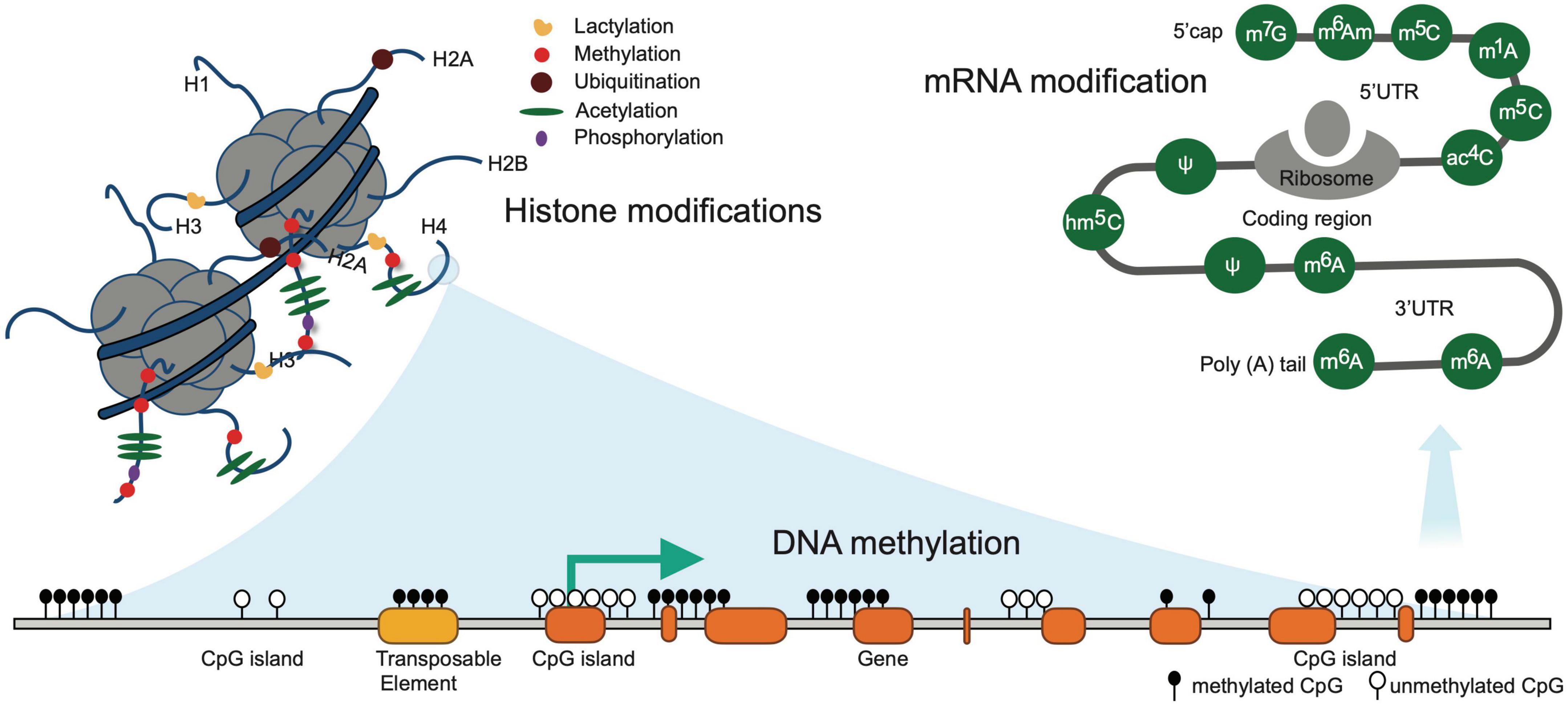
Figure 2. Illustrates common epigenetic modifications in stroke. DNA methylation occurring exclusively at the CpG island is associated with gene silencing and is irreversible modifications. Known histone modifications occurring at the amino-terminal tails are short-term reversible modifications. RNA modifications are the chemical alteration of the RNA molecules post transcription that alters the expression of RNA.
Histone modification
Histone is the basic protein found in the nucleus of eukaryotic cells wrapped around by 146 base pairs (bp) of DNA into a compact structure known as a nucleosome. The interaction between histone and DNA is determined by the electrical charges between them. Briefly, the histones are positively charged due to the presence of a large amount of positively charged amino acids (mainly lysine and arginine). On the other hand, DNA is negatively charged and thus interaction of positive and negative charges maintains the structural integrity of the nucleosome. Unlike DNA methylation, histone modification exclusively occurs at the amino-terminal tail protruding out of the histone subunit and is a short-term reversible modification. The amino-terminal tails are subjected to post-translational modification namely methylation, acetylation, phosphorylation, and ubiquitination (Yu et al., 2021). Post-translational modification of amino-terminal tails is associated with DNA repair, activation or repression of gene expression, telomere integrity, and the total interaction changes in response to these modifications are determined by “histone code” (Ng et al., 2018).
In humans or mammals, the immune system, especially innate immune cells, plays a decisive role in producing signals depending on the response in cerebrovascular events. The predominant innate immune cell in the CNS is microglia, along with subsidiary infiltrating myeloid cells because of the disruption of the BBB. Microglia, even under resting conditions, constantly monitor the surrounding microenvironment and act promptly per changes (Wesselingh et al., 2019). Activated microglia are subjected to altering their morphology, gene expression, and consequently undertaking their role per the changes in the microenvironment (Cherry et al., 2014). Similar to macrophages, pro-inflammatory microglia (M1) has been illustrated to up-regulate inflammatory genes namely interleukin-1 alpha/beta (IL-1α/β), interleukin-6 (IL-6), interleukin-12 (IL-12), interleukin-23 (IL-23), tumor necrosis factor-alpha (TNF-α), inducible nitric oxide synthase (iNOS) whereas the anti-inflammatory subtype (M2) has been illustrated to up-regulate neuroprotective genes such as arginase 1 (Arg-1), insulin-like growth factor-1 (IGF-1), chitinase-3-like protein 3 (Chi3l3/Ym-1), and found in the inflammatory zone (FIZZ) (Cao and He, 2013; Caldeira et al., 2017; Salvi et al., 2017; Zhou T. et al., 2017). Simultaneous down-regulation of M1 and up-regulation of the M2 phenotype in post-stroke conditions can be beneficial in minimizing the post-stroke insults.
Histone 3 lysine acetylation (H3KAc) is up-regulated in microglia around the peri-infarct and infarct zone after ischemic stroke. Similar up-regulation in H3KAc was also noted in lipopolysaccharide (LPS) mediated microglial activation. Thus, H3KAc up-regulation is highly associated with inflammatory cytokines. Histone deacetylase (HDAC) is a key regulator of H3KAc (Demyanenko et al., 2020; Fessler et al., 2013; Kong et al., 2018). HDAC inhibition promotes the downregulation of pro-inflammatory genes, such as TNF-α, iNOS, signal transducer and activator of transcription 1 (STAT1), and IL-6, and up-regulation of interleukin-10 (IL-10) and signal transducer and activator of transcription (STAT3) genes in activated microglia, both in vivo and vitro. The up-regulation of anti-inflammatory genes promotes neuronal survival, reduction in brain infarct volume, and suppression of microglia activation (M1) which shows the neuroprotective abilities of HDAC inhibitors (Kim et al., 2007; Patnala et al., 2017). HDAC6, as an adaptor, can affect aggrephagy in CNS. For instance, HDAC6-mediated aggregation is associated with retrograde axonal transport (Xu et al., 2021). Suberoylanilide hydroxamic acid (SAHA), which is an HDAC inhibitor, has been exhibited to up-regulate 70 kilodalton heat shock protein (Hsp70; essential for protein folding and stress-related protection in cells) and B-cell lymphoma 2 (Bcl-2; anti-apoptotic) along with the reduction of pro-inflammatory cytokines, thus preventing neuronal loss and promoting favorable outcome in post-stroke condition (Faraco et al., 2006; Langley et al., 2009; Abend and Kehat, 2015; Jhelum et al., 2017).
Apart from SAHA, other HDAC inhibitors such as valproic acid (VPA), sodium butyrate (SB), trichostatin-A (TSA), and sodium 4-phenylbutyrate (4-PBA) have been shown to promote similar neuroprotective abilities by regulation of excitotoxicity, oxidative stress, endoplasmic reticulum stress (ER-stress), apoptosis, inflammation, and BBB breakdown (Fessler et al., 2013). Reactive oxygen species (ROS) have a well-established association with cerebrovascular accidents (Olmez and Ozyurt, 2012; Qu et al., 2016). Nuclear factor erythroid 2-related factor 2 (Nrf-2) has been identified as a key regulator in ROS-dependent oxidative insults to CNS (Li et al., 2011; Yamauchi et al., 2016). Up-regulation of Nrf-2 using HDAC inhibitors such as VPA and TSA has been exemplified to promote neuroprotection against oxidative stress (Correa et al., 2011; Fessler et al., 2013).
Histone methylation has also been extensively explored to determine factors associated with prognostic outcomes in both pre and post-stroke conditions. Aging is one of the principal determinants of functional outcomes in cerebrovascular accidents (Manwani et al., 2011; Zhang et al., 2018) and is highly associated with a reduction in brain plasticity (Guggisberg et al., 2019; Nesin et al., 2019). A murine study revealed a significant reduction of Trimethylation of Histone H3 at lysine 4 (H3K4me3) in cortical astrocytes with progression in age (Chisholm et al., 2015). Histone 3 lysine 9 (H3K9) has also been identified as a potential target therapy region as inhibition of Histone-lysine N-methyltransferase SUV39H1 and Euchromatic histone-lysine N-methyltransferase 2 (G9a) promotes up-regulation of brain-derived neurotrophic factor (BDNF) in E17 neuronal cells (Schweizer et al., 2015). Another study using dimethyloxalylglycine (DMOG) to inhibit histone lysine demethylase subfamily 4 (KDM4) has been shown to promote neuronal repair via H3K9me2 dependent manner in CD1 mice (Chakravarty et al., 2017).
Apart from histone acetylation and methylation, post-translational phosphorylation has also been identified in cerebral ischemic conditions (Crowe et al., 2006; Song et al., 2010; Liu et al., 2014; Zhao et al., 2016). Crowe SL and colleagues demonstrated an increase in ionotropic glutamate receptor (NMDA) activity that promotes histone phosphorylation (γ-H2A.X) in rat cortical neurons. However, pretreatment with vitamin E and BAPTA-AM (calcium chelator) attenuated γ-H2A.X formation (Crowe et al., 2006). A study using the Drosophila model demonstrated neuronal necrosis through phosphorylation of histone 3 serine 28 (H3S28Ph) (Liu et al., 2014). A list of commonly undertaken histone modification and histone binding modules has been enlisted in Figures 3A–C.
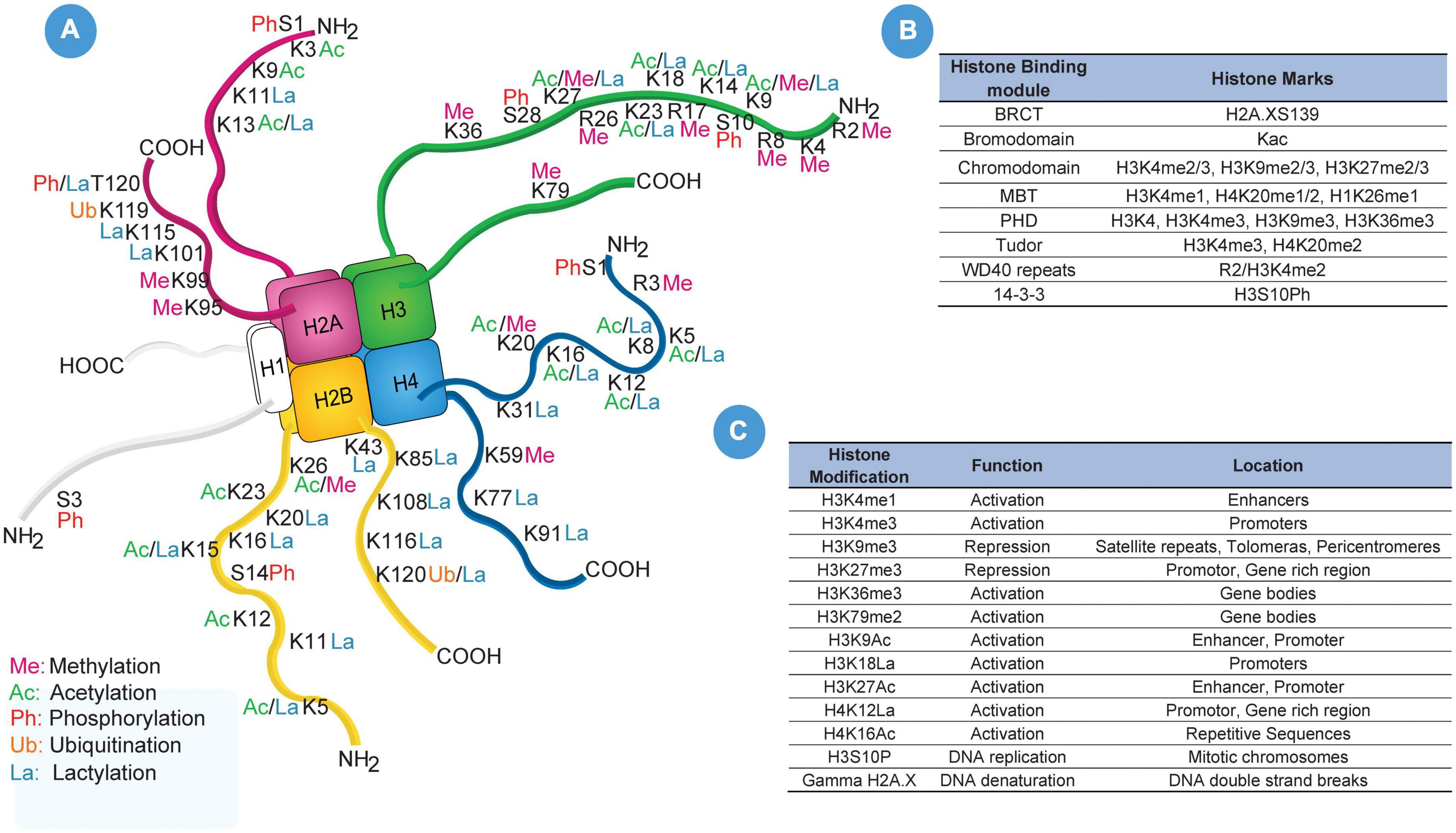
Figure 3. Histone Modifications. (A) Illustrates pictorial representation of all known till date post-translational modification of histone amino-terminal tail and their location regions. (B) Portraits frequency used histone marks and their histone binding modules such as BRCT, bromodomain, chromodomain, MBT, PHD, Tudor, WD40 repeats and 14-3-3. (C) List of frequently used histone-modified regions, functions, and locations in DNA sequence which includes H3K4me1, H3K4me3, H3K9me3, H3K27me3, H3K36me3, H3K79me2, H3K9Ac, H3K9La, H3K27Ac, H4K12La, H4K16Ac, H3S10P, and Gamma H2A.X.
DNA methylation
DNA methylation has been one of the most extensively studied epigenetic modifications, exclusively occurring at CpG dinucleotides in mammals and always symmetrical to maintain the methylation during the cell division process. Notably, DNA methylation depends on the balance between hyper and hypomethylation activity. DNA methylation is carried out by de novo methyltransferases (DNMT); precisely DNMT3a and DNMT3b in mammals. CpGs are clustered into CpG islands, often at the promotor site of the gene. CpG island tends to be protected from methylation. Methylation observed at CpG island is entirely associated with the silencing of gene expression and carried out either by the formation of repressive chromatin structure or inhibiting transcription factor binding and alteration of gene expression.
Long interspersed nuclear element-1 (LINE-1), which is a class I transposable element in the DNA and a member of LINEs has been the center of many study discussions after their discovery concerning the association in predicting increased risk of ischemic stroke and cardiovascular events. Hypomethylation of LINE-1 is associated with an increased risk of ischemic stroke (Baccarelli et al., 2010b; Lin et al., 2014; Soriano-Tarraga et al., 2014; Ng et al., 2018). However, a single sex-specific analytic study has demonstrated that LINE-1 hypomethylation is suggestive of advanced atherosclerotic lesions, which leads to global hypomethylation and has more association in determining the risk of development of ischemic stroke in men as compared to that of women (Lin et al., 2014). A further investigation reported the co-relation between hypomethylation of LINE-1 and an increased level of circulating vascular cell adhesion molecule-1 (VCAM-1) (Baccarelli et al., 2010a).
A cross-sectional study was conducted on the Japanese population aiming to determine the relationship between methylation of LINE-1 in leukocytes and that dyslipidemia. Hypomethylation of LINE-1 in leukocytes was showcased to have a higher odds ratio in individuals with dyslipidemia (Tsuboi et al., 2018). Thus, the methylation status of LINE-1 can be a key risk factor predictor. Similarly, hypomethylation of TNF receptor-associated factor 3 (TRAF3) and hypermethylation of thrombospondin-1 (THBS1) has also been illustrated to be crucial predictor of stroke-related outcomes (Lopez-Dee et al., 2011; Udali et al., 2013; Gallego-Fabrega et al., 2016; Ng et al., 2018). DNMT, especially DNMT1 and DNMT3a has also been identified as pivotal enzymes regulating methylation of various genes (Feng et al., 2010; Wu et al., 2012; Gustafsson et al., 2018), of which DNMT1-dependent DNA methylation has been pinpointed as a mediator of chronic inflammation and development of atherosclerotic disease via the peroxisome proliferator-activated receptor gamma (PPAR-γ) pathway (Yu et al., 2016). On the other hand, DNMT3a has also been identified to promote ischemic brain damage (Morita et al., 2013; Pandi et al., 2013). Thus, DNA hypomethylation may be a potential therapeutic strategy for the treatment of stroke (Sharifulina et al., 2021).
Matrix metalloproteinase-2 (MMP-2) is one of the most studied enzymes concerning their changes in peripheral blood concentration both in acute and chronic phases of post-stroke symptoms (Fatar et al., 2008; Kreisel et al., 2012, 2016). However, various studies have produced not identical data, creating confusion within the research field. A study conducted over a sample size of 556 participants (298 with ischemic stroke versus 258 control) successfully showcased a lower concentration of MMP-2 methylation level in peripheral blood exclusively in male small-vessel occlusion participants (Lin et al., 2017). Thus, narrowing the use of MMP-2 serum concentration as an effective marker in post-ischemic stroke. Apart from the common methylation at the fifth position of the pyrimidine ring of cytosine (5mC), other forms of modifications are also noted at a similar position namely, 5-hydroxymethyl (5hmC), 5-formal (5fC), and 5-carboxyl (5caC). Various studies have successfully showcased 5-hmC to regulate several cellular processes which include neuronal development as well. A neoteric study was conducted in murine specie (mouse), demonstrating the use of ascorbate (mineral salt of ascorbic acid; vitamin C) in post-stroke reperfusion led to Ten-eleven translocation 3 (TET3) dependent conversion of 5mC to 5hmC, promoting up-regulation of neuroprotective genes and functional recovery (Morris-Blanco et al., 2019).
5-aza-2′-deoxycytidine which is a DNA methyltransferase inhibitor (DNA methylation inhibitor) has been illustrated to significantly reduce the infarct volume (Endres et al., 2000). Likewise, another study using zebularine, which is also a DNA methylation inhibitor, has demonstrated dose-dependent (500 μg and 100 μg) reduction in infarct volume (Dock et al., 2015).
RNA modification
Similar to DNA modifications, RNA modifications have also been shown to be a regulator of gene expression (Li et al., 2017; Engel and Chen, 2018; Coker et al., 2019; Sendinc et al., 2019). To date, RNA modifications include N6-methyladenosine (m6A), N6,2′-O-dimethyladenosine (m6Am), N1-methyladenosine (m1A), 5-methylcytosine (m5C), 5-hydroxymethylcytosine (hm5C), N4-acetylcytidine (ac4C), rotation isomerization of uridine/pseudouridine (ψ) and 7-Methylguanosine (m7G) (Figure 4). m6A is one of the most commonly observed mRNA modifications (Ji et al., 2018) and was identified in the 1970s (Desrosiers et al., 1974; Adams and Cory, 1975; Aloni et al., 1979). However, their association with small nuclear RNAs (snRNAs), micro-RNAs (miRNAs) circular RNA (circRNAs), and long non-coding RNAs (lncRNAs) has been recently understood (Dominissini et al., 2012; Chen et al., 2020). Mapping of m6A over human and murine RNA has identified over 18,000 m6A sites in 7,000 human genes with a consensus sequence of [G/A/U][G > A] m6A[U > A/C] (Dominissini et al., 2012; Meyer et al., 2012; Sun et al., 2016). m6A has also been shown to be changed during embryonic brain development and cerebral ischemic conditions (Meyer et al., 2012; Li et al., 2022). Furthermore, the silencing of m6A methyltransferase affects gene expression and modulates the p53 (TRP53) signaling pathway and apoptosis (Dominissini et al., 2012). Likewise, m6Am, m1A, m5C, hm5C, ac4, C, ψ, and m7G are somewhat understood in the context of cancer and as potential biomarkers. For example, m1A was identified as a modulator in cerebral ischemic stroke (Chokkalla et al., 2022), and m6A was showcased to regulate the brain functions, development of synaptic plasticity, and their association with neuropsychiatric disorders (Yoon et al., 2017).
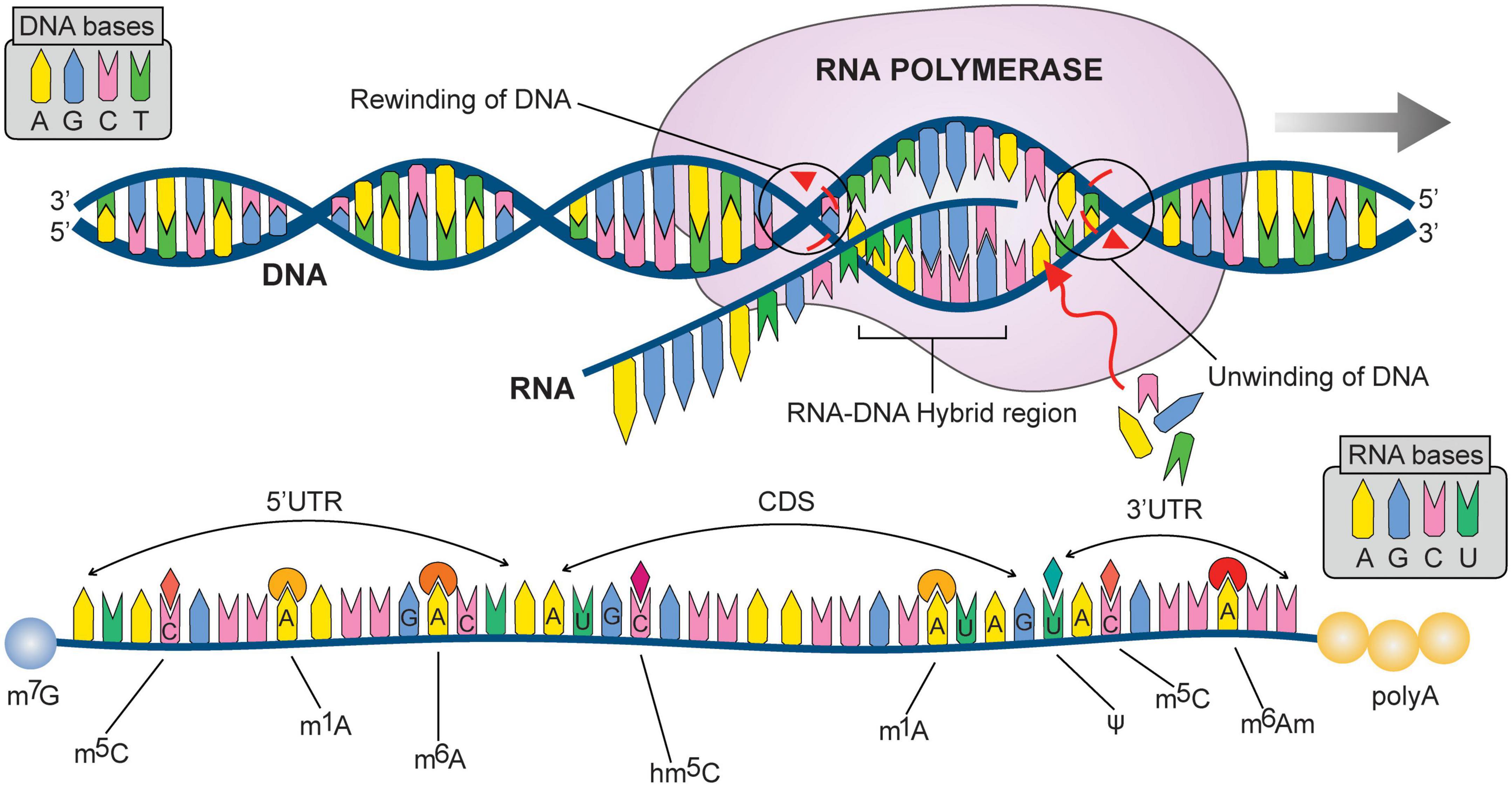
Figure 4. Illustrate DNA to RNA transcription (followed by possible mRNA (messenger RNA) modifications at different nitrogen bases). N6-methyladenosine m6A; Pseudouridine (Ψ); 5-methylcytosine (m6C); 5-hydroxymethylcytidine (hm6C); N1-methyladenosine (m1A); 7-Methylguanosine (m7G); N6,2′-O-dimethyladenosine (m6Am). 3′UTR: Three prime untranslated regions; CDS: CoDing Sequence; 5′UTR: Five prime untranslated regions.
Cumulatively, epigenetic mechanisms offer a promising new therapeutic target in ischemia (Table 1). Histone/DNA/RNA modifications have been widely studied over the last decade. However, their contributions to stroke pathophysiological processes (including hemorrhagic and ischemic stroke) are still limited. Further clinical studies should assess whether these targets can restore or enhance significantly clinical outcomes of stroke patients.
Prospect
As a result of interventions in the hyperacute phase, the mortality of stroke has declined substantially. However, long-term disability and institutionalization of the post-stroke remain unchanged. Stroke is a complex, multifactorial disease in which a wide plethora of pathological processes are simultaneously set in motion. Modulation of a single molecular factor is unlikely to be sufficient to attenuate or reverse the progression of stroke pathology. Epigenetic alterations such as DNA methylation, histone modifications, and RNA modifications are potent modulators of gene regulation, and an accumulating body of evidence suggests that they play a pivotal role in regulating brain remodeling after stroke. As a result, efforts are being made to identify key molecular signatures and development of combination therapy strategies similar to cancer (Dawson and Kouzarides, 2012).
Specifically, DNA methylation has been one of the heavily researched topics over the last decade and their association with risk factor prediction has been well documented. For example, DNA methylation of Cyclin-dependent kinase inhibitor 2B (CDKN2B) has been showcased to promote an increased risk of arterial calcification in ischemic stroke patients (Zhou et al., 2016; Zhou S. et al., 2017). Similarly, histone modifications have been illustrated to be a regulator of gene expression (Crowe et al., 2006; Kim et al., 2007; Schweizer et al., 2015; Patnala et al., 2017). Furthermore, strokes could cause an increase in anaerobic metabolism and lactic acidosis. Recently, a novel function for lactate is utilized in a new histone modification, histone lysine lactylation (Zhang et al., 2019; Figure 2). Pan et al. report an H4K12 lactylation positive feedback loop in microglial inflammation (Pan et al., 2022). This epigenetic mechanism may bring forth new biology and functionality to the role of metabolic homeostasis in regulating the secondary brain after stroke. The antagomir approach has been proven to promote neuroprotective effects in animal models of stroke and potential treatment strategies for the subsequent trend in epigenetics. Pharmacological inhibitors of these epigenetic modifications have been studied in animal models of stroke (Tang et al., 2017) and are readily available as treatment options in the clinic (Santini et al., 2013).
Over the years, several clinical studies or clinical trials were conducted to determine effective treatment after hemorrhagic and ischemic strokes. As mentioned earlier, HDAC inhibition could promote the downregulation of pro-inflammatory genes. VPA, a nitrogen-free broad-spectrum antiepileptic compound, has been used clinically for decades due to its effect on the decrease in neuronal hyperexcitability both by strengthening GABAergic transmission and by inhibiting sodium/especially calcium ion channels and HDACs. Previous clinical trials (Trial No. NCT01115959) reported that VPA-treated ICH patients had improved the National Institute of health stroke scale (NIHSS) scores (Gilad et al., 2011; Brookes et al., 2018). An ongoing study (Trial No. ChiCTR2100050161) also focuses on the effects of sodium valproate in patients with SAH (Chen et al., 2022). Other histone modification-related drugs, including Fluoxetine and Sildenafil citrate, are reported in clinical trials for both hemorrhagic and ischemic stroke (Chollet et al., 2011; Washington et al., 2016; Dennis et al., 2020; Marquez-Romero et al., 2020). Although clinical studies (Trial No. ISRCTN83290762, NCT00657163, NCT01737541) reported that Fluoxetine did not improve patients’ functional outcomes, early prescription of Fluoxetine with physiotherapy enhanced motor recovery in AIS and ICH patients (Chollet et al., 2011; Dennis et al., 2020; Marquez-Romero et al., 2020). Other epigenetic therapies, such as D-cycloserine (Trial No. NCT02082912), intra-arterial autologous bone marrow mononuclear cells injection (RNA modification, Trial No. NCT02178657), intravenous transplantation of autologous mesenchymal stem cells expanded with autologous serum (involved in non-coding RNA functions, Trial No. NCT01716481) also showed beneficial effects in ischemic stroke patients (Butler et al., 2015; Mancha et al., 2020; Bang et al., 2022) (Table 2).
Overall, previous studies have successfully demonstrated that stroke leads to epigenetic dysregulation which in turn triggers a series of cascade changes that cause neuroinflammation, oxidative stress, apoptosis, and several other secondary injury events. Other epigenetic modifications (such as acetylation, phosphorylation, and lactylation) and epigenetic regulators (such as lncRNAs, circRNAs, and miRNAs), although not discussed in this review, were also reported as translational targets in stroke research. Agents targeting epigenetic regulation are under development and entering clinical trials. Epigenetic modifications, such as methylation or non-coding RNA expression levels, may play a crucial role in antiplatelet treatment for stroke patients (Danielak et al., 2022). Regulation of these key triggers would be beneficial to produce the desired outcome in post-stroke conditions.
Conclusion
Advancements in epigenetics research have led us to further understand the mechanisms of secondary injury. Future understanding of the key modulators at the molecular level and combination therapies would be new management strategies in post-stroke conditions.
Author contributions
JPe conceived the entire review project, conceptualization, and literature search. FZ, LY, JW, and JPa drafted the figures. JPe, LZ, SY, and YJ wrote the manuscript. YJ overviewed and guided the conception of the entire project. All the authors contributed to the critical revision of the final manuscript.
Funding
This work was supported by the National Natural Science Foundation of China (82271306 and 81971132) and grants from the Young Elite Scientist Sponsorship Program by the China Association for Science and Technology (YESS20200178), the Sichuan Science and Technology Program (2021ZYD0106 and 2021ZYD0091), and Luzhou Government-Southwest Medical University Strategic Cooperation Project and Southwest Medical University Project (2021LZXNYD-P01 and 2021ZKZD013).
Acknowledgments
One of the co-authors, DG, deceased during the manuscript preparation. In memoriam of Mr. Dipritu Ghosh, we would like to acknowledge his contribution to the research.
Conflict of interest
The authors declare that the research was conducted in the absence of any commercial or financial relationships that could be construed as a potential conflict of interest.
Publisher’s note
All claims expressed in this article are solely those of the authors and do not necessarily represent those of their affiliated organizations, or those of the publisher, the editors and the reviewers. Any product that may be evaluated in this article, or claim that may be made by its manufacturer, is not guaranteed or endorsed by the publisher.
References
Abend, A., and Kehat, I. (2015). Histone deacetylases as therapeutic targets–from cancer to cardiac disease. Pharmacol. Ther. 147, 55–62. doi: 10.1016/j.pharmthera.2014.11.003
Adams, J. M., and Cory, S. (1975). Modified nucleosides and bizarre 5′-termini in mouse myeloma mRNA. Nature 255, 28–33. doi: 10.1038/255028a0
Aloni, Y., Dhar, R., and Khoury, G. (1979). Methylation of nuclear simian virus 40 RNAs. J. Virol. 32, 52–60. doi: 10.1128/JVI.32.1.52-60.1979
Aslanyan, S., Weir, C. J., Lees, K. R., Reid, J. L., and McInnes, G. T. (2003). Effect of area-based deprivation on the severity, subtype, and outcome of ischemic stroke. Stroke 34, 2623–2628. doi: 10.1161/01.STR.0000097610.12803.D7
Avan, A., Digaleh, H., Di Napoli, M., Stranges, S., Behrouz, R., Shojaeianbabaei, G., et al. (2019). Socioeconomic status and stroke incidence, prevalence, mortality, and worldwide burden: An ecological analysis from the Global Burden of Disease Study 2017. BMC Med. 17:191. doi: 10.1186/s12916-019-1397-3
Baccarelli, A., Wright, R., Bollati, V., Litonjua, A., Zanobetti, A., Tarantini, L., et al. (2010b). Ischemic heart disease and stroke in relation to blood DNA methylation. Epidemiology 21, 819–828. doi: 10.1097/EDE.0b013e3181f20457
Baccarelli, A., Tarantini, L., Wright, R. O., Bollati, V., Litonjua, A. A., Zanobetti, A., et al. (2010a). Repetitive element DNA methylation and circulating endothelial and inflammation markers in the VA normative aging study. Epigenetics 5, 222–228. doi: 10.4161/epi.5.3.11377
Bang, O. Y., Kim, E. H., Cho, Y. H., Oh, M. J., Chung, J. W., Chang, W. H., et al. (2022). Circulating Extracellular Vesicles in Stroke Patients Treated With Mesenchymal Stem Cells: A Biomarker Analysis of a Randomized Trial. Stroke 53, 2276–2286. doi: 10.1161/STROKEAHA.121.036545
Bourhy, L., Mazeraud, A., Bozza, F. A., Turc, G., Lledo, P. M., and Sharshar, T. (2022). Neuro-Inflammatory Response and Brain-Peripheral Crosstalk in Sepsis and Stroke. Front. Immunol. 13:834649. doi: 10.3389/fimmu.2022.834649
Brookes, R. L., Crichton, S., Wolfe, C. D. A., Yi, Q., Li, L., Hankey, G. J., et al. (2018). Sodium Valproate, a Histone Deacetylase Inhibitor, Is Associated With Reduced Stroke Risk After Previous Ischemic Stroke or Transient Ischemic Attack. Stroke 49, 54–61. doi: 10.1161/STROKEAHA.117.016674
Butler, A. J., Kallos, J., Housley, S. N., LaPlaca, M. C., Traynelis, S. F., and Wolf, S. L. (2015). Randomized, Placebo-Controlled, Double-Blind Pilot Study of D-Cycloserine in Chronic Stroke. Rehabil. Res. Pract. 2015:534239. doi: 10.1155/2015/534239
Caldeira, C., Cunha, C., Vaz, A. R., Falcao, A. S., Barateiro, A., Seixas, E., et al. (2017). Key Aging-Associated Alterations in Primary Microglia Response to Beta-Amyloid Stimulation. Front. Aging Neurosci. 9:277. doi: 10.3389/fnagi.2017.00277
Cannegieter, S. C., Rosendaal, F. R., and Briet, E. (1994). Thromboembolic and bleeding complications in patients with mechanical heart valve prostheses. Circulation 89, 635–641. doi: 10.1161/01.cir.89.2.635
Cao, L., and He, C. (2013). Polarization of macrophages and microglia in inflammatory demyelination. Neurosci. Bull. 29, 189–198. doi: 10.1007/s12264-013-1324-0
Chakravarty, S., Jhelum, P., Bhat, U. A., Rajan, W. D., Maitra, S., Pathak, S. S., et al. (2017). Insights into the epigenetic mechanisms involving histone lysine methylation and demethylation in ischemia induced damage and repair has therapeutic implication. Biochim. Biophys. Acta Mol. Basis Dis. 1863, 152–164. doi: 10.1016/j.bbadis.2016.09.014
Chen, Y. H., Kang, J. H., and Lin, H. C. (2011). Patients with traumatic brain injury: Population-based study suggests increased risk of stroke. Stroke 42, 2733–2739. doi: 10.1161/STROKEAHA.111.620112
Chen, Y., Fang, M., Wu, P., Xie, Z., Wu, H., Wu, Q., et al. (2022). Seizure prophylaxis following aneurysmal subarachnoid haemorrhage (SPSAH): Study protocol for a multicentre randomised placebo-controlled trial of short-term sodium valproate prophylaxis in patients with acute subarachnoid haemorrhage. BMJ Open 12:e057917. doi: 10.1136/bmjopen-2021-057917
Chen, Y., Lin, Y., Shu, Y., He, J., and Gao, W. (2020). Interaction between N(6)-methyladenosine (m(6)A) modification and noncoding RNAs in cancer. Mol. Cancer 19:94. doi: 10.1186/s12943-020-01207-4
Cherry, J. D., Olschowka, J. A., and O’Banion, M. K. (2014). Neuroinflammation and M2 microglia: The good, the bad, and the inflamed. J. Neuroinflamm. 11:98. doi: 10.1186/1742-2094-11-98
Chisholm, N. C., Henderson, M. L., Selvamani, A., Park, M. J., Dindot, S., Miranda, R. C., et al. (2015). Histone methylation patterns in astrocytes are influenced by age following ischemia. Epigenetics 10, 142–152. doi: 10.1080/15592294.2014.1001219
Chokkalla, A. K., Pajdzik, K., Dou, X., Dai, Q., Mehta, S. L., Arruri, V., et al. (2022). Dysregulation of the Epitranscriptomic Mark m(1)A in Ischemic Stroke. Transl. Stroke Res. [Epub ahead of print]. doi: 10.1007/s12975-022-01056-x
Chollet, F., Tardy, J., Albucher, J. F., Thalamas, C., Berard, E., Lamy, C., et al. (2011). Fluoxetine for motor recovery after acute ischaemic stroke (FLAME): A randomised placebo-controlled trial. Lancet Neurol. 10, 123–130. doi: 10.1016/S1474-4422(10)70314-8
Coker, H., Wei, G., and Brockdorff, N. (2019). M6a modification of non-coding RNA and the control of mammalian gene expression. Biochim. Biophys. Acta Gene Regul. Mech. 1862, 310–318. doi: 10.1016/j.bbagrm.2018.12.002
Collaborators, G. B. D. S. (2019). Global, regional, and national burden of stroke, 1990-2016: A systematic analysis for the Global Burden of Disease Study 2016. Lancet Neurol. 18, 439–458. doi: 10.1016/S1474-4422(19)30034-1
Corces, M. R., Shcherbina, A., Kundu, S., Gloudemans, M. J., Fresard, L., Granja, J. M., et al. (2020). Single-cell epigenomic analyses implicate candidate causal variants at inherited risk loci for Alzheimer’s and Parkinson’s diseases. Nat. Genet. 52, 1158–1168. doi: 10.1038/s41588-020-00721-x
Correa, F., Mallard, C., Nilsson, M., and Sandberg, M. (2011). Activated microglia decrease histone acetylation and Nrf2-inducible anti-oxidant defence in astrocytes: Restoring effects of inhibitors of HDACs, p38 MAPK and GSK3beta. Neurobiol. Dis. 44, 142–151. doi: 10.1016/j.nbd.2011.06.016
Crowe, S. L., Movsesyan, V. A., Jorgensen, T. J., and Kondratyev, A. (2006). Rapid phosphorylation of histone H2A.X following ionotropic glutamate receptor activation. Eur. J. Neurosci. 23, 2351–2361. doi: 10.1111/j.1460-9568.2006.04768.x
Danielak, D., Pawlak, K., Glowka, F., and Karazniewicz-Lada, M. (2022). Influence of Genetic and Epigenetic Factors of P2Y12 Receptor on the Safety and Efficacy of Antiplatelet Drugs. Cardiovasc. Drugs Ther. [Epub ahead of print]. doi: 10.1007/s10557-022-07370-8
Dawson, M. A., and Kouzarides, T. (2012). Cancer epigenetics: From mechanism to therapy. Cell 150, 12–27. doi: 10.1016/j.cell.2012.06.013
Demyanenko, S. V., Dzreyan, V. A., Neginskaya, M. A., and Uzdensky, A. B. (2020). Expression of Histone Deacetylases HDAC1 and HDAC2 and Their Role in Apoptosis in the Penumbra Induced by Photothrombotic Stroke. Mol. Neurobiol. 57, 226–238. doi: 10.1007/s12035-019-01772-w
Dennis, M., Forbes, J., Graham, C., Hackett, M., Hankey, G. J., House, A., et al. (2020). Fluoxetine to improve functional outcomes in patients after acute stroke: The FOCUS RCT. Health Technol. Assess. 24, 1–94. doi: 10.3310/hta24220
Desrosiers, R., Friderici, K., and Rottman, F. (1974). Identification of methylated nucleosides in messenger RNA from Novikoff hepatoma cells. Proc. Natl. Acad. Sci. U.S.A. 71, 3971–3975. doi: 10.1073/pnas.71.10.3971
Dock, H., Theodorsson, A., and Theodorsson, E. D. N. A. (2015). Methylation inhibitor zebularine confers stroke protection in ischemic Rats. Transl. Stroke Res. 6, 296–300. doi: 10.1007/s12975-015-0397-7
Dominissini, D., Moshitch-Moshkovitz, S., Schwartz, S., Salmon-Divon, M., Ungar, L., Osenberg, S., et al. (2012). Topology of the human and mouse m6A RNA methylomes revealed by m6A-seq. Nature 485, 201–206. doi: 10.1038/nature11112
Endres, M., Meisel, A., Biniszkiewicz, D., Namura, S., Prass, K., Ruscher, K., et al. (2000). DNA methyltransferase contributes to delayed ischemic brain injury. J. Neurosci. 20, 3175–3181. doi: 10.1523/JNEUROSCI.20-09-03175.2000
Engel, M., and Chen, A. (2018). The emerging role of mRNA methylation in normal and pathological behavior. Genes Brain Behav. 17:e12428. doi: 10.1111/gbb.12428
Eser Ocak, P., Ocak, U., Sherchan, P., Gamdzyk, M., Tang, J., and Zhang, J. H. (2020). Overexpression of Mfsd2a attenuates blood brain barrier dysfunction via Cav-1/Keap-1/Nrf-2/HO-1 pathway in a rat model of surgical brain injury. Exp. Neurol. 326:113203. doi: 10.1016/j.expneurol.2020.113203
Faraco, G., Pancani, T., Formentini, L., Mascagni, P., Fossati, G., Leoni, F., et al. (2006). Pharmacological inhibition of histone deacetylases by suberoylanilide hydroxamic acid specifically alters gene expression and reduces ischemic injury in the mouse brain. Mol. Pharmacol. 70, 1876–1884. doi: 10.1124/mol.106.027912
Fatar, M., Stroick, M., Steffens, M., Senn, E., Reuter, B., Bukow, S., et al. (2008). Single-nucleotide polymorphisms of MMP-2 gene in stroke subtypes. Cerebrovasc. Dis. 26, 113–119. doi: 10.1159/000139657
Feng, J., Zhou, Y., Campbell, S. L., Le, T., Li, E., Sweatt, J. D., et al. (2010). Dnmt1 and Dnmt3a maintain DNA methylation and regulate synaptic function in adult forebrain neurons. Nat. Neurosci. 13, 423–430. doi: 10.1038/nn.2514
Fessler, E. B., Chibane, F. L., Wang, Z., and Chuang, D. M. (2013). Potential roles of HDAC inhibitors in mitigating ischemia-induced brain damage and facilitating endogenous regeneration and recovery. Curr. Pharm. Des. 19, 5105–5120. doi: 10.2174/1381612811319280009
Freedman, A. N., Eaves, L. A., Rager, J. E., Gavino-Lopez, N., Smeester, L., Bangma, J., et al. (2022). The placenta epigenome-brain axis: Placental epigenomic and transcriptomic responses that preprogram cognitive impairment. Epigenomics 14, 897–911. doi: 10.2217/epi-2022-0061
Gallego-Fabrega, C., Carrera, C., Reny, J. L., Fontana, P., Slowik, A., Pera, J., et al. (2016). TRAF3 epigenetic regulation is associated with vascular recurrence in patients with ischemic stroke. Stroke 47, 1180–1186. doi: 10.1161/STROKEAHA.115.012237
Gamdzyk, M., Doycheva, D. M., Araujo, C., Ocak, U., Luo, Y., Tang, J., et al. (2020). CGAS/STING pathway activation contributes to delayed neurodegeneration in neonatal hypoxia-ischemia rat model: possible involvement of LINE-1. Mol. Neurobiol. 57, 2600–2619. doi: 10.1007/s12035-020-01904-7
Gilad, R., Boaz, M., Dabby, R., Sadeh, M., and Lampl, Y. (2011). Are post intracerebral hemorrhage seizures prevented by anti-epileptic treatment? Epilepsy Res. 95, 227–231. doi: 10.1016/j.eplepsyres.2011.04.002
Go, A. S., Hylek, E. M., Phillips, K. A., Chang, Y., Henault, L. E., Selby, J. V., et al. (2001). Prevalence of diagnosed atrial fibrillation in adults: National implications for rhythm management and stroke prevention: The AnTicoagulation and Risk Factors in Atrial Fibrillation (ATRIA) Study. JAMA 285, 2370–2375. doi: 10.1001/jama.285.18.2370
Gottdiener, J. S., Gay, J. A., VanVoorhees, L., DiBianco, R., and Fletcher, R. D. (1983). Frequency and embolic potential of left ventricular thrombus in dilated cardiomyopathy: Assessment by 2-dimensional echocardiography. Am. J. Cardiol. 52, 1281–1285. doi: 10.1016/0002-9149(83)90588-x
Grau, A. J., Weimar, C., Buggle, F., Heinrich, A., Goertler, M., Neumaier, S., et al. (2001). Risk factors, outcome, and treatment in subtypes of ischemic stroke: The German stroke data bank. Stroke 32, 2559–2566. doi: 10.1161/hs1101.098524
Guggisberg, A. G., Koch, P. J., Hummel, F. C., and Buetefisch, C. M. (2019). Brain networks and their relevance for stroke rehabilitation. Clin. Neurophysiol. 130, 1098–1124. doi: 10.1016/j.clinph.2019.04.004
Gustafsson, J. R., Katsioudi, G., Degn, M., Ejlerskov, P., Issazadeh-Navikas, S., and Kornum, B. R. (2018). DNMT1 regulates expression of MHC class I in post-mitotic neurons. Mol. Brain 11:36. doi: 10.1186/s13041-018-0380-9
Havdahl, A., Niarchou, M., Starnawska, A., Uddin, M., van der Merwe, C., and Warrier, V. (2021). Genetic contributions to autism spectrum disorder. Psychol. Med. 51, 2260–2273. doi: 10.1017/S0033291721000192
Iglesias-Rey, R., da Silva-Candal, A., Rodriguez-Yanez, M., Estany-Gestal, A., Regueiro, U., Maqueda, E., et al. (2022). Neurological instability in ischemic stroke: Relation with outcome, latency time, and molecular markers. Transl. Stroke Res. 13, 228–237. doi: 10.1007/s12975-021-00924-2
Jhelum, P., Karisetty, B. C., Kumar, A., and Chakravarty, S. (2017). Implications of epigenetic mechanisms and their targets in cerebral ischemia models. Curr. Neuropharmacol. 15, 815–830. doi: 10.2174/1570159X14666161213143907
Ji, P., Wang, X., Xie, N., and Li, Y. (2018). N6-Methyladenosine in RNA and DNA: An epitranscriptomic and epigenetic player implicated in determination of stem cell fate. Stem Cells Int. 2018:3256524. doi: 10.1155/2018/3256524
Kamel, H., and Healey, J. S. (2017). Cardioembolic stroke. Circ. Res. 120, 514–526. doi: 10.1161/CIRCRESAHA.116.308407
Kamel, H., Okin, P. M., Elkind, M. S., and Iadecola, C. (2016). Atrial fibrillation and mechanisms of stroke: Time for a new model. Stroke 47, 895–900. doi: 10.1161/STROKEAHA.115.012004
Kim, H. J., Rowe, M., Ren, M., Hong, J. S., Chen, P. S., and Chuang, D. M. (2007). Histone deacetylase inhibitors exhibit anti-inflammatory and neuroprotective effects in a rat permanent ischemic model of stroke: Multiple mechanisms of action. J. Pharmacol. Exp. Ther. 321, 892–901. doi: 10.1124/jpet.107.120188
Kim, W., and Kim, E. J. (2018). Heart failure as a risk factor for stroke. J. Stroke 20, 33–45. doi: 10.5853/jos.2017.02810
Kolominsky-Rabas, P. L., Weber, M., Gefeller, O., Neundoerfer, B., and Heuschmann, P. U. (2001). Epidemiology of ischemic stroke subtypes according to TOAST criteria: Incidence, recurrence, and long-term survival in ischemic stroke subtypes: A population-based study. Stroke 32, 2735–2740. doi: 10.1161/hs1201.100209
Kong, Q., Hao, Y., Li, X., Wang, X., Ji, B., and Wu, Y. (2018). HDAC4 in ischemic stroke: Mechanisms and therapeutic potential. Clin. Epigenetics 10:117. doi: 10.1186/s13148-018-0549-1
Kreisel, S. H., Stroick, M., Griebe, M., Alonso, A., Reuter, B., Hennerici, M. G., et al. (2016). True Effects or Bias? MMP-2 and MMP-9 Serum Concentrations after Acute Stroke. Cerebrovasc. Dis. 42, 352–360. doi: 10.1159/000446405
Kreisel, S. H., Stroick, M., Reuter, B., Senn, E., Hennerici, M. G., and Fatar, M. (2012). MMP-2 concentrations in stroke according to etiology: Adjusting for enzyme degradation in stored deep-frozen serum and other methodological pitfalls. J. Clin. Neurosci. 19, 1564–1567. doi: 10.1016/j.jocn.2011.10.026
Langley, B., Brochier, C., and Rivieccio, M. A. (2009). Targeting histone deacetylases as a multifaceted approach to treat the diverse outcomes of stroke. Stroke 40, 2899–2905. doi: 10.1161/STROKEAHA.108.540229
Li, M., Zhang, X., Cui, L., Yang, R., Wang, L., Liu, L., et al. (2011). The neuroprotection of oxymatrine in cerebral ischemia/reperfusion is related to nuclear factor erythroid 2-related factor 2 (nrf2)-mediated antioxidant response: Role of nrf2 and hemeoxygenase-1 expression. Biol. Pharm. Bull. 34, 595–601. doi: 10.1248/bpb.34.595
Li, Q., Li, X., Tang, H., Jiang, B., Dou, Y., Gorospe, M., et al. (2017). NSUN2-Mediated m5C Methylation and METTL3/METTL14-Mediated m6A Methylation Cooperatively Enhance p21 Translation. J. Cell. Biochem. 118, 2587–2598. doi: 10.1002/jcb.25957
Li, Y., Li, H., Luo, Y., Li, X., Chen, Z., Zhang, W., et al. (2022). The alteration profiles of m(6)A-tagged circRNAs in the peri-infarct cortex after cerebral ischemia in mice. Front. Neurosci. 16:869081. doi: 10.3389/fnins.2022.869081
Lin, H. F., Hsi, E., Huang, L. C., Liao, Y. C., Juo, S. H., and Lin, R. T. (2017). Methylation in the matrix metalloproteinase-2 gene is associated with cerebral ischemic stroke. J. Investig. Med. 65, 794–799. doi: 10.1136/jim-2016-000277
Lin, R. T., Hsi, E., Lin, H. F., Liao, Y. C., Wang, Y. S., and Juo, S. H. (2014). LINE-1 methylation is associated with an increased risk of ischemic stroke in men. Curr. Neurovasc. Res. 11, 4–9. doi: 10.2174/1567202610666131202145530
Liotti, A., Ferrara, A. L., Loffredo, S., Galdiero, M. R., Varricchi, G., Di Rella, F., et al. (2022). Epigenetics: An opportunity to shape innate and adaptive immune responses. Immunology [Epub ahead of print]. doi: 10.1111/imm.13571
Liu, K., Ding, L., Li, Y., Yang, H., Zhao, C., Lei, Y., et al. (2014). Neuronal necrosis is regulated by a conserved chromatin-modifying cascade. Proc. Natl. Acad. Sci. U.S.A. 111, 13960–13965. doi: 10.1073/pnas.1413644111
Lopez-Dee, Z., Pidcock, K., and Gutierrez, L. S. (2011). Thrombospondin-1: Multiple paths to inflammation. Mediators Inflamm. 2011:296069. doi: 10.1155/2011/296069
Lu, M., Guo, J., Wu, B., Zhou, Y., Wu, M., Farzaneh, M., et al. (2021). Mesenchymal Stem Cell-Mediated Mitochondrial Transfer: A Therapeutic Approach for Ischemic Stroke. Transl. Stroke Res. 12, 212–229. doi: 10.1007/s12975-020-00853-6
Mancha, F., Escudero-Martinez, I., Zapata-Arriaza, E., Vega-Salvatierra, A., Cabezas, J. A., Lebrato, L., et al. (2020). Circulating microRNA after autologous bone marrow mononuclear cell (BM-MNC) injection in patients with ischemic stroke. J. Investig. Med. 68, 807–810. doi: 10.1136/jim-2019-001161
Manwani, B., Liu, F., Xu, Y., Persky, R., Li, J., and McCullough, L. D. (2011). Functional recovery in aging mice after experimental stroke. Brain Behav. Immun. 25, 1689–1700. doi: 10.1016/j.bbi.2011.06.015
Marquez-Romero, J. M., Reyes-Martinez, M., Huerta-Franco, M. R., Ruiz-Franco, A., Silos, H., and Arauz, A. (2020). Fluoxetine for motor recovery after acute intracerebral hemorrhage, the FMRICH trial. Clin. Neurol. Neurosurg. 190:105656. doi: 10.1016/j.clineuro.2019.105656
Meyer, K. D., Saletore, Y., Zumbo, P., Elemento, O., Mason, C. E., and Jaffrey, S. R. (2012). Comprehensive analysis of mRNA methylation reveals enrichment in 3′ UTRs and near stop codons. Cell 149, 1635–1646. doi: 10.1016/j.cell.2012.05.003
Morita, S., Horii, T., Kimura, M., Ochiya, T., Tajima, S., and Hatada, I. (2013). Mir-29 represses the activities of DNA methyltransferases and DNA demethylases. Int. J. Mol. Sci. 14, 14647–14658. doi: 10.3390/ijms140714647
Morris-Blanco, K. C., Kim, T., Lopez, M. S., Bertogliat, M. J., Chelluboina, B., and Vemuganti, R. (2019). Induction of DNA hydroxymethylation protects the brain after stroke. Stroke 50, 2513–2521. doi: 10.1161/STROKEAHA.119.025665
Nam, A. S., Chaligne, R., and Landau, D. A. (2021). Integrating genetic and non-genetic determinants of cancer evolution by single-cell multi-omics. Nat. Rev. Genet. 22, 3–18. doi: 10.1038/s41576-020-0265-5
Nesin, S. M., Sabitha, K. R., Gupta, A., and Laxmi, T. R. (2019). Constraint Induced Movement Therapy as a Rehabilitative Strategy for Ischemic Stroke-Linking Neural Plasticity with Restoration of Skilled Movements. J. Stroke Cerebrovasc. Dis. 28, 1640–1653. doi: 10.1016/j.jstrokecerebrovasdis.2019.02.028
Ng, G. Y., Lim, Y. A., Sobey, C. G., Dheen, T., Fann, D. Y., and Arumugam, T. V. (2018). Epigenetic regulation of inflammation in stroke. Ther. Adv. Neurol. Disord. 11:1756286418771815. doi: 10.1177/1756286418771815
Nieuwkamp, D. J., Setz, L. E., Algra, A., Linn, F. H., de Rooij, N. K., and Rinkel, G. J. (2009). Changes in case fatality of aneurysmal subarachnoid haemorrhage over time, according to age, sex, and region: A meta-analysis. Lancet Neurol. 8, 635–642. doi: 10.1016/S1474-4422(09)70126-7
Olmez, I., and Ozyurt, H. (2012). Reactive oxygen species and ischemic cerebrovascular disease. Neurochem. Int. 60, 208–212. doi: 10.1016/j.neuint.2011.11.009
Pan, R. Y., He, L., Zhang, J., Liu, X., Liao, Y., Gao, J., et al. (2022). Positive feedback regulation of microglial glucose metabolism by histone H4 lysine 12 lactylation in Alzheimer’s disease. Cell Metab. 34, 634–648e636. doi: 10.1016/j.cmet.2022.02.013
Pandi, G., Nakka, V. P., Dharap, A., Roopra, A., and Vemuganti, R. (2013). MicroRNA miR-29c down-regulation leading to de-repression of its target DNA methyltransferase 3a promotes ischemic brain damage. PLoS One 8:e58039. doi: 10.1371/journal.pone.0058039
Patnala, R., Arumugam, T. V., Gupta, N., and Dheen, S. T. (2017). Inhibitor sodium butyrate-mediated epigenetic regulation enhances neuroprotective function of microglia during ischemic stroke. Mol. Neurobiol. 54, 6391–6411. doi: 10.1007/s12035-016-0149-z
Peng, J., Ghosh, D., Pang, J., Zhang, L., Yin, S., and Jiang, Y. (2022). Intertwined relation between the endoplasmic reticulum and mitochondria in ischemic stroke. Oxid. Med. Cell. Longev. 2022:3335887. doi: 10.1155/2022/3335887
Putaala, J., and Nieminen, T. (2018). Stroke risk period after acute myocardial infarction revised. J. Am. Heart Assoc. 7:e011200. doi: 10.1161/JAHA.118.011200
Puy, L., Forman, R., Cordonnier, C., and Sheth, K. N. (2022). Protecting the brain, from the heart: Safely mitigating the consequences of thrombosis in intracerebral hemorrhage survivors with atrial fibrillation. Stroke 53, 2152–2160. doi: 10.1161/STROKEAHA.122.036888
Qi, X., Hosoi, T., Okuma, Y., Kaneko, M., and Nomura, Y. (2004). Sodium 4-phenylbutyrate protects against cerebral ischemic injury. Mol. Pharmacol. 66, 899–908. doi: 10.1124/mol.104.001339
Qiu, Y. M., Zhang, C. L., Chen, A. Q., Wang, H. L., Zhou, Y. F., Li, Y. N., et al. (2021). Immune cells in the bbb disruption after acute ischemic stroke: targets for immune therapy? Front. Immunol. 12:678744. doi: 10.3389/fimmu.2021.678744
Qu, J., Chen, W., Hu, R., and Feng, H. (2016). The injury and therapy of reactive oxygen species in intracerebral hemorrhage looking at mitochondria. Oxid. Med. Cell. Longev. 2016:2592935. doi: 10.1155/2016/2592935
Saini, V., Guada, L., and Yavagal, D. R. (2021). Global epidemiology of stroke and access to acute ischemic stroke interventions. Neurology 97:S6–S16. doi: 10.1212/WNL.0000000000012781
Salvi, V., Sozio, F., Sozzani, S., and Del Prete, A. (2017). Role of atypical chemokine receptors in microglial activation and polarization. Front. Aging Neurosci. 9:148. doi: 10.3389/fnagi.2017.00148
Santini, V., Melnick, A., Maciejewski, J. P., Duprez, E., Nervi, C., Cocco, L., et al. (2013). Epigenetics in focus: Pathogenesis of myelodysplastic syndromes and the role of hypomethylating agents. Crit. Rev. Oncol. Hematol. 88, 231–245. doi: 10.1016/j.critrevonc.2013.06.004
Schweizer, S., Harms, C., Lerch, H., Flynn, J., Hecht, J., Yildirim, F., et al. (2015). Inhibition of histone methyltransferases SUV39H1 and G9a leads to neuroprotection in an in vitro model of cerebral ischemia. J. Cereb. Blood Flow Metab. 35, 1640–1647. doi: 10.1038/jcbfm.2015.99
Sendinc, E., Valle-Garcia, D., Dhall, A., Chen, H., Henriques, T., Navarrete-Perea, J., et al. (2019). PCIF1 catalyzes m6Am mRNA methylation to regulate gene expression. Mol. Cell 75, 620–630e629. doi: 10.1016/j.molcel.2019.05.030
Sharifulina, S., Dzreyan, V., Guzenko, V., and Demyanenko, S. (2021). Histone methyltransferases SUV39H1 and G9a and DNA Methyltransferase DNMT1 in penumbra neurons and astrocytes after photothrombotic stroke. Int. J. Mol. Sci. 22:12483. doi: 10.3390/ijms222212483
Song, Y. S., Kim, M. S., Kim, H. A., Jung, B. I., Yang, J., Narasimhan, P., et al. (2010). Oxidative stress increases phosphorylation of IkappaB kinase-alpha by enhancing NF-kappaB-inducing kinase after transient focal cerebral ischemia. J. Cereb. Blood Flow Metab. 30, 1265–1274. doi: 10.1038/jcbfm.2010.6
Soriano-Tarraga, C., Jimenez-Conde, J., Giralt-Steinhauer, E., Mola, M., Ois, A., Rodriguez-Campello, A., et al. (2014). Global DNA methylation of ischemic stroke subtypes. PLoS One 9:e96543. doi: 10.1371/journal.pone.0096543
Sun, W. J., Li, J. H., Liu, S., Wu, J., Zhou, H., Qu, L. H., et al. (2016). RMBase: A resource for decoding the landscape of RNA modifications from high-throughput sequencing data. Nucleic Acids Res. 44:D259–D265. doi: 10.1093/nar/gkv1036
Szyf, M. (2015). Prospects for the development of epigenetic drugs for CNS conditions. Nat. Rev. Drug Discov. 14, 461–474. doi: 10.1038/nrd4580
Tang, Y., Lin, Y. H., Ni, H. Y., Dong, J., Yuan, H. J., Zhang, Y., et al. (2017). Inhibiting histone deacetylase 2 (HDAC2) promotes functional recovery from stroke. J. Am. Heart Assoc. 6:e007236. doi: 10.1161/JAHA.117.007236
Tsuboi, Y., Yamada, H., Munetsuna, E., Yamazaki, M., Mizuno, G., Murase, Y., et al. (2018). Relationship between long interspersed nuclear element-1 DNA methylation in leukocytes and dyslipidemia in the japanese general population. J. Atheroscler. Thromb. 25, 1231–1239. doi: 10.5551/jat.43570
Udali, S., Guarini, P., Moruzzi, S., Choi, S. W., and Friso, S. (2013). Cardiovascular epigenetics: From DNA methylation to microRNAs. Mol. Aspects Med. 34, 883–901. doi: 10.1016/j.mam.2012.08.001
Wan, Y., Guo, H., Shen, J., Chen, S., Li, M., Xia, Y., et al. (2022). Association between preonset anti-hypertensive treatment and intracerebral hemorrhage mortality: A cohort study From CHEERY. Front. Neurol. 13:794080. doi: 10.3389/fneur.2022.794080
Washington, C. W., Derdeyn, C. P., Dhar, R., Arias, E. J., Chicoine, M. R., Cross, D. T., et al. (2016). A Phase I proof-of-concept and safety trial of sildenafil to treat cerebral vasospasm following subarachnoid hemorrhage. J. Neurosurg. 124, 318–327. doi: 10.3171/2015.2.JNS142752
Wesselingh, R., Butzkueven, H., Buzzard, K., Tarlinton, D., O’Brien, T. J., and Monif, M. (2019). Innate immunity in the central nervous system: A missing piece of the autoimmune encephalitis puzzle? Front. Immunol. 10:2066. doi: 10.3389/fimmu.2019.02066
Witt, B. J., Brown, R. D. Jr., Jacobsen, S. J., Weston, S. A., Ballman, K. V., Meverden, R. A., et al. (2006). Ischemic stroke after heart failure: A community-based study. Am. Heart J. 152, 102–109. doi: 10.1016/j.ahj.2005.10.018
Wu, Z., Huang, K., Yu, J., Le, T., Namihira, M., Liu, Y., et al. (2012). Dnmt3a regulates both proliferation and differentiation of mouse neural stem cells. J. Neurosci. Res. 90, 1883–1891. doi: 10.1002/jnr.23077
Xu, W., Ocak, U., Gao, L., Tu, S., Lenahan, C. J., Zhang, J., et al. (2021). Selective autophagy as a therapeutic target for neurological diseases. Cell. Mol. Life Sci. 78, 1369–1392. doi: 10.1007/s00018-020-03667-9
Yamauchi, K., Nakano, Y., Imai, T., Takagi, T., Tsuruma, K., Shimazawa, M., et al. (2016). A novel nuclear factor erythroid 2-related factor 2 (Nrf2) activator RS9 attenuates brain injury after ischemia reperfusion in mice. Neuroscience 333, 302–310. doi: 10.1016/j.neuroscience.2016.07.035
Ye, F., Garton, H. J. L., Hua, Y., Keep, R. F., and Xi, G. (2021). The Role of Thrombin in Brain Injury After Hemorrhagic and Ischemic Stroke. Transl. Stroke Res. 12, 496–511. doi: 10.1007/s12975-020-00855-4
Yoon, K. J., Ringeling, F. R., Vissers, C., Jacob, F., Pokrass, M., Jimenez-Cyrus, D., et al. (2017). Temporal control of mammalian cortical neurogenesis by m(6)A methylation. Cell 171, 877–889e817. doi: 10.1016/j.cell.2017.09.003
Yu, H., Cai, Y., Zhong, A., Zhang, Y., Zhang, J., and Xu, S. (2021). The “Dialogue” between central and peripheral immunity after ischemic stroke: Focus on spleen. Front. Immunol. 12:792522. doi: 10.3389/fimmu.2021.792522
Yu, J., Qiu, Y., Yang, J., Bian, S., Chen, G., Deng, M., et al. (2016). DNMT1-PPARgamma pathway in macrophages regulates chronic inflammation and atherosclerosis development in mice. Sci. Rep. 6:30053. doi: 10.1038/srep30053
Zhang, D., Tang, Z., Huang, H., Zhou, G., Cui, C., Weng, Y., et al. (2019). Metabolic regulation of gene expression by histone lactylation. Nature 574, 575–580. doi: 10.1038/s41586-019-1678-1
Zhang, W., Chen, R., Yang, T., Xu, N., Chen, J., Gao, Y., et al. (2018). Fatty acid transporting proteins: Roles in brain development, aging, and stroke. Prostaglandins Leukot. Essent. Fatty Acids 136, 35–45. doi: 10.1016/j.plefa.2017.04.004
Zhang, X., Connelly, J., Levitan, E. S., Sun, D., and Wang, J. Q. (2021). Calcium/Calmodulin-dependent protein kinase II in cerebrovascular diseases. Transl. Stroke Res. 12, 513–529. doi: 10.1007/s12975-021-00901-9
Zhao, H., Han, Z., Ji, X., and Luo, Y. (2016). Epigenetic regulation of oxidative stress in ischemic stroke. Aging Dis. 7, 295–306. doi: 10.14336/AD.2015.1009
Zhong, W., Cheng, J., Yang, X., Liu, W., and Li, Y. (2022). Heliox preconditioning exerts neuroprotective effects on neonatal ischemia/hypoxia injury by inhibiting necroptosis induced by Ca(2+) elevation. Transl. Stroke Res. [Epub ahead of print]. doi: 10.1007/s12975-022-01021-8
Zhou, S., Cai, B., Zhang, Z., Zhang, Y., Wang, L., Liu, K., et al. (2017). CDKN2B methylation and aortic arch calcification in patients with ischemic stroke. J. Atheroscler. Thromb. 24, 609–620. doi: 10.5551/jat.36897
Zhou, S., Zhang, Y., Wang, L., Zhang, Z., Cai, B., Liu, K., et al. (2016). CDKN2B methylation is associated with carotid artery calcification in ischemic stroke patients. J. Transl. Med. 14:333. doi: 10.1186/s12967-016-1093-4
Keywords: stroke, epigenetic, histone, DNA/RNA modification, neuroinflammation
Citation: Peng J, Ghosh D, Zhang F, Yang L, Wu J, Pang J, Zhang L, Yin S and Jiang Y (2022) Advancement of epigenetics in stroke. Front. Neurosci. 16:981726. doi: 10.3389/fnins.2022.981726
Received: 30 June 2022; Accepted: 27 September 2022;
Published: 13 October 2022.
Edited by:
Sangeetha Sukumari Ramesh, Augusta University, United StatesReviewed by:
Chunli Chen, Central South University, ChinaAnuska V. Andjelkovic, University of Michigan, United States
Umut Ocak, University of Health Sciences, Turkey
Yinghua Jiang, Tulane University, United States
Weilin Xu, Zhejiang University, China
Copyright © 2022 Peng, Ghosh, Zhang, Yang, Wu, Pang, Zhang, Yin and Jiang. This is an open-access article distributed under the terms of the Creative Commons Attribution License (CC BY). The use, distribution or reproduction in other forums is permitted, provided the original author(s) and the copyright owner(s) are credited and that the original publication in this journal is cited, in accordance with accepted academic practice. No use, distribution or reproduction is permitted which does not comply with these terms.
*Correspondence: Yong Jiang, jiangyong@swmu.edu.cn; Shigang Yin, sgyin@swmu.edu.cn
†Deceased