- Department of Oncology, University of Torino, Torino, Italy
Brain metastases (BMs) represent the most frequent event during the course of Non-Small Cell Lung Cancer (NSCLC) disease. Recent advancements in the diagnostic and therapeutic procedures result in increased incidence and earlier diagnosis of BMs, with an emerging need to optimize the prognosis of these patients through the adoption of tailored treatment solutions. Nowadays a personalized and multidisciplinary approach should rely on several clinical and molecular factors like patient’s performance status, extent and location of brain involvement, extracranial disease control and the presence of any “druggable” molecular target. Radiation therapy (RT), in all its focal (radiosurgery and fractionated stereotactic radiotherapy) or extended (whole brain radiotherapy) declinations, is a cornerstone of BMs management, either alone or combined with surgery and systemic therapies. Our review aims to provide an overview of the many modern RT solutions available for the treatment of BMs from NSCLC in the different clinical scenarios (single lesion, oligo and poly-metastasis, leptomeningeal carcinomatosis). This includes a detailed review of the current standard of care in each setting, with a presentation of the literature data and of the possible technical solutions to offer a “state-of-art” treatment to these patients. In addition to the validated treatment options, we will also discuss the future perspectives on emerging RT technical strategies (e.g., hippocampal avoidance whole brain RT, simultaneous integrated boost, radiosurgery for multiple lesions), and present the innovative and promising findings regarding the combination of novel targeted agents such as tyrosine kinase inhibitors and immune checkpoint inhibitors with brain irradiation.
Introduction
Brain metastases (BMs) represents the most frequent Central Nervous System (CNS) neoplasm and Non-Small Cell Lung Cancer (NSCLC) accounts for approximately 50% of the lesions.
In NSCLC, 10-25% of patients present BMs at the time of diagnosis, and up to 50% develop them during the disease course, with increasing incidence in recent years owing to advances in diagnostic and therapeutic procedures (1, 2).
Globally, the prognosis in this setting remains severe (inferior to 3 months without any treatments), and the prognostic stratification of these patients is crucial for an optimal management (3).
In the last 20 years we observed the creation and evolution of different prognostic scoring systems, aiming to guide clinicians to offer tailored treatments (4–7).
Starting from the Recursive Partitioning Analysis (RPA), based on age, Karnosfky Performance Status (KPS), control of the primary tumor and presence of extracranial metastases, in 2008 Sperduto et al. proposed the Graded Prognostic Assessment (GPA) which considers also the number of BMs (4, 5). Finally, the adoption of NSCLC-specific prognostic indices, integrated with molecular data, defined the modern (GPA) for Lung Cancer Using Molecular Markers (Lung-molGPA) (6, 7).
In the era of precision medicine, radiation therapy (RT) still represents a cornerstone of BMs management, and the two major radiotherapeutic options, whole brain radiotherapy (WBRT) and focal radiotherapy, are perfectly integrated with surgery and systemic therapies in a multimodal approach (8). Given the detrimental effect of BMs on the prognosis of NSCLC patients, the selection of the appropriate treatment on a case-by-case judgment is of pivotal importance to obtain the remission of pre-existing BMs, to prevent the development of new lesions and to possibly improve the final outcome.
Our review provides an overview of the actual indications to RT treatments in the management of BMs from NSCLC in the different clinical scenarios (single lesion, oligometastatic disease, polymetastatic disease, leptomeningeal carcinomatosis and prophylactic cranial irradiation (PCI) in locally advanced disease), with a secondary focus on future perspectives on emerging technical RT solutions and their possible combinations with novel targeted agents.
Single Lesion
Single BM represents the most favorable disease presentation in the setting of BMs.
Several randomized controlled trials (RCTs) demonstrated that the radical management of the single lesion with focal treatments, either surgery or RT, in addition to the historical WBRT approach, improves both local control (LC) and overall survival (OS) (9–11).
Surgery is the gold standard treatment for large, edemigenous lesions, allowing an immediate symptoms relief and, when necessary, a histological determination (12). In resected patients, RT is universally adopted as adjuvant therapy to reduce the risk of local relapse.
Radiosurgery (SRS), a highly conformal technique delivering high doses of radiation in a single fraction, represents the best solution for small lesions and a valid option for larger lesions when surgery is not feasible (generally for comorbidities or involvement of brain areas with high risk of post-surgical sequelae).
SRS can be delivered with different technical solutions such as Gamma-knife, Cyber-knife, LINAC-based SRS and none of these have been shown to be superior (13).
Upfront Focal RT
The potential of SRS as an alternative focal strategy to surgery was initially described with case reports between the 1980s and 1990s (14, 15).
The first robust evidence came from the RTOG 9508 trial. In this multi-institutional study, 333 patients with 1 to 3 BMs were randomized to receive WBRT or WBRT + SRS boost; 186 patients (56% of the total) had single brain lesion. In the overall population, the addition of SRS achieved an advantage in LC (1-y LC: 81% WBRT+SRS vs 71% WBRT alone, p=0.01) without any survival benefit. After stratification for the number of brain lesions, patients with a single BM had a superior median OS with the addition of SRS (6.5 vs 4.9 months, p=0.039) (11).
The next generation of RCTs strengthened the role of upfront SRS, proposing the omission of WBRT in patients with oligometastatic disease (1-4 lesions) (16–19). Approximately 50% of the enrolled population of these studies had only a single lesion (range 48%-67%). The results, despite the different primary endpoints considered, were univocal and consistent with the surgical series (20, 21), showing better intracranial disease control in patients who received additional whole brain irradiation, but without a translation into a survival advantage. At the same time, WBRT negatively influenced some aspects of the quality of life (QoL) and the neurocognitive functions of these patients (16, 17, 19, 22). All the historical RCTs considered so far proposed a single-high-dose fraction for lesions with a maximum diameter of less than 3-4 cm (11, 16–19).
The maximum tolerated single fraction dose was established with a risk adapted approach in the RTOG 9005 phase I dose escalation trial. Maximum tolerated doses were 24 Gy, 18 Gy, and 15 Gy for tumors < 20 mm, 21–30 mm, and 31–40 mm in maximum diameter, respectively (23, 24).
This risk adaptive approach is currently adopted in clinical practice, although for larger lesions (>2 cm) 18-15 Gy or less in single fraction could be detrimental in terms of LC. On the other hand, greater RT doses could lead to an excessive risk of complications, in particular radionecrosis (RN).
RN represents the major complication of SRS, with a highly variable incidence rate in the literature (range 5-25%) (25). The time to occurrence varies from few months to several years after radiation, but approximately 80% of cases occur within 3 years (26). The etiology is multifactorial, mainly related to the exposure of a significant volume of healthy brain tissue to high doses of radiation, previous brain irradiation, concomitant systemic therapies and some specific histologies (27). The radiological aspect is characterized by enhancing lesions and/or rounded presentations with intra-lesional areas of necrosis in the previously irradiated brain tissue. Standard neuroimaging may be inadequate to distinguish RN from tumor progression, requiring more advanced Magnetic Resonance Imaging (MRI) sequences and functional imaging (25). Figure 1 shows a typical sequence of MR findings of RN occurred after SRS. The therapeutic approach, particularly relevant in highly symptomatic presentations, ranges from the classic steroid therapy to the use of bevacizumab, up to the need for surgical resection for large, edemigenous lesions (28).
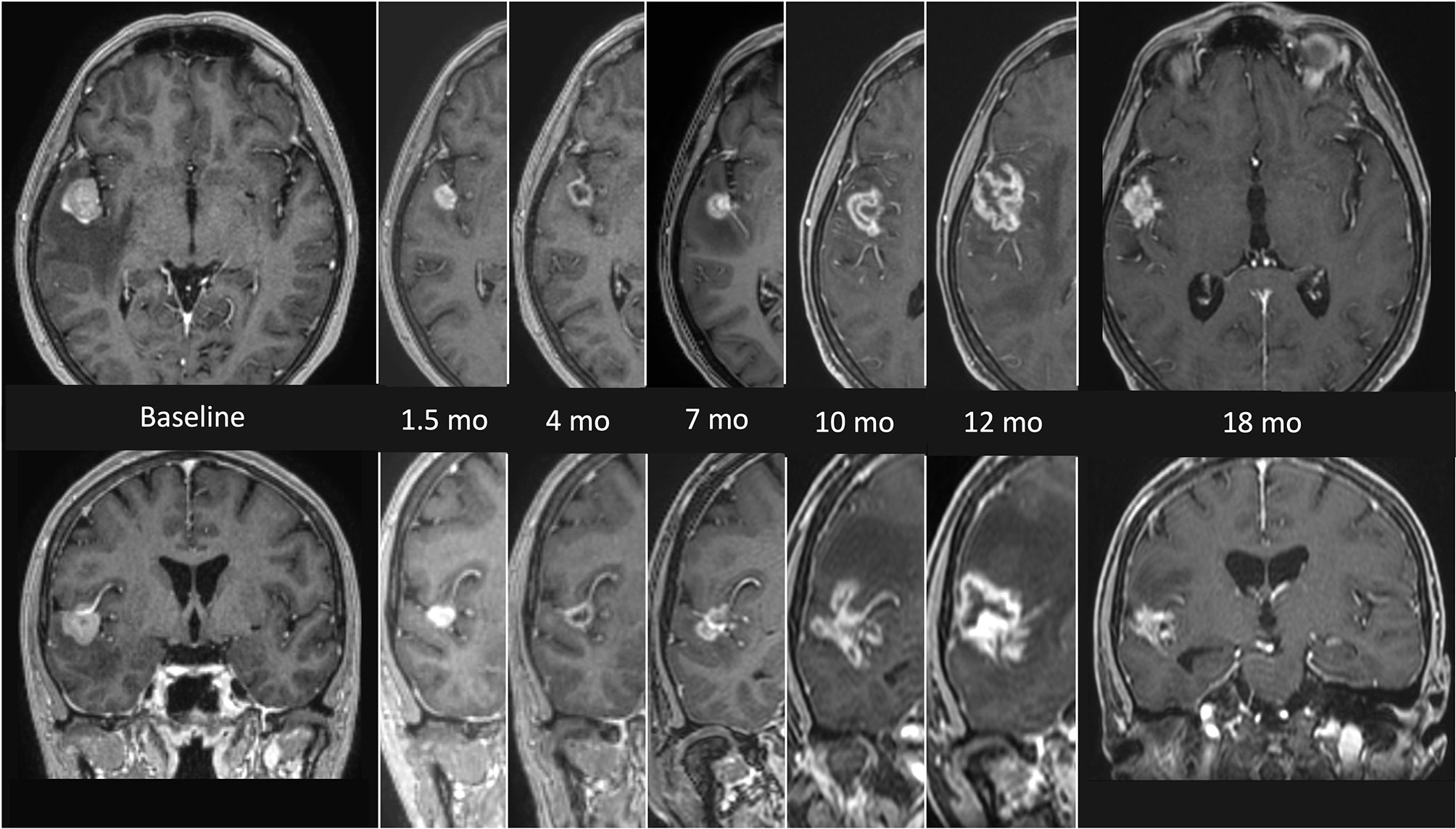
Figure 1 MRI findings presenting the evolution of radionecrosis (RN) within a timeframe of 18 months from the end of radiosurgery (SRS) in a single right temporal BM from NSCLC. The first images on the left (axial and coronal) represent the lesion before focal treatment. Serial follow up images show an initial shrinkage of the treated lesion followed by a significant and continuous increase of the necrotic component. The latest images on the right (axial and coronal) refers to the situation 18 months after SRS, with a clear reduction of the necrotic component, which allows a differential diagnosis with a relapse.
The ideal solution to improve LC without increasing the risk of RN related to focal RT is represented by (hypo-)fractionated stereotactic RT (SRT). By delivering a lower dose per fraction in few (generally ≤ 5) sessions, it is possible to achieve higher biologically effective doses (BED) to the tumor with a lower radiobiological effect to the surrounding brain tissue. This approach became possible as a result of the introduction of Image-Guided RT (IGRT), which enables to deliver high doses of radiation without stereotactic invasive headframe (frameless SRT). Retrospective series of large brain lesions treated with SRT accumulated over the years, providing better rates of LC (1-y LC: 79.2%-92.9%) and RN (RN rates: 6.5%-7.3%) compared with SRS (29).
Data on Tumor Control Probability (TCP), considered as the probability of LC (30) and Normal Tissue Complication Probability (NTCP), considered as the risk of RN (31), were recently published as Organ-Specific Papers from the international collaborative project “HYTEC” (Hypofractionated Treatment Effects in the Clinic), to guide dose and fractionation choices for SRS/SRT in BMs. The authors concluded that single-fraction SRS with doses of 18-24 Gy should be the first choice for tumors ≤ 2 cm, guaranteeing an estimated LC of 85%-95% at 12 months, while SRT should be preferred for lesion >2 cm (30). The risk of RN is modeled on dose/volume parameters. For SRS, the volume of healthy brain tissue receiving 12 Gy (V12) is a strong predictor of symptomatic RN, with a risk lowered to 10% when less than 5 cm3 are exposed to that threshold dose. For SRT, the volume of healthy tissues that might be exposed to high radiation doses is even larger, thus representing the best schedule for bigger lesions (31). Ongoing trials, reported in Table 1, will provide further evidences. The choice of the focal strategy between surgery and SRS in resectable lesions deserves further considerations. To date, there is no evidence from randomized trials directly comparing the two treatment modalities.
Two attempts of RCT between SRS and surgery were stopped early for slow accrual: Muacevic et al. randomized 64 patients with a single, small sized (≤3 cm) resectable BM to receive surgery + WBRT vs SRS alone (32); Roos et al. randomized 21 patients with a solitary BM to SRS vs surgery, both with adjuvant WBRT (33).
A Cochrane review of 2018 tried to pool the 85 patients from these two studies, but a meta-analysis was not possible due to clinical heterogeneity between trial interventions (34). More recent studies compared the efficacy of SRS and surgery as single treatment modalities, as per modern trend to omit WBRT in patients with a limited number of BMs. Quigley et al. compared 162 consecutive patients (46% single lesions) that received surgery + SRS boost (49 patients) vs SRS alone (113 patients). Surgery + SRS boost resulted in greater local control and survival when complete resection was achieved (35).
Recently a secondary analysis of the EORTC 22952-26001 trial investigated for any difference in terms of LC among brain oligometastatic patients treated with SRS (154 patients) or surgery (114 patients). After adjustments for site, size, number of lesions, neurological status and extracranial disease, a similar LC resulted between the two groups. Surgically treated patients experienced a higher rate of early (0-3 months) local recurrence, but the relative benefit of SRS decreased with time (36).
Conclusions: SRS represents the best focal approach for small or unresectable single brain lesions. Surgery or SRT should be preferred when treating lesions with a larger diameter >2 cm. The adoption of SRT provides a better LC with a low risk of RN when treating larger lesions.
RT Complementary to Surgery
Adjuvant WBRT
Adjuvant WBRT has been considered the standard of care after surgery for many decades, with the rationale of improving both local surgical cavity and distant intracranial control. More recently, the advent of highly sensitive neuroimaging, such as multi-parametric brain MRI, made it reasonable to omit adjuvant WBRT, and its related early (hair loss and fatigue) and late (neurocognitive deterioration) complications, when a complete resection is achieved.
Some multicenter RCTs were conducted to investigate the role of WBRT in this setting.
The first one by Patchell and colleagues (20) enrolled 95 patients (60% with a primary diagnosis of NSCLC), receiving a complete-surgical resection of a single BM. WBRT resulted in better intracranial tumor control compared to observation (anywhere recurrence: 18% vs 70%, p<0.001). Furthermore, WBRT determined less neurological deaths (14% adjuvant WBRT vs 44% surgery alone, p=0.03) but no significant differences in functional independence and OS (approximately 10 months in both arms) were observed.
The EORTC 22952-26001 study investigated the role of adjuvant WBRT after surgery or SRS for 1-3 BMs, randomizing patients to WBRT or observation: 359 patients (NSCLC was the primary tumor in 53%) with a limited number of BMs (1–3) were randomized to receive WBRT or to close observation after local therapy, either surgery or SRS. In the surgery group (160 patients) almost all the patients had a single (96%), large (median size 30 mm) metastasis. For these patients, postoperative WBRT significantly reduced the probability of local relapse by 32% (from 59% to 27%, p <0.001) and the probability of distant intracranial relapse by 19% (from 42% to 23%, p=0.001). Again, no difference was found in functional independence and OS (19).
More recently, the Japanese JCOG0504 non-inferiority trial randomly assigned to WBRT or observation and salvage SRS 271 patients (47% diagnosed with NSCLC) with 4 or fewer surgically resected BMs (73% single lesion).
Observation with salvage SRS was not inferior to WBRT in terms of OS (median OS: 15.6 months in both arms, p=0.027), although median intracranial Progression Free Survival (PFS) was shorter (10.4 months for WBRT vs 4 months for salvage SRS). In addition, a deferred brain irradiation with a highly conformal technique resulted in more than half reduction of severe cognitive deterioration for salvage SRS patients (G2-G4 cognitive dysfunction: 16.4% WBRT vs 7.7% salvage SRS) (21).
Conclusions: WBRT is usually avoided as adjuvant treatment, considering the absence of survival benefit and the severe impact on neurocognitive functions. Anyway, it remains the best strategy to prevent the onset of new BMs.
Adjuvant SRS/SRT
Given the suboptimal LC of surgery alone, with an unacceptable high risk of local recurrence (around 50%) (19, 20), and the inescapable severe neurocognitive sequelae of whole brain irradiation, the modern trend is to offer focal postoperative RT to significantly improve the remission rate of the surgical cavity. In the last two decades, several retrospective studies investigated the role of adjuvant SRS to the surgical cavity and a recent meta-analysis, including 3458 patients from 50 studies, showed high LC (1-y LC: 83.7%) and low toxicity profile (RN rate: 6.9%) (37).
Two randomized trials confirmed the efficacy and safety of this treatment. In the study by Mahajan and colleagues (38), the 12-months freedom from local recurrence was 43% in the observation group and 72% in the SRS group (p=0.015), but with no difference in OS. In the NCCTG N107C/CEC.3 multicenter trial 194 patients (59% NSCLC) were randomly assigned to adjuvant SRS or WBRT, with the latter one providing a better time to intracranial tumor progression (median time: 27.5 months vs 6.4 months, p<0.0001), considering both local and distant recurrence. On the other hand, neurocognitive preservation was significantly higher in the SRS group (median cognitive-deterioration-free survival: 3.7 months vs 3 months, p<0.0001) with significant difference in terms of immediate and delayed memory, processing speed and executive function (39). As predictable, OS was comparable between the two arms (12.2 months for SRS vs 11.6 months for WBRT, p=0.70).
Further evidence from the ESTRON German trial (NCT03285932), presenting the same interventional arms, are awaited to provide more robust evidence on the role of SRS in the adjuvant setting after the resection of BMs (40). Although the availability of high quality data in support of the clinical role of adjuvant SRS to the surgical cavity, some practical and technical challenges remain to be solved. First, the optimal dose and fractionation to obtain the ideal balance between LC and risk of RN. Considering that surgery is generally performed for large, symptomatic lesions, it is very common for the treating radiation oncologist to face with large post-operative volumes to be irradiated. The considerations for cavities greater than 2-3 cm in diameter are the same as for large unresected BMs, with SRT representing the best solution. To date, several retrospective and meta-analysis data are available and confirm an excellent risk-benefit balance of focal SRT to the resection cavity (41–45), with a trend for better LC and lower rates of RN compared to SRS (29, 37). The prescribed dose of SRT usually ranges 24-30 Gy in 3-5 fractions in the published series (41, 46). Based on the available data, the HYTEC TCP and NTCP papers provide useful indications on doses and constraints to orientate in the clinical practice, as previously done for the radical and upfront setting (30, 31). The validation of these results in a randomized setting are awaited from the ongoing Alliance trial (NCT04114981), which is randomizing ≥2 cm-sized-operated brain lesions with post-surgical cavities smaller than 5 cm to be irradiated with single fraction SRS or 3-5 fractions SRT (Table 1).
The second issue is related to the uncertainties in the target delineation. The dynamic adaptation of the surgical cavity and of the surrounding tissues after surgical resection, cause significant changes in the shape of the target area, with an average cavity/volume reduction after surgery estimated in a range of 15-43% (47). This volume shrinkage and the timing needed to obtain a reasonable post-surgical clinical recovery, influence the timing for the administrations of adjuvant RT, generally performed within maximum 4-6 weeks after surgery.
In 2017 a consensus contouring guideline for adjuvant SRS in completely resected BM cavity was generated, with the aim of standardizing this highly variable procedure. This paper recommended to use pre- and post-operative contrast-enhancing T1-weighted MRI to guide the contouring process and to include in the clinical target volume (CTV) the entire surgical cavity as well as the surgical tract, with the inclusion of any site of preoperative dural or venous sinus involvement (48).
The last important consideration on post-surgical SRS/SRT is about the risk of leptomeningeal dissemination (LMD). Surgical procedures may disseminate cells in surrounding tissues causing the neoplastic seeding of the cerebrospinal fluid, and focal adjuvant RT seems to be less effective than whole-brain irradiation to prevent this complication. In retrospective series LMD incidence ranges from 8 to 13% (49, 50). In the “Leptomeningeal Metastasis” section this scenario will be further explored.
Conclusions: focal RT is currently the preferred adjuvant treatment after surgical resection of BM. The excellent LC and a good toxicity profile make this strategy preferable to WBRT. The ideal timing, doses, fractionations and volumes for post-operative stereotactic irradiation are currently under investigation.
Neoadjuvant SRS
The new frontier in the treatment of single or few BMs is preoperative SRS. The rational is to sterilize any microscopic disease before the macroscopic resection of a brain lesion and to prevent all critical issues related to adjuvant SRS. Generally, neoadjuvant RT is performed as a single fraction of 16 Gy, given a few days before surgery.
Neoadjuvant SRS allows a better definition of the target, with a potentially reduced risk of LMD (sterilizing microscopic disease) and RN compared to the adjuvant setting. Figures 2, 3 illustrate volume and dose-volume histogram comparison between neoadjuvant and adjuvant focal RT.
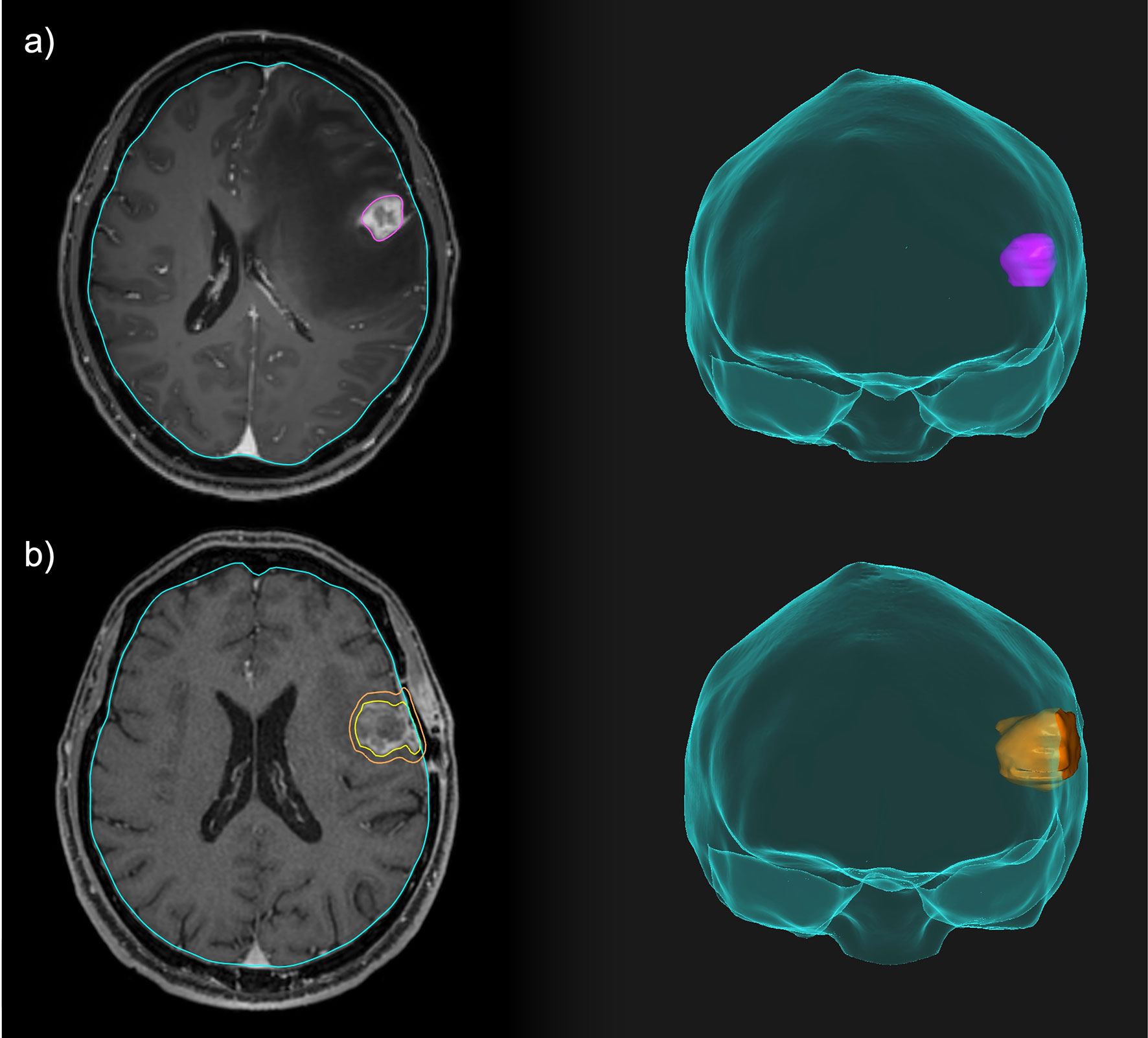
Figure 2 Comparison between neoadjuvant and adjuvant focal RT for a resectable brain lesion. (A) Pre-operative treatment volume including only the macroscopic disease. (B) Post-operative treatment volume including the resection cavity, the surgical tract and a 3-mm expansion margin.
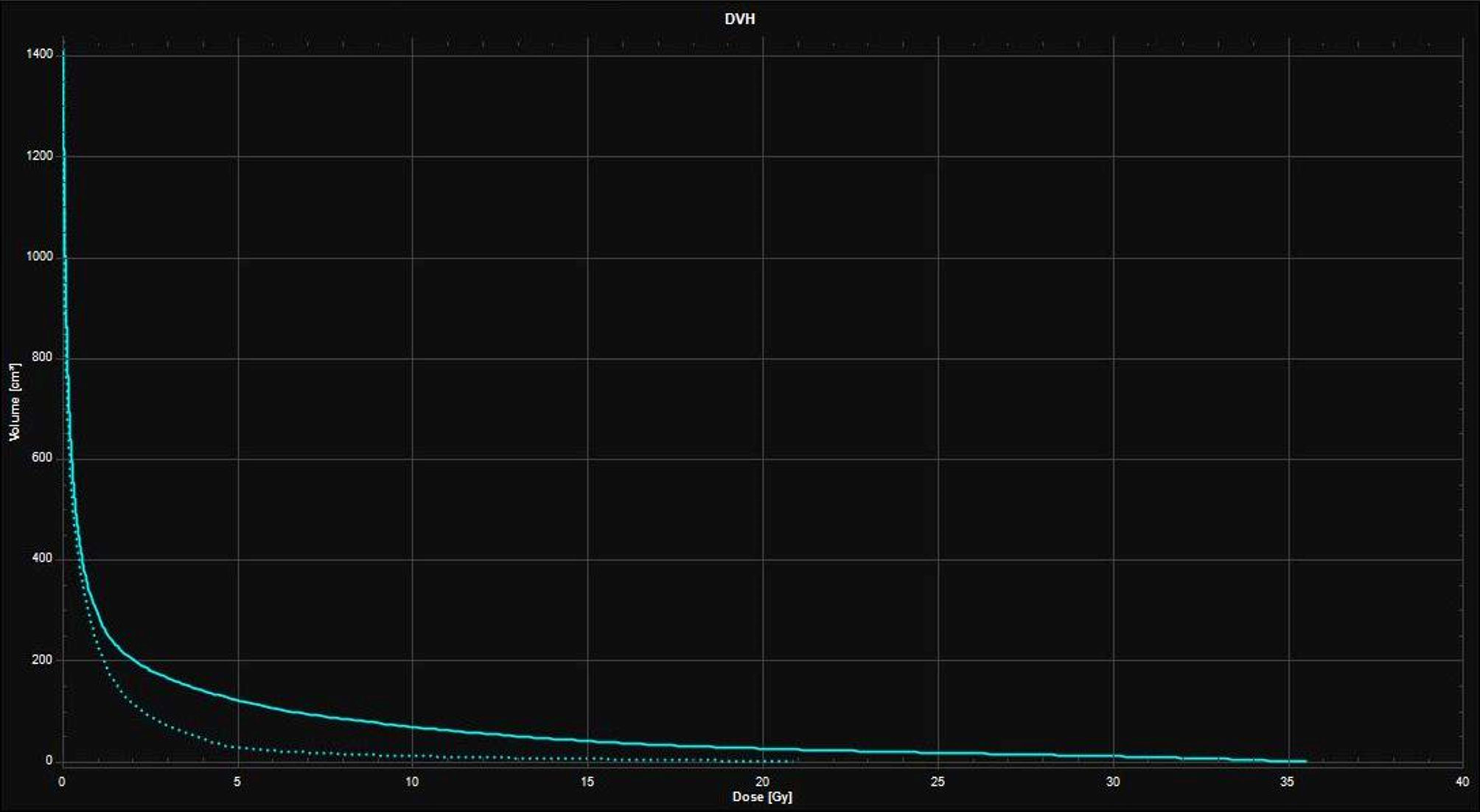
Figure 3 Dose-Volume Histograms (DVH) showing the lower dose received by the healthy brain with the 16 Gy neoadjuvant SRS treatment plan (dotted line) compared to the 27 Gy in 3 fractions adjuvant SRT treatment plan (continuous line).
Several single arm studies have collected retrospective and prospective data on neoadjuvant SRS, showing interesting efficacy and toxicity profile (including low rates of RN and LMD), but the small sample sizes are still inadequate to provide a robust evidence (51–56).
The recent PROPS-BM, a multicenter cohort study from 5 American institutions, represents the largest series of patients treated with neoadjuvant SRS to date. The outcomes of 242 patients (43.4% diagnosed with NSCLC) with 253 lesions (62.4% had a single BM) were analyzed. With a median time between SRS and surgery of 1 day (range 1-3 days), the median prescribed dose at the 80% isodose line was 15 Gy to a median GTV of 9.9 cm3. The treatment was effective (1-year LC: 85%; median survival time 16.9 months; LMD rate: 7.9%) and safe (postoperative complications: 7%; RN rate: 7.1%), with subtotal resection as strong independent predictor for local recurrence (57).
Ongoing studies, particularly the phase III trial from MD Anderson Cancer Centre (MDACC) (NCT03741673), Mayo Clinic (NCT03750227) and Alberta (NCT04474925), will provide further information on this promising approach (Table 1).
Conclusions: For resectable BMs neoadjuvant SRS is a promising approach, with potential advantages if compared to adjuvant RT. Further evidence is needed for a greater implementation of this treatment into clinical practice.
Oligometastases (2-4 LESIONS)
The oligometastatic brain disease represents a stage with limited number (usually up to 3-4) of BMs, expression of an intermediate status between the absence of brain lesions and a disseminated disease.
The distinction between oligo and polymetastic disease arises from the need for a simple system to categorize patients with BMs based on the number of lesions, in order to determine the appropriate therapeutic strategy. It is based on an empirical cut-off, extrapolated from the inclusion criteria of the main RCTs.
In this setting focal therapies determine excellent LC rates, without the same survival rates observed for the single lesion (9–11). Focal SRS represents the best solution to treat all the metastatic spots and to obtain the best oncological and clinical outcomes. Surgery plays a secondary role, and is usually limited to the resection of large and symptomatic lesions.
Historical data from the randomized RTOG 9508 trial showed a significant improvement in LC at 1 year (81% vs 71%, p=0.01) with the addition of SRS to WBRT in patients with 2-3 brain lesions, but OS did not change among the two groups. As an additional finding, patients with good prognosis (RPA I) showed a survival benefit with the combination of WBRT and SRS regardless of the number of lesions (11).
SRS Alone With WBRT Omission
The demonstrated local efficacy of focal SRS has raised the question on the feasibility of omitting WBRT after SRS not only in patients with a single lesion but also in those with a limited number (2–4) of BMs, in order to keep WBRT as a salvage option and to delay or possibly avoid neurocognitive dysfunctions.
Four historical randomized trials and an individual patient data meta-analysis investigated this scenario with different endpoints (16–19, 22).
Overall, WBRT demonstrated an increase in local and distant intracranial control (by approximately 15-30% and 50% respectively), but without any survival benefit and at the price of a worsening in neurocognitive functions and QoL. These results generated a new trend to omit WBRT also in patients with few BMs, despite some controversial aspects.
In fact, it is known that intracranial relapse could be the primary cause of death in these patients, and WBRT has proved to be an effective strategy to achieve both local and distant intracranial control. At the same time, the progression of metastatic brain disease involving critical areas can cause rapid neurocognitive deterioration. The correct patient stratification, according to modern prognostic scores, could help to identify those patients that could benefit from the combination of WBRT and SRS in this peculiar setting.
With this purpose, 3 secondary analyses of the JROSG (58), EORTC (59) and Alliance (60) trials were conducted, post-stratifying the NSCLC populations according to the Disease Specific-GPA (DS-GPA). Among the 88 NSCLC patients of the Japanese study, better OS was observed with SRS + WBRT in the 47 with DS-GPA 2.5-4.0 (WBRT+SRS: 16.7 months vs SRS: 10.6 months, p = 0.04). No difference was observed in the unfavorable DS-GPA group (0.5-2.0), probably due to a less significant impact of intracranial control on patient’s prognosis (HR: 3.57 p =0.04 vs HR: 8.31 P < 0.001) (58). By contrast, the other two exploratory analyses of the EORTC and Alliance trials, did not demonstrate a survival benefit in favor of the addition of WBRT in any risk category according to DS-GPA (59, 60). Table 2 resumes these findings.
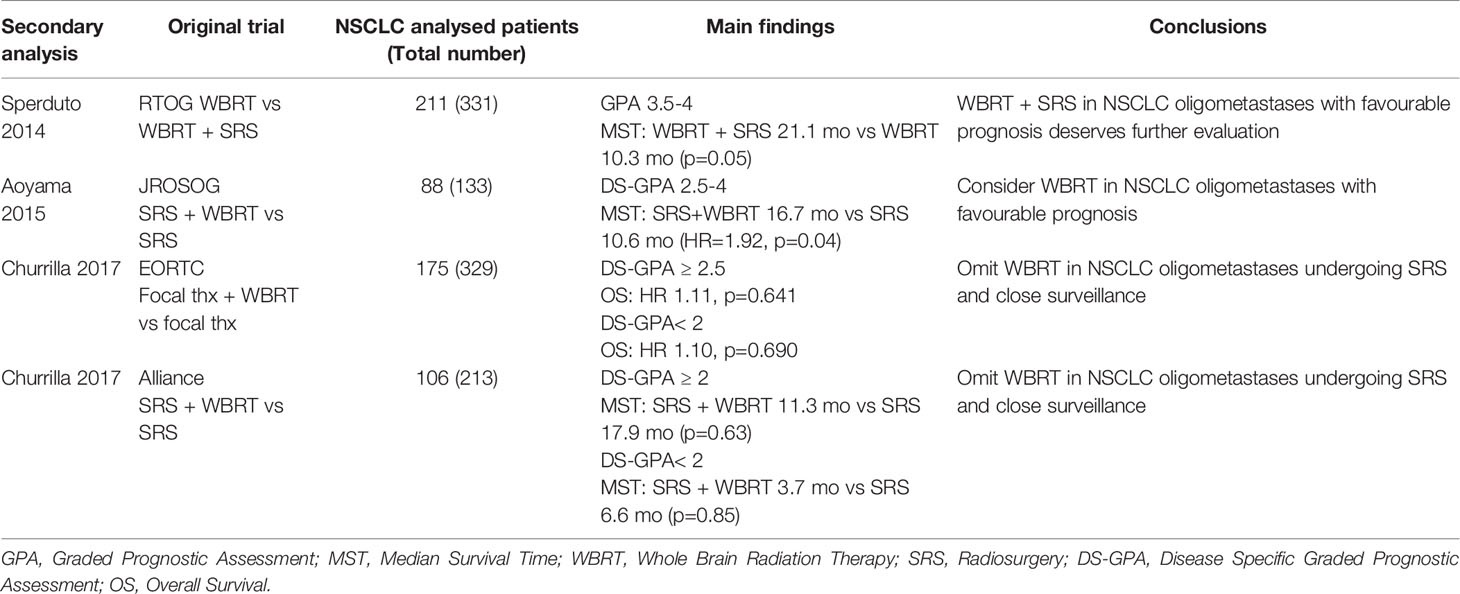
Table 2 Secondary analyses of RCT post-stratifing NSCLC patients with oligometastases to the brain (1-3 or 4 lesions) according to DS-GPA.
Conclusions: SRS alone could be safely administered to patients with 2-4 BMs. The omission of WBRT preserves the neurocognitive functions without compromising survival in the overall NSCLC population. Some secondary analyses suggest a potential survival advantage combining WBRT and SRS in NSCLC patients with 2-4 brain lesions and a favorable GPA score.
Systemic Therapy in Oligometastatic Disease
Historically, systemic therapy played a secondary role in the management of BMs, considering the CNS as a “sanctuary site” for traditional chemotherapy (ChT) due to the blood brain barrier.
In NSCLC, the development of immune checkpoint inhibitors (ICIs) and targeted agents [particularly Epidermal Growth Factor Receptor (EGFR) and Anaplastic Lymphoma Kinase (ALK) Tyrosine Kinase Inhibitors (TKIs)], redesigned this scenario, for the ability of these novel drugs to permeate in the CNS. The new generation of targeted agents, such as osimertinib for EGFR-mutated (61, 62) and alectinib or lorlatinib for ALK-rearranged (63, 64) NSCLC, significantly improved intracranial response rate compared to traditional ChT or first-generation TKIs, with responses durable over time.
Today, these drugs may be integrated with focal therapies in a multimodal approach. The best sequence of treatment is not clear, and the choice must be individualized according to different clinical aspects, such as the time of BMs onset (synchronous vs metachronous), the time to previous treatments for oligoprogressive or oligorecurrent disease and the presence of neurologic symptoms. In patients with NSCLC and synchronous brain oligometastases, particularly with asymptomatic presentations, upfront systemic therapy is one of the most adopted strategy, deferring focal RT upon evaluation of clinical and radiological response.
In 2015 a Korean phase III trial evaluated the role of upfront ChT in de-novo oligometastatic disease from NSCLC. This study randomized 105 patients with 1-4 asymptomatic BMs to receive SRS + ChT vs ChT alone. Upfront ChT alone resulted in good response rates (37%) with no difference in intracranial control (median time: SRS 9.4 months vs ChT 6.6 months, p=0.248) and OS (SRS 14.6 months vs ChT 15.3 months, p=0.418) between the two arms (65). Similar studies with the use of new generation TKIs are expected to even improve these outcomes.
Another solution is the combined administration of SRS and targeted treatments. Data from retrospective series in oncogene-addicted NSCLC, preliminarily showed promising results in favor of the synergistic effect of this regimen in improving the intracranial disease control (66, 67) and this approach is under investigation in ongoing RCTs.
In patients with oligorecurrent or oligoprogressive NSCLC presenting metachronous BMs, the focal approach with SRS is even helpful to delay the start of a systemic treatment or the switch to a new regimen (66).
Conclusions: Upfront systemic therapy with the delay of RT administration is a viable option for asymptomatic synchronous brain oligometastases, particularly interesting in oncogene-addicted NSCLC. Conversely, focal RT can be the way to delay systemic treatment in case of metachronous oligorecurrence or oligoprogression to the brain.
Polymetastatic Disease
The term polymetastatic refers to a clinical condition characterized by a significant number of BMs originating from a primary tumor mainly via the hematogenous route, which exposes the whole brain to the risk of micrometastatic disease. Nearly all published studies on polymetastatic disease have inclusion criteria which allow the enrolment of patients with >5 metastases. In this setting besides the number of BMs, other factors must be taken into account: age and performance status of the patient, volume of intracranial disease, histology, molecular biology, rate of progression of extracranial tumor burden (68). Current guidelines still maintain WBRT as the gold standard for the treatment of multiple symptomatic BMs; it is also strongly recommended in all situations in which the main objective is to prevent the onset of new metastases (69). WBRT was shown to improve neurological symptoms and function with minimal morbidity in this setting (70). It palliates symptoms, significantly improves intracranial control, and reduces the risk of neurological death (58, 71). In historical studies, WBRT increased median OS up to 3 to 6 months if compared to simple observation (70, 72) with an Overall Response Rate (ORR) ranging from 64% to 85% (70, 73).
In NSCLC patients with less than 3 months life expectancy and/or poor performance status, optimal supportive care (OSC) with corticosteroids is a reasonable alternative compared to WBRT as revealed by the phase III non-inferiority Medical Research Council trial (QUARTZ). This study compared OSC alone and OSC plus WBRT in NSCLC patients with BMs not amenable to surgery or SRS, reporting similar QoL at 4-, 8- and 12-weeks and OS (HR 1.06, p=0.8084) in the two arms. The median survival was 9.2 weeks for patients who received OSC plus WBRT and 8.5 weeks for patients who received OSC alone. Anyway, after stratification for prognostic factors, patients aged younger than 60 years were shown to have a better OS with WBRT (p=0.0062). A similar trend was observed for patients with ≥ 5 metastases, good GPA class and KPS ≥ 70 (74).
WBRT is currently the most widely used option in clinical practice worldwide for multiple BMs (69, 75). From a technical point of view WBRT is a relatively simple RT technique (opposed laterals fields) which involves the irradiation of the whole brain and of the meninges. Moderately hypofractionated schedules are employed in order to reduce overall treatment time and to improve patients’ compliance. The WBRT prescription of 30 Gy in 10 fractions is universally accepted; other schedules include 20 Gy/5 fx, 37.5 Gy/15 fx and 40 Gy/20 fx.
Cognitive deterioration is a major complication of WBRT, with severe dementia that can appear several months to years following cranial irradiation. However, according to recent clinical evidence neurocognitive impairment may arise early on, with a component of short-term neurocognitive decline that may occurs within the first 1-4 months (17, 76, 77).
The following section will provide an outline of currently available therapeutic options and future perspectives aiming to reduce or prevent these complications and to simultaneously increase local tumor control.
How to Limit Neurocognitive Impairment
Neuroprotectors
The irradiation of the brain is associated with a dose-dependent radiation-induced leukoencephalopathy as a result of demyelination, vascular compromise and direct damage to neurons (78, 79). Patients affected by leukoencephalopathy may develop some degree of cognitive dysfunction which can compromise the QoL and affect memory, executive function, attention and concentration, and could lead to learning disorders and dementia (80). This entity is associated with diffuse supra-tentorial white matter abnormalities and cerebral atrophy. Apart from leukoencephalopathy, cranial irradiation can damage the hippocampus which has a fundamental role in the memory function.
The use of a tumor-selective agents that enhances the effects of radiation in tumors but spares normal brain tissue might extend the therapeutic ratio of WBRT, improving LC without increasing radiation toxicity.Certain agents target tumors selectively, generating reactive oxygen species intracellularly and lowering the apoptotic threshold to radiation and chemotherapy (81).
One of the most accredited theories suggests vascular damage and mineralizing microangiopathy, with subsequent small vessel insufficiency and infarction as it is seen in vascular dementia, as the leading cause of RT-related neurotoxicity (82, 83). Therefore, neurotransmitter regulators commonly used to treat vascular dementia such as memantine have also been taken into consideration. In the RTOG 0614, a placebo double-blind RCT, the use of daily memantine for 24 weeks during and after WBRT resulted in better cognitive function over time, delayed time to cognitive and memory decline, executive function, and processing speed compared to placebo (84).
A recent drug, donepezil (an acetylcholinesterase inhibitor), is being tested in a phase 3 study among long-term adult brain tumor survivors after a course of fractionated WBRT or PCI, with the aim of improving cognitive impairment associated with brain cancer and its treatments. Despite modest improvements in several key cognitive functions, especially among patients with greater pre-treatment cognitive impairment, treatments with donepezil did not significantly improve the overall composite score (85).
The addition of Motexafin Gadolinium (MGd) to WBRT did not produce a significant overall improvement compared to WBRT alone (86). Anyway, in the intent-to-treat analysis, MGd exhibited a favourable trend in neurologic outcomes, significantly prolonging the interval to neurologic progression in NSCLC patients with BMs receiving prompt WBRT (87). Other molecules have been tested but promising results have been obtained for other histologies (88) and not for NSCLC.
Preliminary studies have demonstrated that BMX-001 provides protection of normal tissues from radiation-induced injury. Ongoing clinical trial (NCT03608020) will provide information on safety, tolerability and neurocognitive preservation of this drug.
Conclusions: Of the several molecules available to limit neurocognitive toxicity, only memantine prolongs time to neurocognitive and neurologic progression in patients with BMs from NSCLC.
Hippocampal Avoiding-WBRT (HA-WBRT)
As anticipated, the hippocampus has an important role in learning, memory and mood regulation (89). Preclinical studies have demonstrated that relatively modest doses of radiation cause an early and significant decline in neurogenesis in the subgranular zone of hippocampi associated with suppression of new memory formation and impaired recall (90). In addition, recent clinical studies have observed a dose-response related risk of postradiotherapy decline in learning delayed recall caused by the dose of radiation absorbed by the hippocampus (91, 92). More in general, cranial irradiation plays a crucial role for memory decline. Memory function, specifically recall and delayed recall, as assessed with the Hopkins Verbal Learning Test Revised (HVLT- R), have a statistically significant neurocognitive decline at 4 and 6 months from WBRT (17), with a simultaneous impairment of patient-reported QoL (93).
Recent technological improvements in radiotherapy, including helical tomotherapy, LINAC-based IMRT or volumetric-modulated arc therapy (VMAT), may be adopted to selectively spare anatomical structures involved in memory and learning during cranial irradiation (Figure 4) (92, 94).
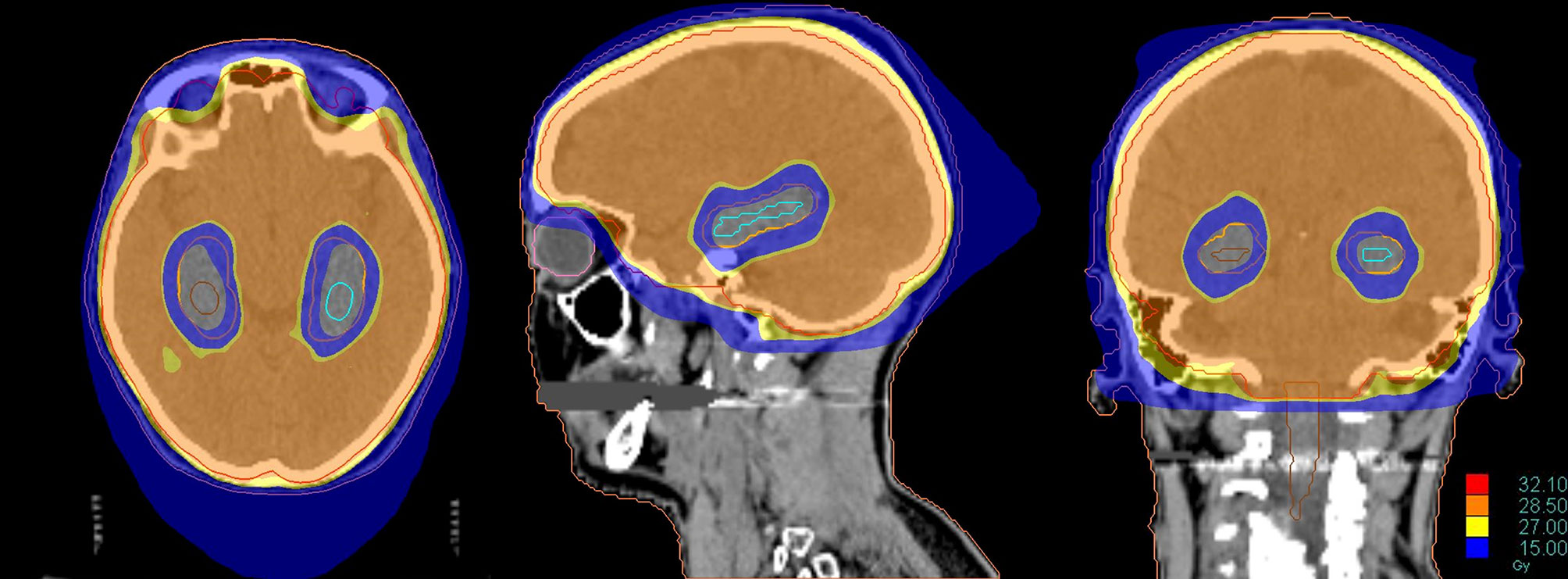
Figure 4 Dose distribution of a Hippocampal Avoidance WBRT (HA-WBRT) in Helical Tomotherapy. The prescription dose was 30 Gy/10 fx with hippocampal sparing (outlined on the right in brown and on the left in light blue, with an expansion margin of 5 mm corresponding to the PRV).
Gondi et al. first proved that HA-WBRT is able to reduce both maximum and mean dose per fraction delivered to the hippocampus (94). For a prescription dose of 30 Gy in 10 fractions to the whole brain, HA-WBRT is able to reduce the mean dose per fraction to the hippocampus (normalized to 2-Gy fractions) by 87% using helical tomotherapy and by 81% using LINAC-based IMRT. The maximum dose received by the hippocampus is 12.8 Gy (Dmean 5.5 Gy) using helical tomotherapy, and 15.3 Gy (Dmean 7.8 Gy) using LINAC based IMRT, with acceptable target coverage and homogeneity (94) (Figure 5).
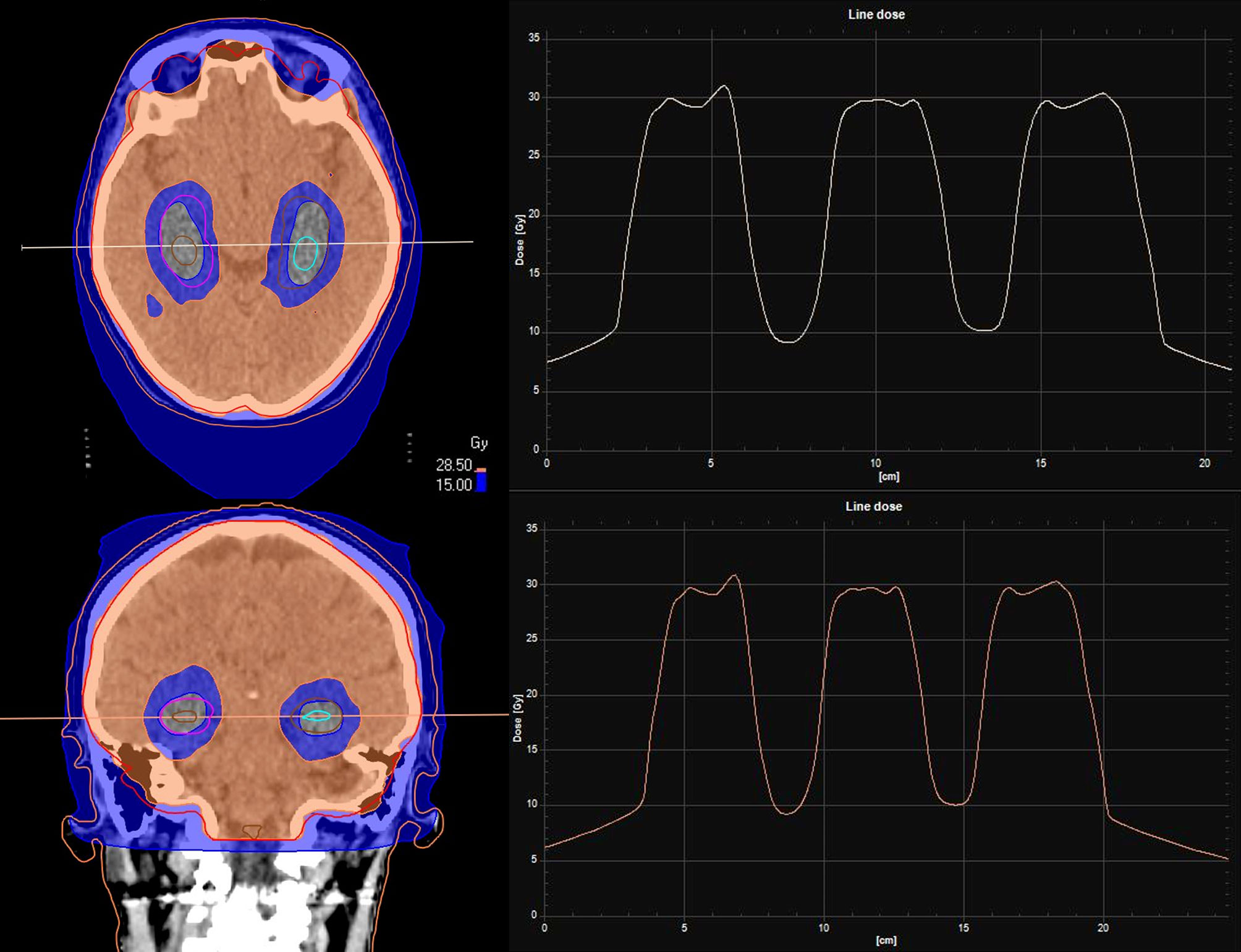
Figure 5 Dose line of HA-WBRT: the dose line shows the drop in dose at the level of the hippocampi, and the rapid rise to the prescription dose of 30 Gy/10 fr in the surrounding areas included in the CTV of the WBRT.
On the basis of these feasibility analyses, randomized prospective trials were designed to prove the efficacy of HA-WBRT as a viable option for polymetastatic disease. The single-arm phase II RTOG 0933 trial on HA-WBRT for BMs reported significant memory preservation (assessed through reduction of HVLT-R Delayed Recall decline), compared with historical data of patients treated with standard WBRT (92). Further confirmation of the effectiveness of HA-WBRT came from a prospective randomized phase III trial (NRG-CC001) which evaluated the potential combined neuroprotective effects of hippocampal avoidance in addition to prophylactic memantine during WBRT. HA-WBRT + memantine prolonged time to neurocognitive failure; decrease in neurocognitive function at 6 months was 59.5% vs. 68.2% (HR: 0.76, p = 0.03) favouring the combination of HA-WBRT + memantine, without any difference in intracranial recurrences (p = 0.208) or OS (p = 0.307) (95).
Ongoing research on the pathophysiology of brain irradiation damage may reveal other possible important brain structures or hippocampal subregions (e.g. cornu ammonis) whose sparing could also contribute to better preservation of neurocognitive function (NCT04801342, NCT03223922).
It should be noted that sparing the hippocampus and the peri-hippocampal region determines the theoretical risk of intra-cranial disease progression/relapse in these anatomical regions. However, it is estimated that this volume accounts for approximately 2% of the whole brain and the incidence of the development of metastases in this area is an uncommon event with a detection rate of less than 10% in previous reports (94).
Conclusions: HA-WBRT is an alternative solution and should be considered in selected patients with good KPS to better preserve cognitive function and patient-reported symptoms. The combination with memantine proved to improve the neurocognitive endpoints in a RTOG trial and is now approved in the United States in combination with HA-WBRT.
Focal Treatments as an Alternative to WBRT
Although current evidence confirms WBRT as the standard treatment for patients with multiple BMs (75), focal irradiation is increasingly used in daily clinical practice and many institutions extend its utilization beyond the oligometastatic setting, mostly in fear of the neurocognitive side effects of WBRT. It remains controversial which subpopulation of multiple BM patients may obtain the greater benefit from local treatments like SRS. In published series, predictive factors for LC comprise delivered dose, total volume of treated metastasis, and histology of the primary tumor (96–100) without a clear correlation with the total number of BMs. To date, only two prospective studies have been published. Thus, clinical data is currently quite limited. The multicenter phase 2 study by the Japanese Leksell Gamma Knife Society (JLGK0901) reported the outcome of patients treated with SRS alone upfront, regardless of the number of BMs, and reported the significant inferiority in OS for patients with 5-10 BMs (largest tumor <10 mL in volume and <3 cm in longest diameter; total cumulative volume ≤15 mL), compared with patients with only 2-4 BMs, although the difference in median survival time among the two groups was not clinically meningful (7.0 vs 7.9 respectively). On the other hand, cumulative incidences of neurological death at 6, 12, and 24 months after SRS did not differ significantly between patients with 2-4 BMs, and those with 5-10 BMs, nor did the cumulative incidence of neurological deterioration after SRS (11.6% vs 13%, p=0.54). At 12 months after SRS, neurocognitive function was maintained in 91% of patients with 2-4 BMs and 88% of patients with 5-10 BMs (p=0.60) (101).
Subsequently, Yamamoto et al. conducted a dedicated case-matched study comparing SRS in patients with 2–9 BMs and in those with 10 or more BMs. In this study, median survival time for the two groups was not significantly different, likewise neurological death-free survival, cumulative incidence of local recurrence, use of salvage SRS for new lesions, neurological deterioration and SRS-related complications. They concluded that even patients with 10 or more BMs may be suitable candidates for SRS after careful selection (e.g., low intracranial tumor burden) (100). A multicentre, single-arm, phase 2 study by Nichol et al. also reported the effectiveness and tolerability of SRS for patients with 1 to 10 BMs (102).
Other retrospective studies have confirmed SRS as appropriate in patients with polymetastatic disease with LC rate and toxicity comparable to those observed in patients with a limited number of BMs (103–105).
Recently, the first phase III randomized controlled trial (Dutch Trial) investigating WBRT versus SRS for patients with 4–10 BM suggested that SRS is a valid palliative treatment option for patients with polymetastatic disease for the reduced incidence of toxicity and for the preservation of QoL. Anyway, the trial was closed prematurely, mainly as a result of patients’ and doctors’ preference for SRS, and no definitive results were produced on the non-inferiority of SRS in term of OS and brain failure-free survival (106).
Ongoing phase III trials directly comparing WBRT to SRS in patients with multiple BMs will hopefully bring more relevant data and evidence to guide the treatment selection.
A randomized phase III trial at the MDACC has randomized 72 patients with 4–15 BMs from a non-melanoma primary tumor to SRS (15-24 Gy) or WBRT (30 Gy) (107). The results presented at the annual ASTRO meeting in 2020 showed similar LC, new onset of BMs and OS (approximately 8 months) among the two cohorts, while patients receiving SRS had a shorter time to systemic therapies (2 weeks vs 4) and a better preservation of neurocognitive function. A clinical trial led by the National Cancer Information Center, is randomizing patients with 5-15 BMs to SRS or WBRT in combination with memantine. OS and neurocognitive deterioration free survival are the primary endpoints (NCT03550391). Another trial from Dana-Farber Cancer Institute (NCT03075072) is randomizing patients with 5-20 BMs to SRS or WBRT plus hippocampal sparing and QoL is the primary endpoint (108). Table 3 resumes the ongoing studies investigating this innovative setting.
Conclusions: WBRT remains the standard treatment for patients with >4 metastases. However, there is growing evidence to support the role of SRS for patients with 4-15 metastases to improve the risk-benefit ratio of these patients, reserving WBRT as salvage treatment in case of rapid and progressive intracranial disease. It remains controversial which subpopulation of multiple BM patients benefits most from local treatments, including SRS.
How to Improve Local Control
WBRT+Simultaneous Integrated Boost (SIB)
In the past, dose escalation with WBRT plus sequential boost was employed to improve LC within the context of oligometastatic disease (11, 16).
The new technical frontier is to combine WBRT with a simultaneous integrated boost (SIB) in patients with multiple BMs. Modern RT techniques, such as dynamic IMRT and VMAT, are able to generate steep dose gradients between close volumes and to simultaneously delivery different doses to the whole brain and to visible BMs. SIB is then the technological evolution of the sequential boost, with several technical and logistical advantages. SIB deliveries/includes WBRT and a boost on visible BMs in the same session, with an optimized dose distribution, a single simulation protocol, an improvement in patients’ compliance and a reduction in overall treatment time and costs (109). The first feasibility studies involved patients with a limited number or volume of BMs (110, 111). The same results emerged even in patients with a larger number and volume of BMs (109, 112). WBRT+SIB on large lesions appears to be safe and effective even for patients with 4-10 BMs, without significant cognitive decline (113); therefore, SIB is frequently employed for the treatment of poly-metastatic disease. Recently it has also been shown to provide a significantly longer median intracranial PFS (9.1 vs 5.9 months, p=0.001) and median OS (14 vs 11 months, p=0.037) compared to WBRT + sequential boost (114, 115). There is no consensus on the most appropriate hypofractionation schedule and each institute bases the choice on clinical judgment and experience. The most frequently used schedule is in 5 daily fractions with a dose of 20 Gy (4 Gy per day) to the whole brain and a SIB of 40 Gy (8 Gy per day) to the visible BMs (110).
Modern technologies allow for a further innovative step, represented by the addition of hippocampal avoidance in this integrated approach (HA-WBRT+SIB). Through a VMAT or, even better, helical tomotherapy planning, it is possible to simultaneously spare the hippocampi, deliver high doses of radiation to multiple metastases and treat the remaining brain volume with a homogeneous dose distribution, all in a single treatment plan/session (116, 117) (Figure 6). HA-WBRT+SIB can be an effective therapeutic option for patients with multiple BMs: it shows improved LC of treated metastases (98% vs 82% at 1 year; P = 0.007) and improves overall intracranial PFS in comparison with WBRT alone (13.5 vs 6.4 months; P = 0.03) (118).
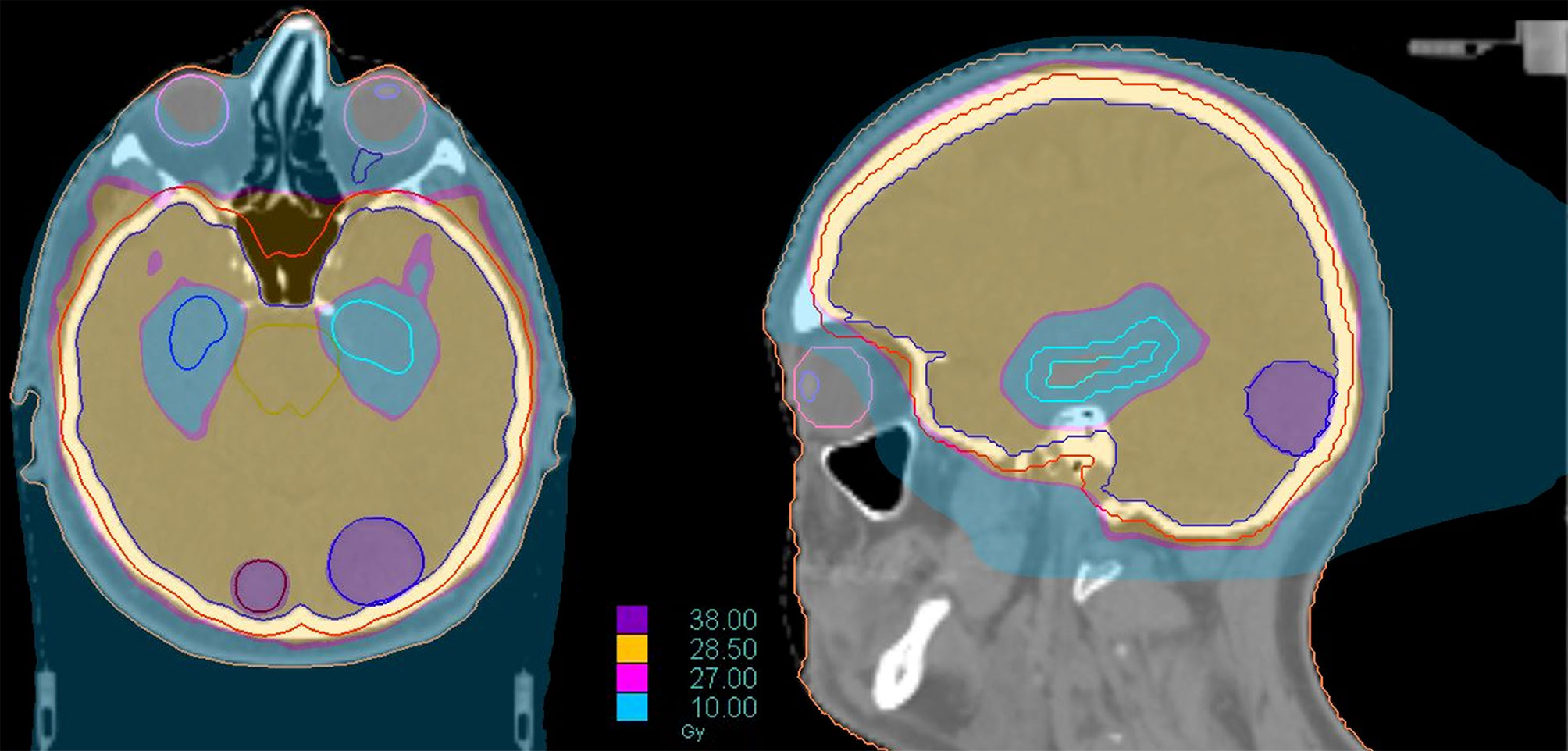
Figure 6 Dose distribution of a treatment of HA-WBRT plus SIB in patient with bilateral occipital BMs (left 2 cm; right 7 mm). The treatment was delivered with Helical tomotherapy and a daily control of the patient’s positioning with MV-CT. The dose delivered to the whole brain was 30 Gy in 10 fx with a concomitant boost of 40 Gy in 10 fx to the 2 lesions.
The potential of HA-WBRT+SIB to prevent neurocognitive effects and to concomitantly improve LC is currently under investigation in randomized, multicenter trials (119, 120).
Conclusions: WBRT+SIB improves LC for the treatment of multiple BMs. The combination of HA-WBRT with SIB could reduce deterioration in neurocognitive function and further improve the therapeutic index for these patients.
Leptomeningeal Dissemination
Leptomeningeal dissemination (LMD), also known as leptomeningeal carcinomatosis, is a rare complication occurring in ∼10% of patients with metastatic cancer, but is particularly frequent in patients with BMs originating from NSCLC (56-82% of cases), especially for adenocarcinomas (84-96%) (121). Neurosurgery may favor tumor cell spreading and therefore the incidence of LMD is higher in patients treated with surgery than in patients treated with upfront SRS (122). Without treatment, the median survival is dismal (6–8 weeks) in NSCLC patients, whereas survival may be prolonged beyond 6 months with treatment, including targeted therapy (123–125) and immunotherapy (126), with a 1-year OS rate of 19% (127, 128). LMD is frequently associated with moderate to severe neurological symptoms and the aim of treatment is to prolong survival while maintaining an acceptable QoL and delaying neurological deterioration.
There is no consensus regarding the optimal treatment for patients with LMD and recommendations for the treatment modalities are supported by a low level of evidence (129), as no randomized trial is currently available.
Diagnosis and management of patients with LMD should follow a multidisciplinary discussion.
Recently, a new radiological classification of LMD, based on MRI findings, is being developed in order to guide the selection of the optimal therapeutic strategy. It identifies linear LMD (type A), nodular leptomeningeal disease (type B), both (type C), no neuroimaging evidence of LMD or, at least, hydrocephalus (type D) (121, 130) (Figure 7).
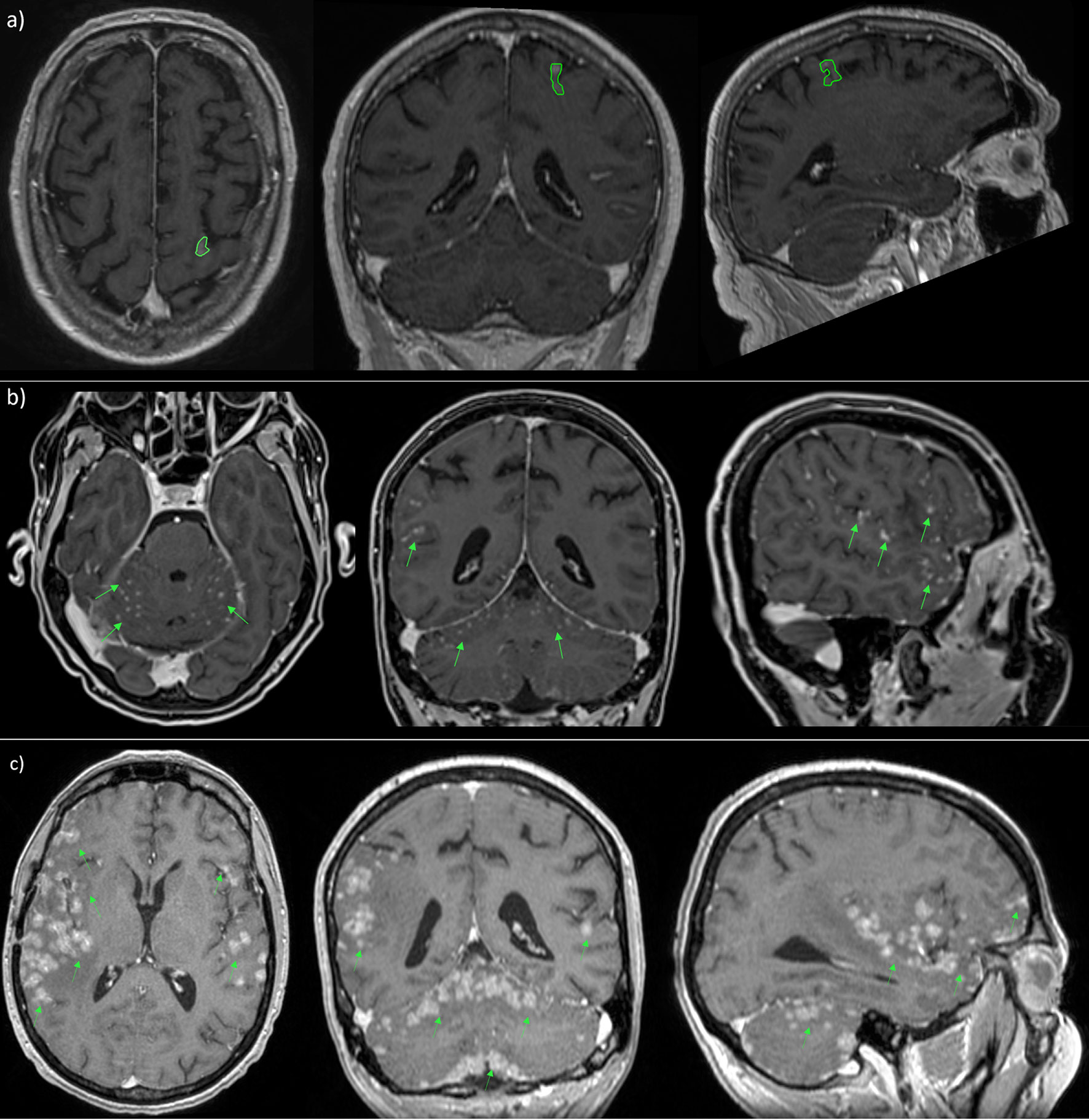
Figure 7 MRI scans in patient with nodular (A), linear (B) and both types (C) of leptomeningeal metastasis.
Current management approaches include a range of treatments such as systemic ChT for the primary tumor and extra-CNS disease (preferred choice for type B/C LMD), intrathecal ChT targeted therapy, RT, and supportive care (131).
In particular, the utility of WBRT for LMD has been evaluated retrospectively by Hirano and colleagues. The authors demonstrated that WBRT (30 Gy/10 fx) was effective for the relief of symptoms but provided only little benefit in these patients expected to have poor survival (132).
The NCCN has recently incorporated risk stratification and guidelines for the treatment of LMD. RT is typically included in the multimodal treatment of patients with Good Risk LMD, namely those with a KPS ≥60, the absence of major neurologic deficits, the presence of minimal systemic disease or the opportunity to start/prosecute a systemic treatment (12).
In current clinical practice, focal RT administered in fractionated regimens such as involved-field RT or SRT or SRS, can be used to treat nodular disease and symptomatic cerebral or spinal lesions. In rare cases, focal RT can be employed for cauda equina syndrome or cranial nerve palsy after exclusion of other causes, even in the absence of corresponding MRI findings.
Since the presence of cerebro-splinal fluid (CSF) flow interruptions is associated with decreased survival, normal CSF flow can be restored with focal RT in 30% of patients with spinal blocks and in 50% of patients with intracranial blocks, and has been proposed to reduce the toxicity, and enhance the efficacy of intra-CSF therapy (121). Typical target volumes for RT in the presence of cranial neuropathies include the base of cranial floor, the interpeduncular cistern and the first two cervical vertebrae, while in the presence of a cauda equina syndrome the lumbosacral vertebrae are also included.
WBRT may be a valid option in extensive nodular LMD, symptomatic linear LMD or coexisting BM.
Given the high rate of toxicity (bone marrow toxicity, enteritis and mucositis) and the usual co-existence of systemic disease, craniospinal RT, especially in combination with systemic or intra-CSF treatment, is rarely employed in reason of its severe toxicity.
A recent report by Prabhu et al. found that nodular LMD was a distinct pattern of LMD, associated with surgery and postoperative SRS, that was less likely to be symptomatic and had better OS rates than classical linear (“sugarcoating”) LMD (130).
Furthermore, when patients with LMD were treated with focal RT (SRS or partial brain), they had a higher risk of LMD recurrence, but no detrimental effect on OS compared with WBRT-containing regimens, which means focal RT provides a reasonable, less toxic, treatment strategy for these patients (131).
In conclusion, there is limited high-quality evidence to guide the optimal use of RT for the treatment of LMD, and there is a great need for prospective trials. Furthermore, in this changing environment with constant advancements in diagnostic procedures and in systemic therapy (targeted therapy and immunotherapy), the role of RT will likely continue to evolve and advance.
Conclusions: WBRT remains the standard treatment in case of LMD. Despite limited high-quality evidence, focal RT could be considered for localized lesions, especially if symptomatic.
Combined Modality Treatment: RT and Systemic Therapy
The increasing use of focal brain radiotherapy (SRS/SRT), even when dealing with multiple BMs, has led to consider the possibility of combining these techniques with systemic treatments, in order to exploit any potential synergic effect of “chemo-immuno-radiation” (133). Despite the various studies aimed at examining this combination various issues remain uncertain, including the potential toxicities of these associations (134).
In particular, there is lack of consensus regarding the ideal timing for RT and the need for a washout when a patient is receiving a systemic treatment. A recent review included 6384 patients and analyzed the toxicity of concurrent SRS and ChT, immunotherapy (IT), and/or targeted therapies (TT). Despite the heterogeneity of the studies included in this evaluation, the authors concluded that SRS combined with systemic therapy appears to be safe, with no significant increase of side effects (major bleeding, RN, skin toxicity), allowing the maintenance of systemic agents during SRS (135).
Cho et al. presented similar results for patients receiving Gamma knife SRS concurrently with IT or TT (136). They observed no statistically significant differences in the occurrence of RN, or intralesional hemorrhage in association with IT or TT during or after SRS.
Radiotherapy and Targeted Therapy
Early studies investigating the RT-TKI combination have compared patients treated with WBRT + erlotinib or WBRT alone, revealing a statistically significant increase in ORR, median PFS and median OS (137, 138). These results were not confirmed in a recent phase III trial, showing that concurrent erlotinib with WBRT does not improve intracranial PFS or OS for NSCLC patients with BM, but the combination therapy is well tolerated with no unexpected neurotoxicity (139). A retrospective study by Chen et al, comparing the combination of EGFR-TKI and RT (WBRT and SRS) and EGFR-TKI alone, revealed no statistically significant differences in PFS and OS but only in median time to intracranial progression (21.5 vs 15 mo, p=0.036) (140). In view of the inconclusive data published until now, ongoing prospective trials are examining the effects of the SRS/EGFR-TKI combination in patients with known EGFR-status (Table 4).
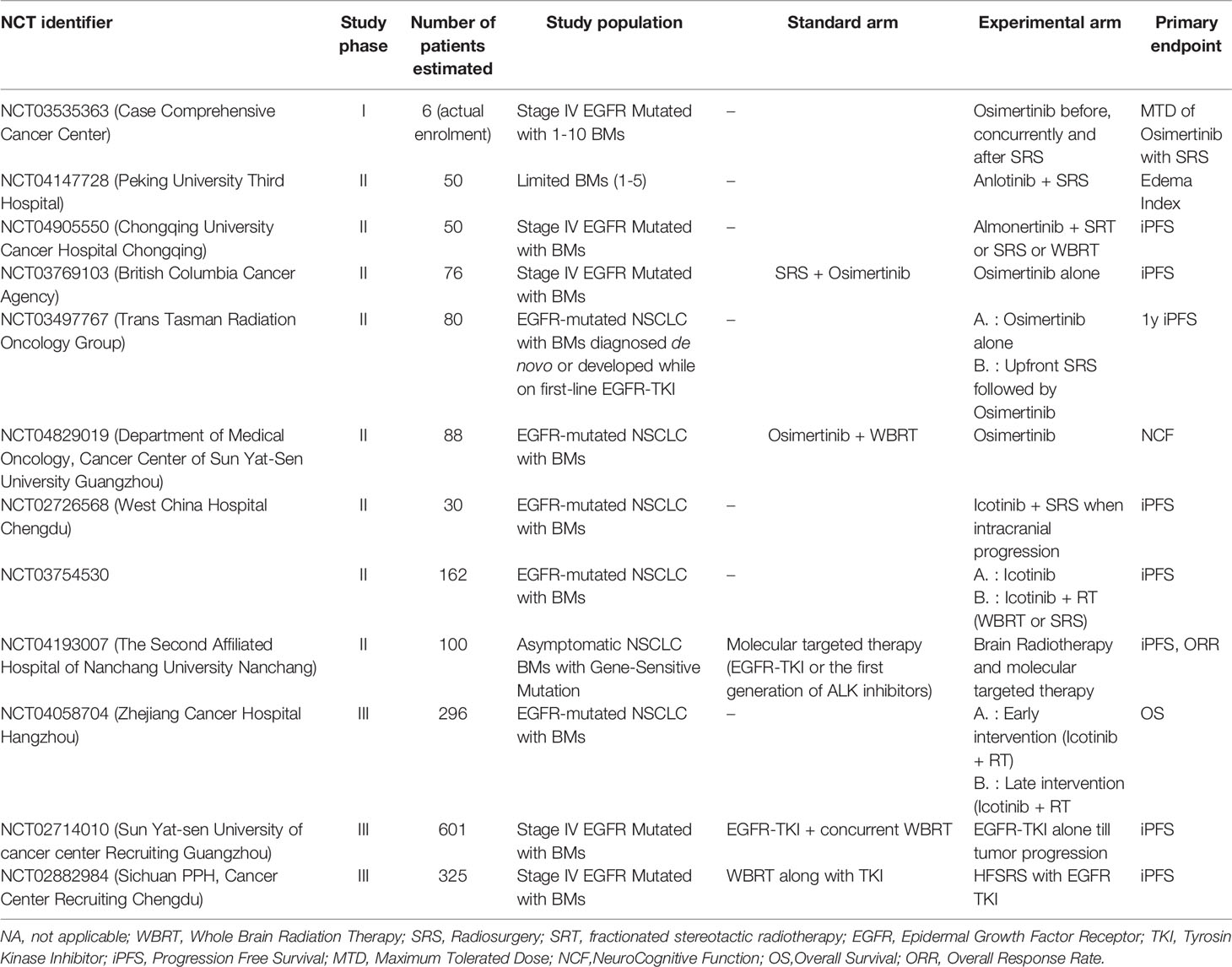
Table 4 Selected ongoing clinical trials investigating the combination of target therapies and RT in NSCLC patients with BMs.
Regarding the association of ALK inhibitors and RT, the combination of crizotinib and brain RT brought a statistically significant increase in ORR and median time to tumor progression (7 vs 13.2 months, respectively) (141). Another cohort of ALK-rearranged NSCLC patients treated with RT and TKIs showed favorable intracranial PFS and OS rates (142). However, the ASCEND-4 trial presented no statistically significant differences in terms of outcomes for the addition of RT to ceritinib (143). No relevant results and acute toxicity data are available to justify the implementation in clinical practice of the combination ALK-TKI- RT. To date most of the ongoing trials evaluate the combination EGFR-TKI- RT.
Conclusions: The combination of EGFR and ALK TKIs + RT is still under investigation and preliminary results suggest a possible benefit, particularly in terms of intracranial control, regardless of the radiotherapy technique (SRT or WBRT) and number of brain lesions. The ideal timing for the combination, the type of RT (focal/WBRT) and possible toxicities must be investigated.
Radiotherapy and Immunotherapy
The discovery of a lymphatic drainage system in the brain and the ability of T-cells to cross the blood-brain barrier has led to believe that the combination of RT and IT may improve antigen presentation in T-cells (144).
Data regarding the IT-RT combination in driver negative NSCLC patients, mostly from retrospective studies, is contradictory.
Clinical data revealed an increase in OS for the combination of RT and ICIs, starting immune checkpoint inhibitors (ICIs) at least 30 days earlier and continued throughout the RT treatment (145).
The retrospective study by Ahmed et al. presented no additional toxicity for NSCLC patients with BMs who received SRS and anti-PD1/PDL1 therapy (146).
So, there is reassuring data regarding the safety profile and efficacy of the combination of anti-PD1/anti-PDL1 agents and various RT regimens (SRS, WBRT) (147), with only a potential warning on an increased risk of RN (M155). In general practice, at least 50% of physicians do not interrupt ICIs when patients require SRS or WBRT (148).
A randomized study on ICIs with or without SRS in patients with asymptomatic BMs is still lacking to date. The Table 5 shows various ongoing trials examining the use of IT together with different brain radiation techniques.
Conclusions: The role of the RT-IT combination is still unclear. The current trend favors focal treatments such as SRS alone, or adjuvant SRS over WBRT, so further investigation of this combination is still required.
Prophylactic Cranial Irradiation (PCI)
Prophylactic cranial irradiation (PCI) is performed with the aim of preventing the occurrence of BMs in BMs-naïve patients. Its role is well-established in patients with small cell lung carcinoma (SCLC), with a demonstrated improvement in OS (149), while it is not clearly supported by current literature data for the treatment of stage III NSCLC.
The first trial dates back to 50 years ago. The VALG study identified a potential benefit of PCI in patients with NSCLC (150) with a reduced incidence of BMs by approximately 6%, but with no impact on OS. The most recent randomized phase III studies showed similar results with a significant reduction in the incidence of BMs, but no benefit on OS (151–153), except for Li’s trial (but the study did not complete the recruitment), which showed a marginal and not statistically significant benefit in median OS (31 vs 27.4 p=0.13). Three meta-analyses of randomized studies have been published in recent years and showed identical results, with a decrease in the incidence of metastasis with PCI and no difference in OS, QoL or toxicity (154).
The results of the phase 3 NVALT/DLCRG- 02 trial were recently published. They confirmed a statistically significant reduction in BM incidence (27% vs 7% at 2 years after therapy, p=0.001) and a prolonged time to BM onset (p=0.012) in favor of PCI, but again no statistically significant difference in QoL and OS (155). On the other hand, patients receiving PCI had a significant increase of cognitive disorders (19% vs 3%) and of memory impairment (G1-2 in 30% vs 8%).
In conclusion, the addition of PCI significantly prolongs PFS and BM free survival in NSCLC patients, but has no impact on OS (156). Given the potential neurotoxicity of this approach, observation is the preferred strategy in the clinical routine, with the possibility to offer salvage RT, even as focal SRS or WBRT, at the time of progression.
Conclusions: The actual evidence do not support PCI as a standard treatment in patients with NSCLC.
Discussion
The management of NSCLC patients with BMs is rapidly evolving. The advent of technological advances in imaging, radiotherapy planning and delivery techniques are rapidly replacing standard WBRT with SRS or with more conformal and less toxic solution of “extended brain irradiation” in order to reduce the therapeutic burden and to improve the risk benefit ratio in patients with metastatic brain disease. This review presents the different clinical settings of CNS metastatic involvement in NSCLC patients, with a main focus on the actual evidence-based indications and on the new technological frontiers to offer a high-quality and up-to-date RT treatment. In particular, we described the multiple technical solution to offer a focal RT treatment and to prevent the neurocognitive impairment related to WBRT, when and if the literature data do not support anymore the extended irradiation of the whole brain as an upfront management of BMs. In fact, WBRT is no longer the standard treatment for many patients with BMs from NSCLC. In reason of the growing evidence and understanding on the negative effects of WBRT on neurocognitive function and QoL, alternative RT options such as SRS and SRT are increasingly considered. The main aim of this strategy is to manage BMs with a minimal therapeutic burden to delay as much as possible WBRT and all its related side effects. This approach is certainly feasible and supported by literature data in patients with 1 or few (2–4) BMs, with the possibility even to repeat multiple sessions at different times without compromising patients’ outcome. The ambitious attempt to extend the indication to SRS and SRT to patients with a poly-metastatic CNS involvement (>5 BMs) is currently under investigation. When WBRT is eventually indicated, the evolving landscape offers the opportunity to revitalize this controversial RT solution through the implementation of hippocampal sparing (eventually combined with a neuroprotector like memantine), that may significantly reduce the related risk of neurotoxicity. Moreover, the advent of target therapies and immunotherapies, whose optimal role has been well established in selected patients, offers a novel and effective tool to treat NSCLC patients with BMs. A new era in upon us and the timing of SRS, SRT, WBRT and systemic agents will need to be reassessed and refined in order to find the best combination of these approaches for each single patient on a case-by-case selection. In the era of precision medicine, it is fundamental to guide treatment selection with the available prognostic scores (like lung-mol GPA), which include clinical, molecular and cancer-related parameters in order to stratify patients’ risk and to accurately estimate their prognosis. Finally, a multidisciplinary discussion is mandatory to accurately weigh each single risk factor and to tailor the therapeutic offer to the patients’ need.
Author Contributions
Study conception: CM and ML. Literature research: AG and MC. Manuscript preparation: CM, AG, MC and ML. Figure and table design: AG and MC. Manuscript editing and revision: all the authors. All authors contributed to the article and approved the submitted version.
Funding
The publication fee was supported by a grant from the Department of Oncology of University of Torino (Italy).
Conflict of Interest
The authors declare that the research was conducted in the absence of any commercial or financial relationships that could be construed as a potential conflict of interest.
Publisher’s Note
All claims expressed in this article are solely those of the authors and do not necessarily represent those of their affiliated organizations, or those of the publisher, the editors and the reviewers. Any product that may be evaluated in this article, or claim that may be made by its manufacturer, is not guaranteed or endorsed by the publisher.
Acknowledgments
The authors would like to thank Dr. Elena Gallio for the invaluable help in preparing and editing the figures of this paper.
References
1. Ulahannan D, Khalifa J, Faivre-Finn C, Lee S-M. Emerging Treatment Paradigms for Brain Metastasis in Non-Small-Cell Lung Cancer: An Overview of the Current Landscape and Challenges Ahead. Ann Oncol (2017) 28:2923–31. doi: 10.1093/annonc/mdx481
2. Waqar SN, Samson PP, Robinson CG, Bradley J, Devarakonda S, Du L, et al. Non-Small-Cell Lung Cancer With Brain Metastasis at Presentation. Clin Lung Cancer (2018) 19:e373–9. doi: 10.1016/j.cllc.2018.01.007
3. Lagerwaard FJ, Levendag PC, Nowak PJ, Eijkenboom WM, Hanssens PE, Schmitz PI. Identification of Prognostic Factors in Patients With Brain Metastases: A Review of 1292 Patients. Int J Radiat Oncol Biol Phys (1999) 43:795–803. doi: 10.1016/s0360-3016(98)00442-8
4. Gaspar L, Scott C, Rotman M, Asbell S, Phillips T, Wasserman T, et al. Recursive Partitioning Analysis (RPA) of Prognostic Factors in Three Radiation Therapy Oncology Group (RTOG) Brain Metastases Trials. Int J Radiat Oncol Biol Phys (1997) 37:745–51. doi: 10.1016/s0360-3016(96)00619-0
5. Sperduto PW, Berkey B, Gaspar LE, Mehta M, Curran W. A New Prognostic Index and Comparison to Three Other Indices for Patients With Brain Metastases: An Analysis of 1,960 Patients in the RTOG Database. Int J Radiat Oncol Biol Phys (2008) 70:510–4. doi: 10.1016/j.ijrobp.2007.06.074
6. Sperduto PW, Kased N, Roberge D, Xu Z, Shanley R, Luo X, et al. Summary Report on the Graded Prognostic Assessment: An Accurate and Facile Diagnosis-Specific Tool to Estimate Survival for Patients With Brain Metastases. J Clin Oncol (2012) 30:419–25. doi: 10.1200/JCO.2011.38.0527
7. Sperduto PW, Yang TJ, Beal K, Pan H, Brown PD, Bangdiwala A, et al. Estimating Survival in Patients With Lung Cancer and Brain Metastases: An Update of the Graded Prognostic Assessment for Lung Cancer Using Molecular Markers (Lung-molGPA). JAMA Oncol (2017) 3:827–31. doi: 10.1001/jamaoncol.2016.3834
8. Scoccianti S, Ricardi U. Treatment of Brain Metastases: Review of Phase III Randomized Controlled Trials. Radiother Oncol (2012) 102:168–79. doi: 10.1016/j.radonc.2011.08.041
9. Patchell RA, Tibbs PA, Walsh JW, Dempsey RJ, Maruyama Y, Kryscio RJ, et al. A Randomized Trial of Surgery in the Treatment of Single Metastases to the Brain. N Engl J Med (1990) 322:494–500. doi: 10.1056/NEJM199002223220802
10. Vecht CJ, Haaxma-Reiche H, Noordijk EM, Padberg GW, Voormolen JH, Hoekstra FH, et al. Treatment of Single Brain Metastasis: Radiotherapy Alone or Combined With Neurosurgery? Ann Neurol (1993) 33:583–90. doi: 10.1002/ana.410330605
11. Andrews DW, Scott CB, Sperduto PW, Flanders AE, Gaspar LE, Schell MC, et al. Whole Brain Radiation Therapy With or Without Stereotactic Radiosurgery Boost for Patients With One to Three Brain Metastases: Phase III Results of the RTOG 9508 Randomised Trial. Lancet (2004) 363:1665–72. doi: 10.1016/S0140-6736(04)16250-8
12. Nabors LB, Portnow J, Ahluwalia M, Baehring J, Brem H, Brem S. Central Nervous System Cancers, Version 3.2020, NCCN Clinical Practice Guidelines in Oncology. J Natl Compr Canc Netw (2020) 18:1537–70. doi: 10.6004/jnccn.2020.0052
13. Han EY, Wang H, Luo D, Li J, Wang X. Dosimetric Comparison of Fractionated Radiosurgery Plans Using Frameless Gamma Knife ICON and CyberKnife Systems With Linear Accelerator-Based Radiosurgery Plans for Multiple Large Brain Metastases. J Neurosurg (2019) 132:1473–9. doi: 10.3171/2019.1.JNS182769
14. Sturm V, Kober B, Höver KH, Schlegel W, Boesecke R, Pastyr O, et al. Stereotactic Percutaneous Single Dose Irradiation of Brain Metastases With a Linear Accelerator. Int J Radiat Oncol Biol Phys (1987) 13:279–82. doi: 10.1016/0360-3016(87)90140-4
15. Lindquist C. Gamma Knife Surgery for Recurrent Solitary Metastasis of a Cerebral Hypernephroma: Case Report. Neurosurgery (1989) 25:802–4. doi: 10.1097/00006123-198911000-00018
16. Aoyama H, Shirato H, Tago M, Nakagawa K, Toyoda T, Hatano K, et al. Stereotactic Radiosurgery Plus Whole-Brain Radiation Therapy vs Stereotactic Radiosurgery Alone for Treatment of Brain Metastases: A Randomized Controlled Trial. JAMA (2006) 295:2483–91. doi: 10.1001/jama.295.21.2483
17. Chang EL, Wefel JS, Hess KR, Allen PK, Lang FF, Kornguth DG, et al. Neurocognition in Patients With Brain Metastases Treated With Radiosurgery or Radiosurgery Plus Whole-Brain Irradiation: A Randomised Controlled Trial. Lancet Oncol (2009) 10:1037–44. doi: 10.1016/S1470-2045(09)70263-3
18. Kocher M, Soffietti R, Abacioglu U, Villà S, Fauchon F, Baumert BG, et al. Adjuvant Whole-Brain Radiotherapy Versus Observation After Radiosurgery or Surgical Resection of One to Three Cerebral Metastases: Results of the EORTC 22952-26001 Study. J Clin Oncol (2011) 29:134–41. doi: 10.1200/JCO.2010.30.1655
19. Brown PD, Jaeckle K, Ballman KV, Farace E, Cerhan JH, Anderson SK, et al. Effect of Radiosurgery Alone vs Radiosurgery With Whole Brain Radiation Therapy on Cognitive Function in Patients With 1 to 3 Brain Metastases: A Randomized Clinical Trial. JAMA (2016) 316:401–9. doi: 10.1001/jama.2016.9839
20. Patchell RA, Tibbs PA, Regine WF, Dempsey RJ, Mohiuddin M, Kryscio RJ, et al. Postoperative Radiotherapy in the Treatment of Single Metastases to the Brain: A Randomized Trial. JAMA (1998) 280:1485–9. doi: 10.1001/jama.280.17.1485
21. Kayama T, Sato S, Sakurada K, Mizusawa J, Nishikawa R, Narita Y, et al. Effects of Surgery With Salvage Stereotactic Radiosurgery Versus Surgery With Whole-Brain Radiation Therapy in Patients With One to Four Brain Metastases (JCOG0504): A Phase III, Noninferiority, Randomized Controlled Trial. J Clin Oncol (2018) 33:3282–9. doi: 10.1200/JCO.2018.78.6186
22. Sahgal A, Aoyama H, Kocher M, Neupane B, Collette S, Tago M, et al. Phase 3 Trials of Stereotactic Radiosurgery With or Without Whole-Brain Radiation Therapy for 1 to 4 Brain Metastases: Individual Patient Data Meta-Analysis. Int J Radiat Oncol Biol Phys (2015) 91:710–7. doi: 10.1016/j.ijrobp.2014.10.024
23. Shaw E, Scott C, Souhami L, Dinapoli R, Bahary JP, Kline R, et al. Radiosurgery for the Treatment of Previously Irradiated Recurrent Primary Brain Tumors and Brain Metastases: Initial Report of Radiation Therapy Oncology Group Protocol (90-05). Int J Radiat Oncol Biol Phys (1996) 34:647–54. doi: 10.1016/0360-3016(95)02106-x
24. Shaw E, Scott C, Souhami L, Dinapoli R, Kline R, Loeffler J, et al. Single Dose Radiosurgical Treatment of Recurrent Previously Irradiated Primary Brain Tumors and Brain Metastases: Final Report of RTOG Protocol 90-05. Int J Radiat Oncol Biol Phys (2000) 47:291–8. doi: 10.1016/s0360-3016(99)00507-6
25. Vellayappan B, Tan CL, Yong C, Khor LK, Koh WY, Yeo TT, et al. Diagnosis and Management of Radiation Necrosis in Patients With Brain Metastases. Front Oncol (2018) 8:395. doi: 10.3389/fonc.2018.00395
26. Walker AJ, Ruzevick J, Malayeri AA, Rigamonti D, Lim M, Redmond KJ, et al. Postradiation Imaging Changes in the CNS: How can We Differentiate Between Treatment Effect and Disease Progression? Future Oncol (2014) 10:1277–97. doi: 10.2217/fon.13.271
27. Miller JA, Bennett EE, Xiao R, Kotecha R, Chao ST, Vogelbaum MA, et al. Association Between Radiation Necrosis and Tumor Biology After Stereotactic Radiosurgery for Brain Metastasis. Int J Radiat Oncol Biol Phys (2016) 96:1060–9. doi: 10.1016/j.ijrobp.2016.08.039
28. Chung C, Bryant A, Brown PD. Interventions for the Treatment of Brain Radionecrosis After Radiotherapy or Radiosurgery. Cochrane Database Syst Rev (2018) 7:CD011492. doi: 10.1002/14651858.CD011492.pub2
29. Lehrer EJ, Peterson JL, Zaorsky NG, Brown PD, Sahgal A, Chiang VL, et al. Single Versus Multifraction Stereotactic Radiosurgery for Large Brain Metastases: An International Meta-Analysis of 24 Trials. Int J Radiat Oncol Biol Phys (2019) 103:618–30. doi: 10.1016/j.ijrobp.2018.10.038
30. Redmond KJ, Gui C, Benedict S, Milano MT, Grimm J, Vargo JA, et al. Tumor Control Probability of Radiosurgery and Fractionated Stereotactic Radiosurgery for Brain Metastases. Int J Radiat Oncol Biol Phys (2021) 110:53–67. doi: 10.1016/j.ijrobp.2020.10.034
31. Milano MT, Grimm J, Niemierko A, Soltys SG, Moiseenko V, Redmond KJ, et al. Single- and Multifraction Stereotactic Radiosurgery Dose/Volume Tolerances of the Brain. Int J Radiat Oncol Biol Phys (2021) 110:68–86. doi: 10.1016/j.ijrobp.2020.08.013
32. Muacevic A, Wowra B, Siefert A, Tonn J-C, Steiger H-J, Kreth FW. Microsurgery Plus Whole Brain Irradiation Versus Gamma Knife Surgery Alone for Treatment of Single Metastases to the Brain: A Randomized Controlled Multicentre Phase III Trial. J Neurooncol (2008) 87:299–307. doi: 10.1007/s11060-007-9510-4
33. Roos DE, Smith JG, Stephens SW. Radiosurgery Versus Surgery, Both With Adjuvant Whole Brain Radiotherapy, for Solitary Brain Metastases: A Randomised Controlled Trial. Clin Oncol (R Coll Radiol) (2011) 23:646–51. doi: 10.1016/j.clon.2011.04.009
34. Fuentes R, Osorio D, Expósito Hernandez J, Simancas-Racines D, Martinez-Zapata MJ, Bonfill Cosp X. Surgery Versus Stereotactic Radiotherapy for People With Single or Solitary Brain Metastasis. Cochrane Database Syst Rev (2018) 8:CD012086. doi: 10.1002/14651858.CD012086.pub2
35. Quigley MR, Bello N, Jho D, Fuhrer R, Karlovits S, Buchinsky FJ. Estimating the Additive Benefit of Surgical Excision to Stereotactic Radiosurgery in the Management of Metastatic Brain Disease. Neurosurgery (2015) 76:707–12. doi: 10.1227/NEU.0000000000000707
36. Churilla TM, Chowdhury IH, Handorf E, Collette L, Collette S, Dong Y, et al. Comparison of Local Control of Brain Metastases With Stereotactic Radiosurgery vs Surgical Resection: A Secondary Analysis of a Randomized Clinical Trial. JAMA Oncol (2019) 5:243–7. doi: 10.1001/jamaoncol.2018.4610
37. Akanda ZZ, Hong W, Nahavandi S, Haghighi N, Phillips C, Kok DL. Post-Operative Stereotactic Radiosurgery Following Excision of Brain Metastases: A Systematic Review and Meta-Analysis. Radiother Oncol (2020) 142:27–35. doi: 10.1016/j.radonc.2019.08.024
38. Mahajan A, Ahmed S, McAleer MF, Weinberg JS, Li J, Brown P, et al. Post-Operative Stereotactic Radiosurgery Versus Observation for Completely Resected Brain Metastases: A Single-Centre, Randomised, Controlled, Phase 3 Trial. Lancet Oncol (2017) 18:1040–8. doi: 10.1016/S1470-2045(17)30414-X
39. Brown PD, Ballman KV, Cerhan JH, Anderson SK, Carrero XW, Whitton AC, et al. Postoperative Stereotactic Radiosurgery Compared With Whole Brain Radiotherapy for Resected Metastatic Brain Disease (NCCTG N107C/CEC·3): A Multicentre, Randomised, Controlled, Phase 3 Trial. Lancet Oncol (2017) 18:1049–60. doi: 10.1016/S1470-2045(17)30441-2
40. El Shafie RA, Paul A, Bernhardt D, Hauswald H, Welzel T, Sprave T, et al. Evaluation of Stereotactic Radiotherapy of the Resection Cavity After Surgery of Brain Metastases Compared to Postoperative Whole-Brain Radiotherapy (ESTRON)-A Single-Center Prospective Randomized Trial. Neurosurgery (2018) 83:566–73. doi: 10.1093/neuros/nyy021
41. Minniti G, Esposito V, Clarke E, Scaringi C, Lanzetta G, Salvati M, et al. Multidose Stereotactic Radiosurgery (9 Gy × 3) of the Postoperative Resection Cavity for Treatment of Large Brain Metastases. Int J Radiat Oncol Biol Phys (2013) 86:623–9. doi: 10.1016/j.ijrobp.2013.03.037
42. Keller A, Doré M, Cebula H, Thillays F, Proust F, Darié I, et al. Hypofractionated Stereotactic Radiation Therapy to the Resection Bed for Intracranial Metastases. Int J Radiat Oncol Biol Phys (2017) 99:1179–89. doi: 10.1016/j.ijrobp.2017.08.014
43. Faruqi S, Ruschin M, Soliman H, Myrehaug S, Zeng KL, Husain Z, et al. Adverse Radiation Effect After Hypofractionated Stereotactic Radiosurgery in 5 Daily Fractions for Surgical Cavities and Intact Brain Metastases. Int J Radiat Oncol Biol Phys (2020) 106:772–9. doi: 10.1016/j.ijrobp.2019.12.002
44. Shi S, Sandhu N, Jin MC, Wang E, Jaoude JA, Schofield K, et al. Stereotactic Radiosurgery for Resected Brain Metastases: Single-Institutional Experience of Over 500 Cavities. Int J Radiat Oncol Biol Phys (2020) 106:764–71. doi: 10.1016/j.ijrobp.2019.11.022
45. Eitz KA, Lo SS, Soliman H, Sahgal A, Theriault A, Pinkham MB, et al. Multi-Institutional Analysis of Prognostic Factors and Outcomes After Hypofractionated Stereotactic Radiotherapy to the Resection Cavity in Patients With Brain Metastases. JAMA Oncol (2020) 6:1901–9. doi: 10.1001/jamaoncol.2020.4630
46. Al-Omair A, Soliman H, Xu W, Karotki A, Mainprize T, Phan N, et al. Hypofractionated Stereotactic Radiotherapy in Five Daily Fractions for Post-Operative Surgical Cavities in Brain Metastases Patients With and Without Prior Whole Brain Radiation. Technol Cancer Res Treat (2013) 12:493–9. doi: 10.7785/tcrt.2012.500336
47. Minniti G, Niyazi M, Andratschke N, Guckenberger M, Palmer JD, Shih HA, et al. Current Status and Recent Advances in Resection Cavity Irradiation of Brain Metastases. Radiat Oncol (2021) 16:73. doi: 10.1186/s13014-021-01802-9
48. Soliman H, Ruschin M, Angelov L, Brown PD, Chiang VLS, Kirkpatrick JP, et al. Consensus Contouring Guidelines for Postoperative Completely Resected Cavity Stereotactic Radiosurgery for Brain Metastases. Int J Radiat Oncol Biol Phys (2018) 100:436–42. doi: 10.1016/j.ijrobp.2017.09.047
49. Atalar B, Modlin LA, Choi CYH, Adler JR, Gibbs IC, Chang SD, et al. Risk of Leptomeningeal Disease in Patients Treated With Stereotactic Radiosurgery Targeting the Postoperative Resection Cavity for Brain Metastases. Int J Radiat Oncol Biol Phys (2013) 87:713–8. doi: 10.1016/j.ijrobp.2013.07.034
50. Hsieh J, Elson P, Otvos B, Rose J, Loftus C, Rahmathulla G, et al. Tumor Progression in Patients Receiving Adjuvant Whole-Brain Radiotherapy vs Localized Radiotherapy After Surgical Resection of Brain Metastases. Neurosurgery (2015) 76:411–20. doi: 10.1227/NEU.0000000000000626
51. Asher AL, Burri SH, Wiggins WF, Kelly RP, Boltes MO, Mehrlich M, et al. A New Treatment Paradigm: Neoadjuvant Radiosurgery Before Surgical Resection of Brain Metastases With Analysis of Local Tumor Recurrence. Int J Radiat Oncol Biol Phys (2014) 88:899–906. doi: 10.1016/j.ijrobp.2013.12.013
52. Prabhu RS, Miller KR, Asher AL, Heinzerling JH, Moeller BJ, Lankford SP, et al. Preoperative Stereotactic Radiosurgery Before Planned Resection of Brain Metastases: Updated Analysis of Efficacy and Toxicity of a Novel Treatment Paradigm. J Neurosurg (2018) 131:1387–94. doi: 10.3171/2018.7.JNS181293
53. Patel KR, Burri SH, Asher AL, Crocker IR, Fraser RW, Zhang C, et al. Comparing Preoperative With Postoperative Stereotactic Radiosurgery for Resectable Brain Metastases: A Multi-Institutional Analysis. Neurosurgery (2016) 79:279–85. doi: 10.1227/NEU.0000000000001096
54. Patel KR, Burri SH, Boselli D, Symanowski JT, Asher AL, Sumrall A, et al. Comparing Pre-Operative Stereotactic Radiosurgery (SRS) to Post-Operative Whole Brain Radiation Therapy (WBRT) for Resectable Brain Metastases: A Multi-Institutional Analysis. J Neurooncol (2017) 131:611–8. doi: 10.1007/s11060-016-2334-3
55. Vetlova E, Golbin DA, Golanov AV, Potapov AA, Banov SM, Antipina N, et al. Preoperative Stereotactic Radiosurgery of Brain Metastases: Preliminary Results. Cureus (2017) 9:e1987. doi: 10.7759/cureus.1987
56. Patel AR, Nedzi L, Lau S, Barnett SL, Mickey BE, Moore W, et al. Neoadjuvant Stereotactic Radiosurgery Before Surgical Resection of Cerebral Metastases. World Neurosurg (2018) 120:e480–7. doi: 10.1016/j.wneu.2018.08.107
57. Prabhu RS, Dhakal R, Vaslow ZK, Dan T, Mishra MV, Murphy ES, et al. Preoperative Radiosurgery for Resected Brain Metastases: The PROPS-BM Multicenter Cohort Study. Int J Radiat Oncol Biol Phys (2021) 111:764–72. doi: 10.1016/j.ijrobp.2021.05.124
58. Aoyama H, Tago M, Shirato H. Japanese Radiation Oncology Study Group 99-1 (JROSG 99-1) Investigators. Stereotactic Radiosurgery With or Without Whole-Brain Radiotherapy for Brain Metastases: Secondary Analysis of the JROSG 99-1 Randomized Clinical Trial. JAMA Oncol (2015) 1:457–64. doi: 10.1001/jamaoncol.2015.1145
59. Churilla TM, Handorf E, Collette S, Collette L, Dong Y, Aizer AA, et al. Whole Brain Radiotherapy After Stereotactic Radiosurgery or Surgical Resection Among Patients With One to Three Brain Metastases and Favorable Prognoses: A Secondary Analysis of EORTC 22952-26001. Ann Oncol (2017) 28:2588–94. doi: 10.1093/annonc/mdx332
60. Churilla TM, Ballman KV, Brown PD, Twohy EL, Jaeckle K, Farace E, et al. Stereotactic Radiosurgery With or Without Whole-Brain Radiation Therapy for Limited Brain Metastases: A Secondary Analysis of the North Central Cancer Treatment Group N0574 (Alliance) Randomized Controlled Trial. Int J Radiat Oncol Biol Phys (2017) 99:1173–8. doi: 10.1016/j.ijrobp.2017.07.045
61. Wu Y-L, Ahn M-J, Garassino MC, Han J-Y, Katakami N, Kim HR, et al. CNS Efficacy of Osimertinib in Patients With T790M-Positive Advanced Non-Small-Cell Lung Cancer: Data From a Randomized Phase III Trial (Aura3). J Clin Oncol (2018) 36:2702–9. doi: 10.1200/JCO.2018.77.9363
62. Reungwetwattana T, Nakagawa K, Cho BC, Cobo M, Cho EK, Bertolini A, et al. CNS Response to Osimertinib Versus Standard Epidermal Growth Factor Receptor Tyrosine Kinase Inhibitors in Patients With Untreated EGFR-Mutated Advanced Non-Small-Cell Lung Cancer. J Clin Oncol (2018) 33:3290–7. doi: 10.1200/JCO.2018.78.3118
63. Gadgeel S, Peters S, Mok T, Shaw AT, Kim DW, Ou SI, et al. Alectinib Versus Crizotinib in Treatment-Naive Anaplastic Lymphoma Kinase-Positive (ALK+) non-Small-Cell Lung Cancer: CNS Efficacy Results From the ALEX Study. Ann Oncol (2018) 29:2214–22. doi: 10.1093/annonc/mdy405
64. Felip E, Shaw AT, Bearz A, Camidge DR, Solomon BJ, Bauman JR, et al. Intracranial and Extracranial Efficacy of Lorlatinib in Patients With ALK-Positive non-Small-Cell Lung Cancer Previously Treated With Second-Generation ALK TKIs. Ann Oncol (2021) 32:620–30. doi: 10.1016/j.annonc.2021.02.012
65. Lim SH, Lee JY, Lee M-Y, Kim HS, Lee J, Sun J-M, et al. A Randomized Phase III Trial of Stereotactic Radiosurgery (SRS) Versus Observation for Patients With Asymptomatic Cerebral Oligo-Metastases in Non-Small-Cell Lung Cancer. Ann Oncol (2015) 26:762–8. doi: 10.1093/annonc/mdu584
66. Yu F, Ni J, Zeng W, Zhou Y, Guo T, Zeng Y, et al. Clinical Value of Upfront Cranial Radiation Therapy in Osimertinib-Treated Epidermal Growth Factor Receptor-Mutant Non-Small Cell Lung Cancer With Brain Metastases. Int J Radiat Oncol Biol Phys (2021) 111:804–15. doi: 10.1016/j.ijrobp.2021.05.125
67. Magnuson WJ, Lester-Coll NH, Wu AJ, Yang TJ, Lockney NA, Gerber NK, et al. Management of Brain Metastases in Tyrosine Kinase Inhibitor-Naïve Epidermal Growth Factor Receptor-Mutant Non-Small-Cell Lung Cancer: A Retrospective Multi-Institutional Analysis. J Clin Oncol (2017) 35:1070–7. doi: 10.1200/JCO.2016.69.7144
68. Palma DA, Salama JK, Lo SS, Senan S, Treasure T, Govindan R, et al. The Oligometastatic State - Separating Truth From Wishful Thinking. Nat Rev Clin Oncol (2014) 11:549–57. doi: 10.1038/nrclinonc.2014.96
69. Glatzer M, Faivre-Finn C, De Ruysscher D, Widder J, Van Houtte P, Troost EGC, et al. Role of Radiotherapy in the Management of Brain Metastases of NSCLC - Decision Criteria in Clinical Routine. Radiother Oncol (2021) 154:269–73. doi: 10.1016/j.radonc.2020.10.043
70. Tsao MN, Xu W, Wong RK, Lloyd N, Laperriere N, Sahgal A, et al. Whole Brain Radiotherapy for the Treatment of Newly Diagnosed Multiple Brain Metastases. Cochrane Database Syst Rev (2018) 1:CD003869. doi: 10.1002/14651858.CD003869.pub4
71. Khuntia D, Brown P, Li J, Mehta MP. Whole-Brain Radiotherapy in the Management of Brain Metastasis. J Clin Oncol (2006) 24:1295–304. doi: 10.1200/JCO.2005.04.6185
72. Zimm S, Wampler GL, Stablein D, Hazra T, Young HF. Intracerebral Metastases in Solid-Tumor Patients: Natural History and Results of Treatment. Cancer (1981) 48:384–94. doi: 10.1002/1097-0142(19810715)48:2<384::aid-cncr2820480227>3.0.co;2-8
73. Sneed PK, Larson DA, Wara WM. Radiotherapy for Cerebral Metastases. Neurosurg Clin N Am (1996) 7:505–15. doi: 10.1016/S1042-3680(18)30376-0
74. Mulvenna P, Nankivell M, Barton R, Faivre-Finn C, Wilson P, McColl E, et al. Dexamethasone and Supportive Care With or Without Whole Brain Radiotherapy in Treating Patients With Non-Small Cell Lung Cancer With Brain Metastases Unsuitable for Resection or Stereotactic Radiotherapy (QUARTZ): Results From a Phase 3, Non-Inferiority, Randomised Trial. Lancet (2016) 388:2004–14. doi: 10.1016/S0140-6736(16)30825-X
75. Brown PD, Ahluwalia MS, Khan OH, Asher AL, Wefel JS, Gondi V. Whole-Brain Radiotherapy for Brain Metastases: Evolution or Revolution? J Clin Oncol (2018) 36:483–91. doi: 10.1200/JCO.2017.75.9589
76. Raber J, Rola R, LeFevour A, Morhardt D, Curley J, Mizumatsu S, et al. Radiation-Induced Cognitive Impairments Are Associated With Changes in Indicators of Hippocampal Neurogenesis. Radiat Res (2004) 162:39–47. doi: 10.1667/rr3206
77. Monje ML, Toda H, Palmer TD. Inflammatory Blockade Restores Adult Hippocampal Neurogenesis. Science (2003) 302:1760–5. doi: 10.1126/science.1088417
78. Sheline GE, Wara WM, Smith V. Therapeutic Irradiation and Brain Injury. Int J Radiat Oncol Biol Phys (1980) 6:1215–28. doi: 10.1016/0360-3016(80)90175-3
79. Soussain C, Ricard D, Fike JR, Mazeron J-J, Psimaras D, Delattre J-Y. CNS Complications of Radiotherapy and Chemotherapy. Lancet (2009) 374:1639–51. doi: 10.1016/S0140-6736(09)61299-X
80. Bompaire F, Lahutte M, Buffat S, Soussain C, Ardisson AE, Terziev R, et al. New Insights in Radiation-Induced Leukoencephalopathy: A Prospective Cross-Sectional Study. Support Care Cancer (2018) 26:4217–26. doi: 10.1007/s00520-018-4296-9
81. Mehta MP, Rodrigus P, Terhaard CHJ, Rao A, Suh J, Roa W, et al. Survival and Neurologic Outcomes in a Randomized Trial of Motexafin Gadolinium and Whole-Brain Radiation Therapy in Brain Metastases. J Clin Oncol (2003) 21:2529–36. doi: 10.1200/JCO.2003.12.122
82. Monje ML, Palmer T. Radiation Injury and Neurogenesis. Curr Opin Neurol (2003) 16:129–34. doi: 10.1097/01.wco.0000063772.81810.b7
83. Belka C, Budach W, Kortmann RD, Bamberg M. Radiation Induced CNS Toxicity–Molecular and Cellular Mechanisms. Br J Cancer (2001) 85:1233–9. doi: 10.1054/bjoc.2001.2100
84. Brown PD, Pugh S, Laack NN, Wefel JS, Khuntia D, Meyers C, et al. Memantine for the Prevention of Cognitive Dysfunction in Patients Receiving Whole-Brain Radiotherapy: A Randomized, Double-Blind, Placebo-Controlled Trial. Neuro Oncol (2013) 15:1429–37. doi: 10.1093/neuonc/not114
85. Rapp SR, Case LD, Peiffer A, Naughton MM, Chan MD, Stieber VW, et al. Donepezil for Irradiated Brain Tumor Survivors: A Phase III Randomized Placebo-Controlled Clinical Trial. J Clin Oncol (2015) 33:1653–9. doi: 10.1200/JCO.2014.58.4508
86. Meyers CA, Smith JA, Bezjak A, Mehta MP, Liebmann J, Illidge T, et al. Neurocognitive Function and Progression in Patients With Brain Metastases Treated With Whole-Brain Radiation and Motexafin Gadolinium: Results of a Randomized Phase III Trial. J Clin Oncol (2004) 22:157–65. doi: 10.1200/JCO.2004.05.128
87. Mehta MP, Shapiro WR, Phan SC, Gervais R, Carrie C, Chabot P, et al. Motexafin Gadolinium Combined With Prompt Whole Brain Radiotherapy Prolongs Time to Neurologic Progression in Non-Small-Cell Lung Cancer Patients With Brain Metastases: Results of a Phase III Trial. Int J Radiat Oncol Biol Phys (2009) 73:1069–76. doi: 10.1016/j.ijrobp.2008.05.068
88. Suh JH, Stea B, Nabid A, Kresl JJ, Fortin A, Mercier J-P, et al. Phase III Study of Efaproxiral as an Adjunct to Whole-Brain Radiation Therapy for Brain Metastases. J Clin Oncol (2006) 24:106–14. doi: 10.1200/JCO.2004.00.1768
89. Kazda T, Jancalek R, Pospisil P, Sevela O, Prochazka T, Vrzal M, et al. Why and How to Spare the Hippocampus During Brain Radiotherapy: The Developing Role of Hippocampal Avoidance in Cranial Radiotherapy. Radiat Oncol (2014) 9:139. doi: 10.1186/1748-717X-9-139
90. Eriksson PS, Perfilieva E, Björk-Eriksson T, Alborn AM, Nordborg C, Peterson DA, et al. Neurogenesis in the Adult Human Hippocampus. Nat Med (1998) 4:1313–7. doi: 10.1038/3305
91. Nagai R, Tsunoda S, Hori Y, Asada H. Selective Vulnerability to Radiation in the Hippocampal Dentate Granule Cells. Surg Neurol (2000) 53:503–6. doi: 10.1016/s0090-3019(00)00214-7
92. Gondi V, Pugh SL, Tome WA, Caine C, Corn B, Kanner A, et al. Preservation of Memory With Conformal Avoidance of the Hippocampal Neural Stem-Cell Compartment During Whole-Brain Radiotherapy for Brain Metastases (RTOG 0933): A Phase II Multi-Institutional Trial. J Clin Oncol (2014) 32:3810–6. doi: 10.1200/JCO.2014.57.2909
93. Soffietti R, Kocher M, Abacioglu UM, Villa S, Fauchon F, Baumert BG, et al. A European Organisation for Research and Treatment of Cancer Phase III Trial of Adjuvant Whole-Brain Radiotherapy Versus Observation in Patients With One to Three Brain Metastases From Solid Tumors After Surgical Resection or Radiosurgery: Quality-of-Life Results. J Clin Oncol (2013) 31:65–72. doi: 10.1200/JCO.2011.41.0639
94. Gondi V, Tolakanahalli R, Mehta MP, Tewatia D, Rowley H, Kuo JS, et al. Hippocampal-Sparing Whole-Brain Radiotherapy: A “How-to” Technique Using Helical Tomotherapy and Linear Accelerator-Based Intensity-Modulated Radiotherapy. Int J Radiat Oncol Biol Phys (2010) 78:1244–52. doi: 10.1016/j.ijrobp.2010.01.039
95. Brown PD, Gondi V, Pugh S, Tome WA, Wefel JS, Armstrong TS, et al. Hippocampal Avoidance During Whole-Brain Radiotherapy Plus Memantine for Patients With Brain Metastases: Phase III Trial NRG Oncology Cc001. J Clin Oncol (2020) 38:1019–29. doi: 10.1200/JCO.19.02767
96. Baschnagel AM, Meyer KD, Chen PY, Krauss DJ, Olson RE, Pieper DR, et al. Tumor Volume as a Predictor of Survival and Local Control in Patients With Brain Metastases Treated With Gamma Knife Surgery. J Neurosurg (2013) 119:1139–44. doi: 10.3171/2013.7.JNS13431
97. de Azevedo Santos TR, Tundisi CF, Ramos H, Maia MAC, Pellizzon ACA, Silva MLG, et al. Local Control After Radiosurgery for Brain Metastases: Predictive Factors and Implications for Clinical Decision. Radiat Oncol (2015) 10:63. doi: 10.1186/s13014-015-0367-y
98. Chang WS, Kim HY, Chang JW, Park YG, Chang JH. Analysis of Radiosurgical Results in Patients With Brain Metastases According to the Number of Brain Lesions: Is Stereotactic Radiosurgery Effective for Multiple Brain Metastases? J Neurosurg (2010) 113 Suppl:73–8. doi: 10.3171/2010.8.GKS10994
99. Bhatnagar AK, Flickinger JC, Kondziolka D, Lunsford LD. Stereotactic Radiosurgery for Four or More Intracranial Metastases. Int J Radiat Oncol Biol Phys (2006) 64:898–903. doi: 10.1016/j.ijrobp.2005.08.035
100. Yamamoto M, Kawabe T, Sato Y, Higuchi Y, Nariai T, Watanabe S, et al. Stereotactic Radiosurgery for Patients With Multiple Brain Metastases: A Case-Matched Study Comparing Treatment Results for Patients With 2-9 Versus 10 or More Tumors. J Neurosurg (2014) 121 Suppl:16–25. doi: 10.3171/2014.8.GKS141421
101. Yamamoto M, Serizawa T, Shuto T, Akabane A, Higuchi Y, Kawagishi J, et al. Stereotactic Radiosurgery for Patients With Multiple Brain Metastases (JLGK0901): A Multi-Institutional Prospective Observational Study. Lancet Oncol (2014) 15:387–95. doi: 10.1016/S1470-2045(14)70061-0
102. Nichol A, Ma R, Hsu F, Gondara L, Carolan H, Olson R, et al. Volumetric Radiosurgery for 1 to 10 Brain Metastases: A Multicenter, Single-Arm, Phase 2 Study. Int J Radiat Oncol Biol Phys (2016) 94:312–21. doi: 10.1016/j.ijrobp.2015.10.017
103. Pfeffer RM, Levin D, Spiegelmann R. Linac-Based Radiosurgery for Multiple Brain Metastases: A Quality Assurance and Feasibility Study. J Clin Oncol (2017) 35 Suppl:2077. doi: 10.1200/JCO.2017.35.15_suppl.2077
104. Yamamoto M, Sato Y, Higuchi Y, Kasuya H, Barfod BE. A Cohort Study of Stereotactic Radiosurgery Results for Patients With 5 to 15 Versus 2 to 4 Brain Metastatic Tumors. Adv Radiat Oncol (2020) 5:358–68. doi: 10.1016/j.adro.2019.11.001
105. Hughes RT, McTyre ER, LeCompte M, Cramer CK, Munley MT, Laxton AW, et al. Clinical Outcomes of Upfront Stereotactic Radiosurgery Alone for Patients With 5 to 15 Brain Metastases. Neurosurgery (2019) 85:257–63. doi: 10.1093/neuros/nyy276
106. Hartgerink D, Bruynzeel A, Eekers D, Swinnen A, Hurkmans C, Wiggenraad R, et al. A Dutch Phase III Randomized Multicenter Trial: Whole Brain Radiotherapy Versus Stereotactic Radiotherapy for 4-10 Brain Metastases. Neurooncol Adv (2021) 3:vdab021. doi: 10.1093/noajnl/vdab021
107. Li J, Ludmir EB, Wang Y, Guha-Thakurta N, McAleer MF, Settle SH Jr, et al. Stereotactic Radiosurgery Versus Whole-Brain Radiation Therapy for Patients With 4-15 Brain Metastases: A Phase III Randomized Controlled Trial. Int J Radiat Oncol Biol Phys (2020) 108 Suppl:S21–2. doi: 10.1016/j.ijrobp.2020.07.2108
108. Kirakli EK, Yilmaz U. Radiotherapy of Brain Metastases From Non-Small Cell Lung Cancer. J Cancer Metastasis Treat (2019) 10:5. doi: 10.20517/2394-4722.2018.73
109. Dobi Á, Fodor E, Maráz A, Együd Z, Cserháti A, Tiszlavicz L, et al. Boost Irradiation Integrated to Whole Brain Radiotherapy in the Management of Brain Metastases. Pathol Oncol Res (2020) 26:149–57. doi: 10.1007/s12253-018-0383-y
110. Lagerwaard FJ, van der Hoorn EAP, Verbakel WFAR, Haasbeek CJA, Slotman BJ, Senan S. Whole-Brain Radiotherapy With Simultaneous Integrated Boost to Multiple Brain Metastases Using Volumetric Modulated Arc Therapy. Int J Radiat Oncol Biol Phys (2009) 75:253–9. doi: 10.1016/j.ijrobp.2009.03.029
111. Rodrigues G, Zindler J, Warner A, Bauman G, Senan S, Lagerwaard F. Propensity-Score Matched Pair Comparison of Whole Brain With Simultaneous in-Field Boost Radiotherapy and Stereotactic Radiosurgery. Radiother Oncol (2013) 106:206–9. doi: 10.1016/j.radonc.2012.10.014
112. Zhou L, Liu J, Xue J, Xu Y, Gong Y, Deng L, et al. Whole Brain Radiotherapy Plus Simultaneous in-Field Boost With Image Guided Intensity-Modulated Radiotherapy for Brain Metastases of Non-Small Cell Lung Cancer. Radiat Oncol (2014) 9:117. doi: 10.1186/1748-717X-9-117
113. Zhong J, Waldman AD, Kandula S, Eaton BR, Prabhu RS, Huff SB, et al. Outcomes of Whole-Brain Radiation With Simultaneous in-Field Boost (SIB) for the Treatment of Brain Metastases. J Neurooncol (2020) 147:117–23. doi: 10.1007/s11060-020-03405-y
114. Lin B, Huang D, Du H, Fan J, Zhang Y, Feng G, et al. Whole-Brain Radiation Therapy With Simultaneous Integrated Boost Versus Whole-Brain Radiation Therapy Plus Stereotactic Radiosurgery for the Treatment of Brain Metastasis From Lung Cancer. Front Oncol (2021) 11:631422. doi: 10.3389/fonc.2021.631422
115. Du T-Q, Li X, Zhong W-S, Tian J-D, Zhao Y-X, Liu D. Brain Metastases of Lung Cancer: Comparison of Survival Outcomes Among Whole Brain Radiotherapy, Whole Brain Radiotherapy With Consecutive Boost, and Simultaneous Integrated Boost. J Cancer Res Clin Oncol (2021) 147:569–77. doi: 10.1007/s00432-020-03359-8
116. Gutiérrez AN, Westerly DC, Tomé WA, Jaradat HA, Mackie TR, Bentzen SM, et al. Whole Brain Radiotherapy With Hippocampal Avoidance and Simultaneously Integrated Brain Metastases Boost: A Planning Study. Int J Radiat Oncol Biol Phys (2007) 69:589–97. doi: 10.1016/j.ijrobp.2007.05.038
117. Hsu F, Carolan H, Nichol A, Cao F, Nuraney N, Lee R, et al. Whole Brain Radiotherapy With Hippocampal Avoidance and Simultaneous Integrated Boost for 1-3 Brain Metastases: A Feasibility Study Using Volumetric Modulated Arc Therapy. Int J Radiat Oncol Biol Phys (2010) 76:1480–5. doi: 10.1016/j.ijrobp.2009.03.032
118. Popp I, Rau S, Hintz M, Schneider J, Bilger A, Fennell JT, et al. Hippocampus-Avoidance Whole-Brain Radiation Therapy With a Simultaneous Integrated Boost for Multiple Brain Metastases. Cancer (2020) 126:2694–703. doi: 10.1002/cncr.32787
119. Grosu A-L, Frings L, Bentsalo I, Oehlke O, Brenner F, Bilger A, et al. Whole-Brain Irradiation With Hippocampal Sparing and Dose Escalation on Metastases: Neurocognitive Testing and Biological Imaging (HIPPORAD) - a Phase II Prospective Randomized Multicenter Trial (NOA-14, ARO 2015-3, DKTK-ROG). BMC Cancer (2020) 20:532. doi: 10.1186/s12885-020-07011-z
120. Chia BSH, Leong JY, Ong ALK, Lim C, Poon SH, Chua MLK, et al. Randomised Prospective Phase II Trial in Multiple Brain Metastases Comparing Outcomes Between Hippocampal Avoidance Whole Brain Radiotherapy With or Without Simultaneous Integrated Boost: HA-SIB-WBRT Study Protocol. BMC Cancer (2020) 20:1045. doi: 10.1186/s12885-020-07565-y
121. Le Rhun E, Weller M, Brandsma D, Van den Bent M, de Azambuja E, Henriksson R, et al. EANO-ESMO Clinical Practice Guidelines for Diagnosis, Treatment and Follow-Up of Patients With Leptomeningeal Metastasis From Solid Tumours. Ann Oncol (2017) 28:iv84–99. doi: 10.1093/annonc/mdx221
122. Johnson MD, Avkshtol V, Baschnagel AM, Meyer K, Ye H, Grills IS, et al. Surgical Resection of Brain Metastases and the Risk of Leptomeningeal Recurrence in Patients Treated With Stereotactic Radiosurgery. Int J Radiat Oncol Biol Phys (2016) 94:537–43. doi: 10.1016/j.ijrobp.2015.11.022
123. Gainor JF, Sherman CA, Willoughby K, Logan J, Kennedy E, Brastianos PK, et al. Alectinib Salvages CNS Relapses in ALK-Positive Lung Cancer Patients Previously Treated With Crizotinib and Ceritinib. J Thorac Oncol (2015) 10:232–6. doi: 10.1097/JTO.0000000000000455
124. Yang JCH, Kim S-W, Kim D-W, Lee J-S, Cho BC, Ahn J-S, et al. Osimertinib in Patients With Epidermal Growth Factor Receptor Mutation-Positive Non-Small-Cell Lung Cancer and Leptomeningeal Metastases: The BLOOM Study. J Clin Oncol (2020) 38:538–47. doi: 10.1200/JCO.19.00457
125. Ahn M-J, Chiu C-H, Cheng Y, Han J-Y, Goldberg SB, Greystoke A, et al. Osimertinib for Patients With Leptomeningeal Metastases Associated With EGFR T790M-Positive Advanced NSCLC: The AURA Leptomeningeal Metastases Analysis. J Thorac Oncol (2020) 15:637–48. doi: 10.1016/j.jtho.2019.12.113
126. Zheng M-M, Tu H-Y, Yang J-J, Zhang X-C, Zhou Q, Xu C-R, et al. Clinical Outcomes of non-Small Cell Lung Cancer Patients With Leptomeningeal Metastases After Immune Checkpoint Inhibitor Treatments. Eur J Cancer (2021) 150:23–30. doi: 10.1016/j.ejca.2021.03.037
127. Morris PG, Reiner AS, Szenberg OR, Clarke JL, Panageas KS, Perez HR, et al. Leptomeningeal Metastasis From Non-Small Cell Lung Cancer: Survival and the Impact of Whole Brain Radiotherapy. J Thorac Oncol (2012) 7:382–5. doi: 10.1097/JTO.0b013e3182398e4f
128. Kuiper JL, Hendriks LE, van der Wekken AJ, de Langen AJ, Bahce I, Thunnissen E, et al. Treatment and Survival of Patients With EGFR-Mutated Non-Small Cell Lung Cancer and Leptomeningeal Metastasis: A Retrospective Cohort Analysis. Lung Cancer (2015) 89:255–61. doi: 10.1016/j.lungcan.2015.05.023
129. Le Rhun E, Rudà R, Devos P, Hoang-Xuan K, Brandsma D, Pérez Segura P, et al. Diagnosis and Treatment Patterns for Patients With Leptomeningeal Metastasis From Solid Tumors Across Europe. J Neurooncol (2017) 133:419–27. doi: 10.1007/s11060-017-2452-6
130. Prabhu RS, Turner BE, Asher AL, Marcrom SR, Fiveash JB, Foreman PM, et al. A Multi-Institutional Analysis of Presentation and Outcomes for Leptomeningeal Disease Recurrence After Surgical Resection and Radiosurgery for Brain Metastases. Neuro Oncol (2019) 21:1049–59. doi: 10.1093/neuonc/noz049
131. Buszek SM, Chung C. Radiotherapy in Leptomeningeal Disease: A Systematic Review of Randomized and Non-Randomized Trials. Front Oncol (2019) 9:1224. doi: 10.3389/fonc.2019.01224
132. Hirano Y, Konishi K, Ejima Y. Utility of Whole Brain Radiation Therapy for Leptomeningeal Carcinomatosis. Int J Clin Oncol (2020) 25:1432–9. doi: 10.1007/s10147-020-01668-z
133. Chen L, Douglass J, Kleinberg L, Ye X, Marciscano AE, Forde PM, et al. Concurrent Immune Checkpoint Inhibitors and Stereotactic Radiosurgery for Brain Metastases in Non-Small Cell Lung Cancer, Melanoma, and Renal Cell Carcinoma. Int J Radiat Oncol Biol Phys (2018) 100:916–25. doi: 10.1016/j.ijrobp.2017.11.041
134. Jablonska PA, Bosch-Barrera J, Serrano D, Valiente M, Calvo A, Aristu J. Challenges and Novel Opportunities of Radiation Therapy for Brain Metastases in Non-Small Cell Lung Cancer. Cancers (Basel) (2021) 13:2141. doi: 10.3390/cancers13092141
135. Borius P-Y, Régis J, Carpentier A, Kalamarides M, Valery CA, Latorzeff I. Safety of Radiosurgery Concurrent With Systemic Therapy (Chemotherapy, Targeted Therapy, and/or Immunotherapy) in Brain Metastases: A Systematic Review. Cancer Metastasis Rev (2021) 40:341–54. doi: 10.1007/s10555-020-09949-9
136. Cho A, Untersteiner H, Hirschmann D, Shaltout A, Göbl P, Dorfer C, et al. Gamma Knife Radiosurgery for Brain Metastases in Non-Small Cell Lung Cancer Patients Treated With Immunotherapy or Targeted Therapy. Cancers (Basel) (2020) 12:E3668. doi: 10.3390/cancers12123668
137. Welsh JW, Komaki R, Amini A, Munsell MF, Unger W, Allen PK, et al. Phase II Trial of Erlotinib Plus Concurrent Whole-Brain Radiation Therapy for Patients With Brain Metastases From Non-Small-Cell Lung Cancer. J Clin Oncol (2013) 31:895–902. doi: 10.1200/JCO.2011.40.1174
138. Zhuang H, Yuan Z, Wang J, Zhao L, Pang Q, Wang P. Phase II Study of Whole Brain Radiotherapy With or Without Erlotinib in Patients With Multiple Brain Metastases From Lung Adenocarcinoma. Drug Des Devel Ther (2013) 7:1179–86. doi: 10.2147/DDDT.S53011
139. Yang Z, Zhang Y, Li R, Yisikandaer A, Ren B, Sun J, et al. Whole-Brain Radiotherapy With and Without Concurrent Erlotinib in NSCLC With Brain Metastases: A Multicenter, Open-Label, Randomized, Controlled Phase III Trial. Neuro Oncol (2021) 23:967–78. doi: 10.1093/neuonc/noaa281
140. Chen Y, Wei J, Cai J, Liu A. Combination Therapy of Brain Radiotherapy and EGFR-TKIs Is More Effective Than TKIs Alone for EGFR-Mutant Lung Adenocarcinoma Patients With Asymptomatic Brain Metastasis. BMC Cancer (2019) 19:793. doi: 10.1186/s12885-019-6005-6
141. Costa DB, Shaw AT, Ou S-HI, Solomon BJ, Riely GJ, Ahn M-J, et al. Clinical Experience With Crizotinib in Patients With Advanced ALK-Rearranged Non-Small-Cell Lung Cancer and Brain Metastases. J Clin Oncol (2015) 33:1881–8. doi: 10.1200/JCO.2014.59.0539
142. Johung KL, Yeh N, Desai NB, Williams TM, Lautenschlaeger T, Arvold ND, et al. Extended Survival and Prognostic Factors for Patients With ALK-Rearranged Non-Small-Cell Lung Cancer and Brain Metastasis. J Clin Oncol (2016) 34:123–9. doi: 10.1200/JCO.2015.62.0138
143. Soria J-C, Tan DSW, Chiari R, Wu Y-L, Paz-Ares L, Wolf J, et al. First-Line Ceritinib Versus Platinum-Based Chemotherapy in Advanced ALK-Rearranged Non-Small-Cell Lung Cancer (ASCEND-4): A Randomised, Open-Label, Phase 3 Study. Lancet (2017) 389:917–29. doi: 10.1016/S0140-6736(17)30123-X
144. Lorger M. Tumor Microenvironment in the Brain. Cancers (Basel) (2012) 4:218–43. doi: 10.3390/cancers4010218
145. Samstein R, Rimner A, Barker C, Yamada Y. Combined Immune Checkpoint Blockade and Radiation Therapy: Timing and Dose Fractionation Associated With Greatest Survival Duration Among Over 750 Treated Patients. Int J Radiat Oncol Biol Phys (2017) 99 Suppl:S129–30. doi: 10.1016/j.ijrobp.2017.06.303
146. Ahmed KA, Kim S, Arrington J, Naghavi AO, Dilling TJ, Creelan BC, et al. Outcomes Targeting the PD-1/PD-L1 Axis in Conjunction With Stereotactic Radiation for Patients With Non-Small Cell Lung Cancer Brain Metastases. J Neurooncol (2017) 133:331–8. doi: 10.1007/s11060-017-2437-5
147. Hubbeling HG, Schapira EF, Horick NK, Goodwin KEH, Lin JJ, Oh KS, et al. Safety of Combined PD-1 Pathway Inhibition and Intracranial Radiation Therapy in Non-Small Cell Lung Cancer. J Thorac Oncol (2018) 13:550–8. doi: 10.1016/j.jtho.2018.01.012
148. Levy A, Faivre-Finn C, Hasan B, De Maio E, Berghoff AS, Girard N, et al. Diversity of Brain Metastases Screening and Management in Non-Small Cell Lung Cancer in Europe: Results of the European Organisation for Research and Treatment of Cancer Lung Cancer Group Survey. Eur J Cancer (2018) 93:37–46. doi: 10.1016/j.ejca.2018.01.067
149. Aupérin A, Arriagada R, Pignon JP, Le Péchoux C, Gregor A, Stephens RJ, et al. Prophylactic Cranial Irradiation for Patients With Small-Cell Lung Cancer in Complete Remission. Prophylactic Cranial Irradiation Overview Collaborative Group. N Engl J Med (1999) 341:476–84. doi: 10.1056/NEJM199908123410703
150. Cox JD, Stanley K, Petrovich Z, Paig C, Yesner R. Cranial Irradiation in Cancer of the Lung of All Cell Types. JAMA (1981) 245:469–72. doi: 10.1001/jama.1981.03310300023013
151. Li N, Zeng Z-F, Wang S-Y, Ou W, Ye X, Li J, et al. Randomized Phase III Trial of Prophylactic Cranial Irradiation Versus Observation in Patients With Fully Resected Stage IIIA-N2 Nonsmall-Cell Lung Cancer and High Risk of Cerebral Metastases After Adjuvant Chemotherapy. Ann Oncol (2015) 26:504–9. doi: 10.1093/annonc/mdu567
152. Russell AH, Pajak TE, Selim HM, Paradelo JC, Murray K, Bansal P, et al. Prophylactic Cranial Irradiation for Lung Cancer Patients at High Risk for Development of Cerebral Metastasis: Results of a Prospective Randomized Trial Conducted by the Radiation Therapy Oncology Group. Int J Radiat Oncol Biol Phys (1991) 21:637–43. doi: 10.1016/0360-3016(91)90681-s
153. Gore EM, Bae K, Wong SJ, Sun A, Bonner JA, Schild SE, et al. Phase III Comparison of Prophylactic Cranial Irradiation Versus Observation in Patients With Locally Advanced non-Small-Cell Lung Cancer: Primary Analysis of Radiation Therapy Oncology Group Study RTOG 0214. J Clin Oncol (2011) 29:272–8. doi: 10.1200/JCO.2010.29.1609
154. Precival C, Landy M, Poole C, Mullaney L. The Role of Prophylactic Cranial Irradiation for Non-Small Cell Lung Cancer. Anticancer Res (2018) 38:7–14. doi: 10.21873/anticanres.12185
155. De Ruysscher D, Dingemans A-MC, Praag J, Belderbos J, Tissing-Tan C, Herder J, et al. Prophylactic Cranial Irradiation Versus Observation in Radically Treated Stage III Non-Small-Cell Lung Cancer: A Randomized Phase III NVALT-11/DLCRG-02 Study. J Clin Oncol (2018) 36:2366–77. doi: 10.1200/JCO.2017.77.5817
Keywords: brain metastases, radiosurgery, SRS, WBRT (whole brain radiotherapy), modern radiotherapy, leptomeningeal dissemination, NSCLC, hippocampal avoidance
Citation: Mantovani C, Gastino A, Cerrato M, Badellino S, Ricardi U and Levis M (2021) Modern Radiation Therapy for the Management of Brain Metastases From Non-Small Cell Lung Cancer: Current Approaches and Future Directions. Front. Oncol. 11:772789. doi: 10.3389/fonc.2021.772789
Received: 08 September 2021; Accepted: 18 October 2021;
Published: 02 November 2021.
Edited by:
Tiziana Vavala, SC of Oncology ASL CN1, ItalyReviewed by:
Timothy F. Burns, University of Pittsburgh, United StatesHeloisa De Andrade Carvalho, Universidade de São Paulo, Brazil
Copyright © 2021 Mantovani, Gastino, Cerrato, Badellino, Ricardi and Levis. This is an open-access article distributed under the terms of the Creative Commons Attribution License (CC BY). The use, distribution or reproduction in other forums is permitted, provided the original author(s) and the copyright owner(s) are credited and that the original publication in this journal is cited, in accordance with accepted academic practice. No use, distribution or reproduction is permitted which does not comply with these terms.
*Correspondence: Mario Levis, mario.levis@unito.it