- 1Institute of Innovation, Science and Sustainability, Future Regions Research Centre, Federation University, Berwick, VIC, Australia
- 2Institute of Geography and Agroecology, Chinese Academy of Sciences, Changchun, China
- 3Institute of Innovation, Science and Sustainability, Future Regions Research Centre, Federation University, Ballarat, VIC, Australia
Cassytha, also known as laurel dodder or love vine, is a stem hemiparasite of the Lauraceae family. It has long been used for medicinal purposes in many countries and has increasingly influenced agricultural and natural ecosystems by its effects on a wide range of host species. Previous studies have focused on the taxonomy and evolutionary position of different Cassytha, with the pan-tropical species Cassytha filiformis being the most widely studied. However, Cassytha–host interactions have never been reviewed, which is an essential issue related to the understanding of mechanisms underlying plant hemiparasitic and the assessment of benefits and damage caused by aerial parasitic plants. This review explores the parasitic habits, worldwide distribution, and host range of Cassytha, and examines its impacts on the biology of host plants and the overall influence of environmental changes on Cassytha–host associations. We also comment on areas of future research directions that require to better understanding Cassytha–host interactions. It appeared that some traits, such as flowering phenology, facilitated Cassytha’s widespread distribution and successful parasitism and that Cassytha preferred woody species rather than herbaceous species as a host, and preferred species from certain families as hosts, such as Fabaceae and Myrtaceae. Cassytha often decreased biomass and impacted the physiology of host species and global environmental changes seemed to intensify the negative impacts of Cassytha on their hosts. Cassytha was not only a noxious weed, but can also function as a biocontrol agent to mitigate alien plant invasion.
Introduction
Parasitism is a widespread phenomenon and an important ecological interaction, with many organisms being engaged as either parasites or hosts (Combes, 2001; Krasylenko et al., 2021). Plant parasitism includes directly parasitizing host plants and absorbing water and nutrition via haustorium and indirectly parasitizing other plants and acquiring nutrition via mycorrhizal fungi (Nickrent and Musselman, 2004; Nickrent, 2014; Krasylenko et al., 2021). These parasitic flowering plants include about 4,500 species and have been divided into 12 independent evolutionary lineages (Nickrent, 2020; Těšitel et al., 2021). Parasitic flowering plants either attach to host roots or shoots. They can be chlorophyllous and thus, capable of photosynthesis (hemiparasites) or not (holoparasites) and they can be further divided into four types: root vs. stem hemiparasites, and root vs. stem holoparasites (Musselman and Press, 1995; Teixeira-Costa and Davis, 2021). They can also be classified as obligate parasites (which indicates that they need a host plant to acquire nutrients to survive after germination) and facultative parasites (which are capable of reaching maturity without attachment to the host) (Shen et al., 2006). According to studies of functional diversity, parasitic flowering plants have been recognized as euphytoid parasites (resembling true plants that are capable of photosynthesis while infesting the underground root system of their hosts), mistletoes (shrubby plants with seeds that germinate autonomously and directly upon the branches of their hosts), parasitic vines (i.e., Cassytha and Cuscuta), obligate root parasites (parasites that germinate underground, often in response to host-derived chemicals, and infest the root systems of their hosts) and endoparasites (vegetative body of parasitic plants is reduced to mycelial-like strands of cells embedded within their respective host roots or stems) (Teixeira-Costa and Davis, 2021).
Parasitic flowering plants have had renewed attention over the past three decades (Nickrent, 2020), since they caused serious problems across a wide range of major ecosystems, from subarctic tundra, heathlands, savanna woodlands, deserts, temperate and tropical forests, and agricultural ecosystem (Press and Phoenix, 2005; Shen et al., 2006). Concurrently, the parasitic plants have medicinal and cultural values (Těšitel et al., 2021) and play important roles as keystone species (species that exert a disproportionate impact on community biodiversity relative to its presence in a community; Paine, 1969; Miller et al., 2003; Caraballo-Ortiz, 2019; Těšitel et al., 2021), and ecosystem engineers (species that directly and indirectly shift the availability of resources to other species in the community) in these habitats (Jones et al., 1994; Press and Phoenix, 2005; Bardgett et al., 2006; Spasojevic and Suding, 2011). Despite a large body of research on the biology of root hemiparasites regarding the Scrophulariaceae and Santalaceae species, plus, mistletoes of families Loranthaceae and Viscaceae, and the stem holoparasites Cuscuta (Tennakoon et al., 1997; Lanini and Kogan, 2005; Phoenix and Press, 2005; Carnegie et al., 2009; Glatzel and Geils, 2009; Mishra, 2009; Furuhashi et al., 2011; Clarke et al., 2019), a notable exception is stem hemiparasitic genus Cassytha. Being stem-parasitic vines, Cassytha and Cuscuta behave similarly and are often referred to together or represented inadvertently as Cuscuta (Weber, 1981; Mishra, 2009). However, Cassytha is a hemiparasite whilst Cuscuta is a holoparasite, and they actually differ in many aspects such as the action of the haustorium, their stem appearance, and life span (Tennakoon et al., 2016; Těšitel, 2016; Teixeira-Costa and Davis, 2021). Study on Cassytha has been relatively neglected, leading to it being less well characterized compared to its companion Cuscuta (Lanini and Kogan, 2005; Mishra, 2009).
Cassytha, belongs to the sub-family Cassythoideae, the family Lauraceae and the magnoliid clade (Awang et al., 2018). Cassytha filiformis has been exploited for medicines, cosmetics, rope-making, and cushioning in the Pacific Islands (Whistler, 1992), and is treated as an important medicinal plant both in China (Huang et al., 2021) and Nigeria (Ambi et al., 2017). C. filiformis and Cassytha glabella have been treated as sources of bush tucker and medicines by the Australian Aboriginals (Levitt, 1981), and Cassytha pubescens has the potential to be used as a biocontrol agent for alien invasive species in southern Australia (Těšitel et al., 2020). Cassytha pondoensis is recognized as a medicinal plant in Angola (Novotna et al., 2020) whilst C. pubescens, Cassytha melantha, Cassytha racemosa, Cassytha pomiformis, and C. filiformis contain alkaloids (Collins et al., 1990) and C. filiformis, C. pubescens, and Cassytha capillaris contain essential oils (Brophy et al., 2009). The Cassytha grouping contains 19 species (Table 1) according to The Plant List1, 16 of which occur in Australia. There are 13 species endemic to Australia, one being pantropical (C. filiformis), one extending into Assam, Borneo, Lesser Sunda Islands, Malulu, New guinea, and Vietnam (C. capillaris), and one also being found in New Zealand (C. pubescens). The other three species are endemic to Africa (Cassytha ciliolata and C. pondoensis) or Thailand (Cassytha larsenii) (see Weber, 1981; Weber, 2007; Kokubugata et al., 2012; Nickrent, 2020). It has been reported that C. capillaris also occurs in Indonesia and China (Song et al., 2017; Liu et al., 2021), which has not been confirmed. Kokubugata et al. (2012) claimed that Cassytha pergracilis was an endemic species found in Japan (Kokubugata and Yokota, 2012). However, it is not recorded in Global Biodiversity Information Facility2 and is recognized as a synonym of C. glabella in The Plant List. Cassytha muelleri, Cassytha paniculata, and Cassytha phaeolasia were recorded as species in Weber (2007) and in the Flora of Australia3 that follows the Australian Plant Census4, but they are treated as synonyms of C. racemosa and C. pubescens, respectively (Supplementary Appendix Table S1).
As a widespread pan-tropical species, C. filiformis has been more extensively studied than other species of this genus. However, a group of scientists from South Australia has recently investigated the potential of using native C. pubescens to control the alien invasive shrubs Ulex europaeus and Cytisus scoparius (Cirocco et al., 2016a, 2017, 2018; Facelli et al., 2020). For other species in the Cassytha genus, there are relatively a few taxonomic studies and field investigations concentrating on species in certain habitats, with few empirical studies. For example, the cuticular character of all the Cassytha species and the stem and systematic anatomy of C. ciliolata, C. filiformis, C. glabella, C. melantha, and C. pubescens has been studied (Sastri, 1962; Beaman, 1971; Awang et al., 2018). The chlorophyll content and photosynthetic characteristics of C. ciliolata and C. filiformis in South Africa (De La Harpe et al., 1979, 1980, 1981) and the seasonal fluctuations in pigment chemistry of C. glabella and C. pubescens in Australia (Close et al., 2006) have also been investigated. However, the Cassytha–host interactions of any of these species have not been reviewed.
We suggest that a detailed interpretation of Cassytha–host interactions are important to allow an understanding of their complex interactive biology and to allow us to build up a relatively detailed picture of the ecophysiological behavior of these parasite–host associations. Further, parasites have a great impact on plant communities even though they might contribute a minor component in the mix, and a single parasite may seriously influence a large portion of an ecosystem (Press and Phoenix, 2005). Hence, the understanding of Cassytha–host interactions can also help to control the damage induced by these parasites in both agriculture and natural settings. In addition, it may be possible to utilize these stem parasites to control invasive weeds and use them separately for raw material and medicinal purposes (Těšitel et al., 2021). In this review, we summarize currently available information on Cassytha–host interactions focusing on its parasitic nature and worldwide distribution, identifying host range and preference, noting the impacts of Cassytha on host species and understanding the overall responses to the changes in climate, viable control strategies under heavy infestations and its sustainable utilization. We also identify gaps in current knowledge of this area and suggest future study directions deemed necessary for Cassytha–host interactions.
Parasitic Habits and Host Range of Cassytha
In this section, we mainly test whether particular habits facilitate the wide parasitism of Cassytha and if host species of certain life forms and/or hosts belonging to selected families are preferred.
Life History Habits
Cassytha (Figure 1) has twining stems with scaly leaves (Kuijt, 1969). Half of the Australian distributed species have evidence of flowering throughout the entire year (Cassytha aurea, Cassytha candida, C. capillaris, C. filiformis, Cassytha flava, C. glabella, C. racemosa, and Cassytha rufa), whilst the other half of the species are seasonally flowering. For example, C. melantha flowers from June to October (Weber, 1981, 2007). The fruit developments appear to be around 2 months, such as with Cassytha flindersii, but the information is scarce for other species (Weber, 2007). The dispersal of Cassytha is mainly dependent on seeds (Tennakoon et al., 2016), and the fruit is a drupe with a single seed and a white translucent, fleshy pericarp (Mishra, 2009). Thus, it is assumed that zoochory (e.g., dispersal by vertebrates) is important for the spread of Cassytha (French and Westoby, 1996). Recent experiments also provide evidence that mammals are involved in the dispersal of Cassytha pubescens (Maciunas et al., 2022). Some Cassytha species, such as C. filiformis, have refractory seeds with a hard seed coat and are found predominantly in coastal regions (Heide-Jørgensen, 2008; Mahadevan and Jayasuriya, 2013). It has been found that the fruits of C. filiformis floated for months in the Pacific (Muir, 1933). These lead to an additional water-mediated dispersal hypothesis for this species (Teixeira-Costa and Davis, 2021), but further evidence is lacking.
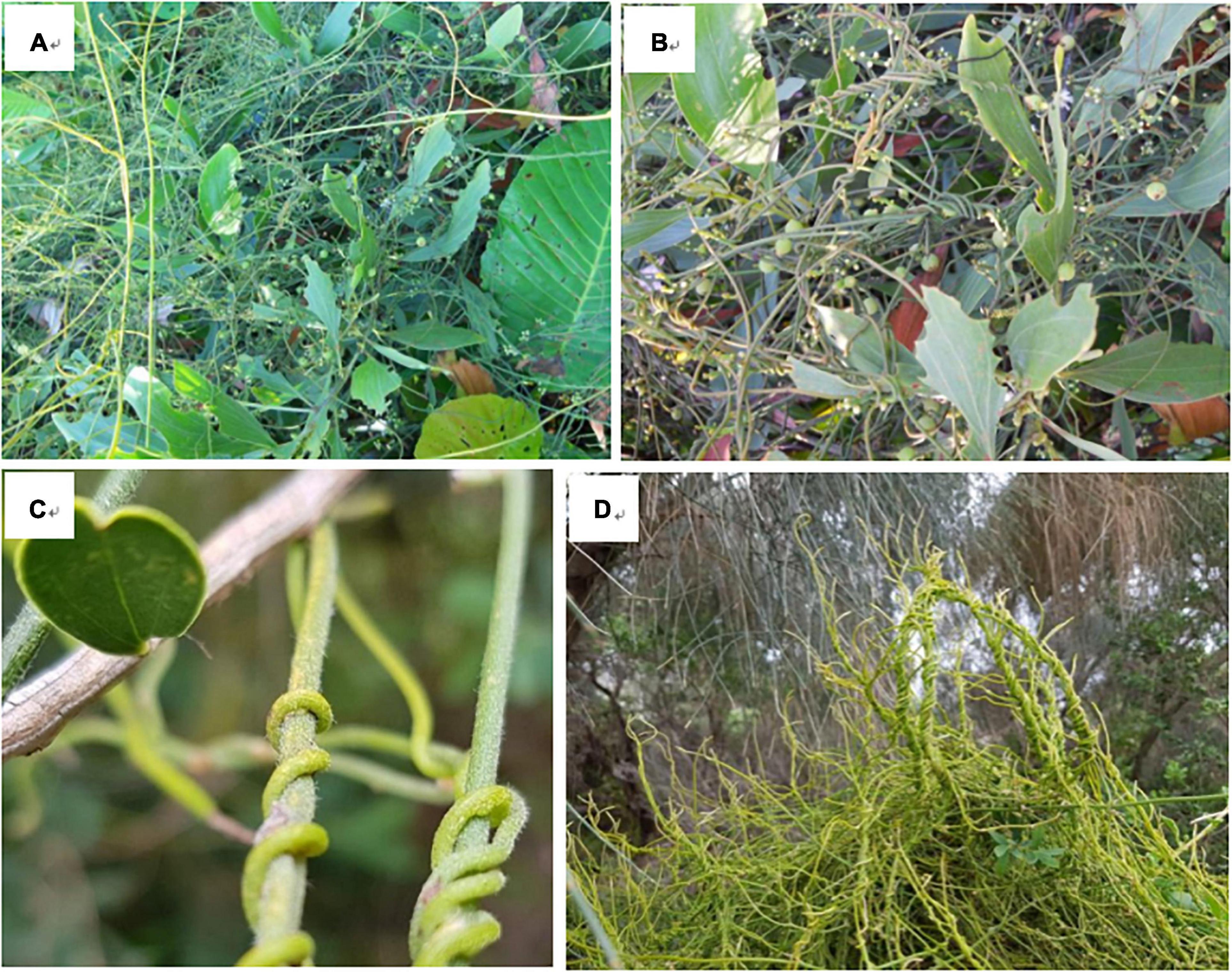
Figure 1. Images of Cassytha filiformis (A,B, in Brunei) and Cassytha pubescens (C,D, in Australia) on host species (source: KT).
Seeds of C. pubescens also have physical dormancy, which can be broken by heat and scarification of the husk, and the germination rate of heated seeds was found to be much higher than that of scarified seeds (Tsang, 2010). This suggests C. pubescens may have evolved fire-related germination cues with its native hosts (Tsang, 2010). Cassytha seeds germinate on the ground, with a rudimentary and short-lived root (McLuckie, 1924; Kuijt, 1969) and without needing any host influence. This independent (autotrophic) growth period is reported to be between one to a few weeks (McLuckie, 1924). C. filiformis seedlings can survive for more than 1 month prior to parasitizing a viable host, as long as there is photosynthesis, plus water and nutrient absorption from the soil by rudimentary roots. It can grow up to 30 cm in length without attaching to a host (Nelson, 2008; Furuhashi et al., 2016), even though Cassytha belongs to the obligate parasite class (Shen et al., 2010; Balasubramanian et al., 2014).
The flowering phenology, seed dispersal, and autotrophic habits before attaching to a host facilitate wide distribution and parasitism of Cassytha species. However, very little has been reported regarding the early life history of Cassytha, leaving us with a paucity of information related to the autotrophic stage of germinated Cassytha prior to establishment of successful attachments with hosts, and the cues involved with the stimulation of haustorial initiation from the Cassytha seedlings. It is thought, nevertheless, that all these growth and reproduction habits are closely related to host selection and parasite–host interactions.
Haustorial Development and Attachment Mechanisms
Cassytha can actively move their young stems to find suitable hosts (Tennakoon et al., 2016). It is not clear whether chemical cues released by hosts trigger Cassytha’s attachment to hosts, in a similar manner to Cuscuta foraging volatile substances from host plants (Furuhashi et al., 2016; Tennakoon et al., 2016). The attachment structure of Cassytha on hosts is by means of the haustorium, and a single Cassytha has been observed to produce hundreds of haustoria (Kuijt, 1969; Groom and Lamont, 2015). Haustoria are generally produced on young shoots or leaf rachises of the host plants (Werth et al., 1979). Twining is the critical first step of attachment, but less attention has been paid compared to haustorial development. Incident light and plant hormones have been shown to control tendril coiling in laboratory conditions (Furuhashi et al., 2021). Blue light and a lower far-red/red light (FR/R) ratio were noted to be essential for twining and subsequent haustorial induction of C. filiformis, respectively. Regarding plant hormones, seedlings of C. filiformis solely with auxin or cytokinin under blue light showed twining and haustorial induction. Seedlings with the hormones brassinolide and cytokinin showed twining even under dark conditions, but brassinolide acting alone did not stimulate twining (Furuhashi et al., 2021). This observation indicates that cytokinin and auxins may be the key hormones responsible in Cassytha to allow twining around hosts that facilitate subsequent haustorial initiation and successful Cassytha–host associations.
The swelling or cushion-like haustorium of Cassytha has two parts; the upper haustorium that lies external to the host and the endophyte that penetrates host tissues (Heide-Jørgensen, 1991). The vertical sections of the haustorium of C. filiformis on the leaves of host Canthium rheedii, included a vascular core, interrupted zone, collapsed layer, and clasping folds. Researchers have observed graniferous tracheary cells containing granules in the vascular core of the haustorium (Rajanna and Shivamurthy, 2001). When a stem of C. filiformis comes into contact with the compatible host stem, the cortical cells of the hemiparasite stem divide quickly and form the upper haustorium. The cells continually elongate and protuberate inside the host, modifying into finger-like digitate cells. The digitate cells break host stem cells with mechanical pressure and then differentiate into hypha-like lower endophyte structures (Balasubramanian et al., 2014). For example, in an association between C. filiformis and the host plant Morinda tinctoria, the link initially was slack and easy to be separated; however, subsequent formation of the endophyte and its ability to penetrate the host tissue facilitated a firm haustorial connection (Balasubramanian et al., 2014). Li and Yao (1992) studied the anatomical aspects of haustorial development of C. filiformis attached to a Salix purpurea stem. They divided the process into four stages: (i) polarity occurrence, (ii) cushion-shaped haustorial plate formation, (iii) haustorial (endophytic) primordium initiation in the cortex, with growth penetrating into the stem of the host, and finally (iv) tracheary element differentiation and connection with host’s vessels. Phloem sieve elements differentiation was not observed in this association. C. filiformis had developed xylem and degenerating phloem, which suggested mainly water and inorganic nutrition absorption of C. filiformis from the hosts such as S. purpurea (Li and Yao, 1992). It has been noted that the lack of phloem connections in haustoria with host plants is one of the substantial differences between the stem parasitic vines Cassytha and Cuscuta (Těšitel, 2016). However, in the parasitic interaction between C. filiformis and M. tinctoria, it has been reported that Cassytha haustoria have made contact with the phloem to obtain photosynthetic nutrients (Balasubramanian et al., 2014). The difference may be due to different host species or different infection stages, which needs well-coordinated further studies.
In addition to both mechanical and physical activities involved with the successful haustorial establishment of Cassytha with hosts, biochemical processes are also involved. The combination of these processes facilitates the quick, successful attachment of Cassytha haustoria to the conducting tissues of hosts. The penetrating haustoria of C. filiformis can release acid phosphatase (ACP) to injure host cells in conjunction with mechanical breakage of host cortical cells (Yao et al., 1994). Additionally, when twining on the host S. purpurea, the starch granules in C. filiformis stems have been seen to increase near the host end and further accumulated in the cells of critical regions along with haustorial development. After penetrating the host, starch granules gradually decrease and then disappear in the haustorium. The allocation and change of protein were contrary to that of the starch granules, indicating that when and where the starch granules decrease, the protein content increases and vice versa (Li and Yao, 1992). These results indicate that starch hydrolysis and protein synthesis provide matter and energy for cell division and other biochemical activities during haustorial development. Yao et al. (1994) have further suggested that haustorial development is closely correlated with the hormone cytokinin (CTK). The evidence for the above statement was found when haustoria of C. filiformis attached to the host Salix integra, and the isopentenyl adenine (iPa) and zeatin nucleotide (ZR) contents in the haustorial primordium initiation stage were observed to be much higher than those of the twining stage and penetrating stage.
Distribution and Host Range of Cassytha
Cassytha species are mainly distributed in tropics and subtropical regions (Figure 2), in coastal habitats and some species also in shrublands and forests (Table 1; Olson and Dinerstein, 2002). Cassytha is considered to be shade intolerant and is found to be best developed on relatively shorter trees and shrubs in open habitats, especially by roadsides and coastal vegetation (Werth et al., 1979). Cassytha is reported to parasitize a wide range of herbaceous and woody host species. But a survey conducted by Buriyo et al. (2015) in a cashew growing area in Tanzania has reported that C. filiformis has parasitized 75.4% of tree species followed by 23.2% of shrub species. In sharp contrast, herbaceous plants were rarely parasitized. We summarized 272 affirmatory host species of six Cassytha species from published literature covering 10 countries or regions, among which 226 are woody plants (Supplementary Appendix Table S2). This preference may accord with the perennial life form and hemiparasitic nature of C. filiformis. Herbaceous species might be bridging hosts that allow juvenile Cassytha to grow toward the perennial shrub or tree hosts in shrublands or forest ecosystems.
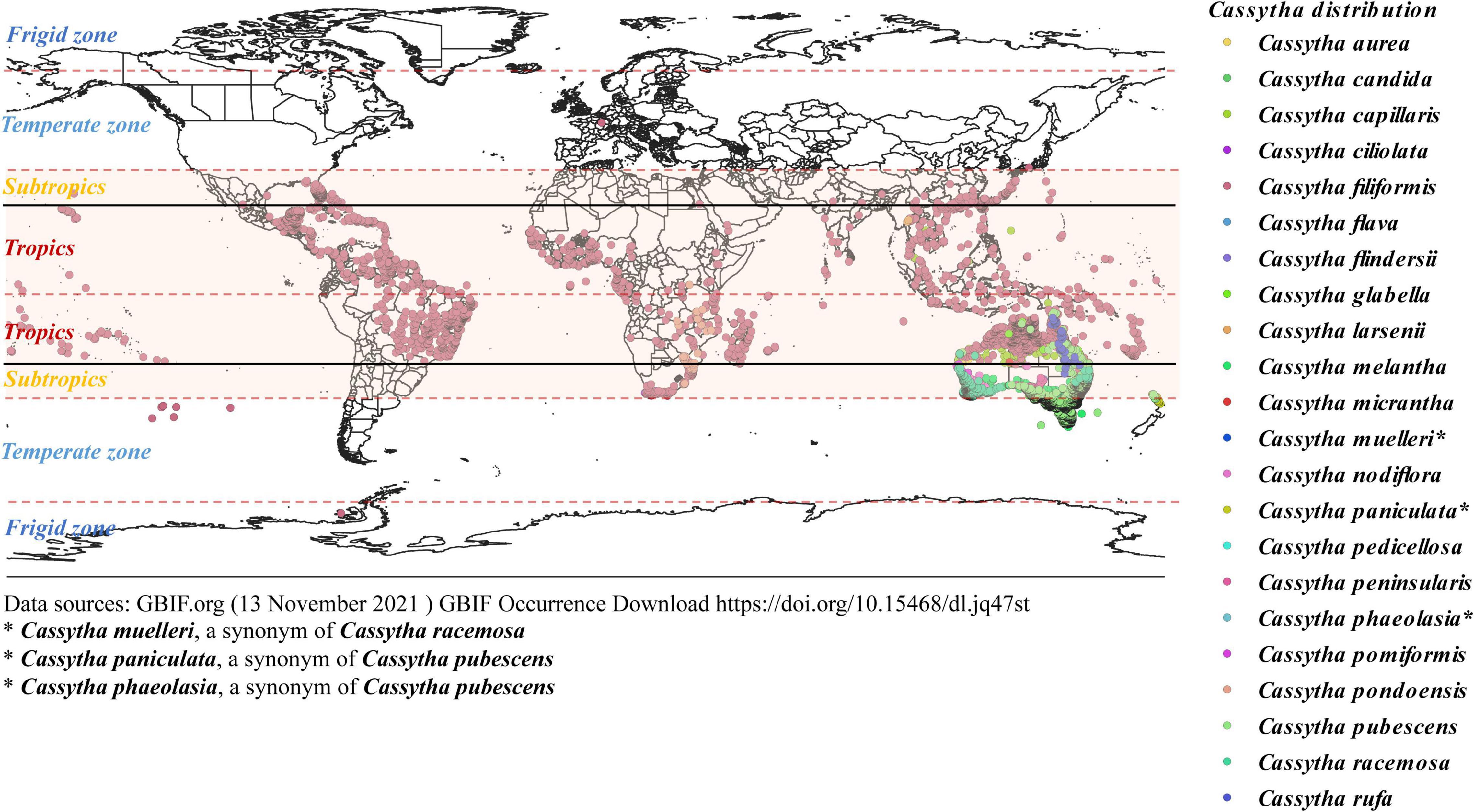
Figure 2. Global distribution map of Cassytha species across climatic zones. We modified the GBIF map according to published literature and POWO to indicate the world distribution of Cassytha.
According to the species list we collected, Fabaceae is the most preferred host family parasitized by Cassytha based on species number, followed by family Myrtaceae and Asteraceae (Table 2). There are 32 reported host species in the Fabaceae family from 7 countries and regions, 19 host species for Myrtaceae, and 16 host species for Asteraceae. In this respect, Acacia (six host species in this genus) and Eucalyptus (five species in this genus) are the most preferred host genus parasitized by Cassytha (Supplementary Appendix Table S2). For example, Acacia auriculiformis, Acacia confusa, and Acacia sieberiana are recognized to be host species of C. filiformis in Benin (Quetin-Leclercq et al., 2004), China (Gong, 1986), and Tanzania (Buriyo et al., 2015), respectively. The Key to Tasmanian Vascular Plants (2019) states that C. melantha is distributed across a range of woody species and Acacia spp., such as Acacia melanoxylon is recognized as its preferred host plant (Ziegler, 1995; Dueholm et al., 2017). Acacia myrtifolia and Acacia paradoxa are also preferred host species for C. pubescens (Cirocco et al., 2017; Facelli et al., 2020). Eucalyptus tetrodonta is the host species for C. filiformis in Australia (Ziegler, 1995), and Eucalyptus citriodora, Eucalyptus exserta, Eucalyptus robusta, and Eucalyptus rudis are host species for C. filiformis in China (Gong, 1986; Li et al., 1992). C. melantha has caused severe damage to Eucalyptus spp. in Australia (Pederick and Zimmer, 1961) and Eucalypts are found to be specific hosts for C. melantha in Western Australia (Archer, 2012). Additionally, C. filiformis also infect crop species such as cashew (Anacardium occidentale), orange (Citrus sinensis), lemon (Citrus limon), mango (Mangifera indica), cloves (Eugenia aromatica), nutmeg (Myristica fragrans), and avocado (Persea americana) (Nelson, 2008; Buriyo et al., 2015).
Twenty-eight host species of C. filiformis from India (Nayar and Nayar, 1952) and 81 host species from the Bahamas (Werth et al., 1979) are mentioned but have not been accompanied by a detailed list. C. filiformis was also reported to be found in Brazil (Giannerini et al., 2015), Nigeria (Ambi et al., 2017), Puerto Rico (Levins and Heatwole, 1973), Polynesia, Sri Lanka, Bangladesh (Ara et al., 2007), Brunei Darussalam (Tennakoon et al., 2016), Vietnam, Malaysia, Philippines, Indonesia, and Fiji (Nugraha et al., 2020), but information regarding host species is lacking. C. glabella is found to be a climber of many plant communities in Gibraltar Range and part of Washpool National Parks in New South Wales, Australia, including Eucalyptus olida–Eucalyptus ligustrina–Eucalyptus cameronii forest and woodland, Baeckea omissa–Epacris obtusifolia–Leptospermum arachnoides bogs, and Callicoma serratifolia–Eucalyptus oreades open forest and shrubland (Hunter and Sheringham, 2008). C. ciliolata is known as a common parasite in the Cape region of South Africa with a number of hosts (Schroeder, 1967). Unfortunately, this study has not reported the specific host plants parasitized by C. ciliolata. Cassytha pedicellosa, endemic to Tasmania, is distributed in heathland habitat and its associated species include Lepidosperma concavum, Leptospermum scoparium, Hibbertia procumbens, Banksia marginata, Dillwynia glaberrima, Amperea xiphoclada, Epacris impressa, Monotoca scoparia, Monotoca glauca, Allocasuarina monilifera, and Selaginella uliginosa (Wapstra et al., 2009). However, it is not explicitly stated if these are true host species (establishing successful haustorial connections) of C. pedicellosa. More host species for Cassytha should be identified in the Cassytha distribution habitats, so as to confirm their host preference.
Despite the availability of a wide range of host species, the level of infection by Cassytha varies among those hosts. For example, 30 host species in forests of the Jhargram district of West Bengal had 30–98% infection percentage/frequency parasitized by C. filiformis (Debabrata, 2018). Werth et al. (1979) have stated that the 81 host species from the Bahamas were not equally infected. Additionally, it has been reported that C. filiformis has a broader host range than Cuscuta in Brunei Darussalam, but the host preference (true haustorial initiation) is much narrower (Tennakoon et al., 2016). In line with our expectation, Cassytha tended to parasitize woody host species and species from certain families. Cassytha may have a variable preference for host species, perhaps due to the availability of more suitable host-derived resources in those plants. Different host species may also have different susceptibility, i.e., resistance levels to Cassytha parasitism. It is not known what factors might contribute to the susceptibility of various hosts. One study direction may be to investigate host stem exogenous histology and Cassytha haustorial penetration behavior. Host plants with soft thin barks and periderm seem to be more preferred by C. filiformis than species having hard-thick or suberized-scaly barks (Buriyo et al., 2015). Another study direction would be to compare the growth habits of host species, such as height and branch quantity. For example, C. filiformis seems to prefer low and much-branched woody host plants (Werth et al., 1979).
Influence of Cassytha Parasite on Host Growth and Development
In this section, we discuss the impact of Cassytha parasitism on ecological, physiological, and molecular aspects of host species.
Effect on Growth and Photosynthesis
Cassytha usually absorbs xylem-derived nutrients and water from host plants, decreasing their growth, reproduction, and biomass (Figure 3) and can even lead to the death of some hosts under heavy infestation (Burch, 1997; Prider et al., 2011). It has been reported that C. pubescens reduced the flowering of the legume host C. scoparius by 50% and consequently impacted fruit and seed production (Prider et al., 2011). Additionally, the noxious alien invasive weed U. europaeus when parasitized by native C. pubescens in South Australia, had a significantly lower shoot, root, and total biomass. The total biomass of infected hosts was 65–88% lower than the of uninfected plants (Cirocco et al., 2020). The adverse impact of the parasite on small invasive shrub host plants U. europaeus was more severe than on larger plants within the same species. On the other hand, the biomass of the parasite was lower when it was parasitizing smaller host plants, but was similar on a per gram of host total biomass basis in C. pubescens (Cirocco et al., 2020). This pattern may be expected at the cross-species level because Cirocco et al. (2021a) suggested that the native host was strongly affected by C. pubescens due to its smaller size. We do not know if this is true for other Cassytha–host associations involving different Cassytha species and/or other host species.
Biomass decrease of host species is partially attributed to decreased photosynthesis by Cassytha parasitism. It has been found that the photosynthetic rates, stomatal conductance, transpiration rate, light-saturated electron transport rates, pre-dawn (Fv/Fm), and midday (ΦPSII) quantum yields of the C. pubescens parasitized host C. scoparius were significantly lower than uninfected plants (Shen et al., 2010). It has also been reported that Cassytha infection significantly decreases midday PSII efficiency and the maximum electron transport rates of the alien invasive shrub host U. europaeus, regardless of different environmental variations across several field sites in South Australia (Cirocco et al., 2018). The results may be correlated to the decreased N and K levels in infected plants due to Cassytha infestation and increased Fe and Al content due to rhizosphere acidification induced by parasitism. This inevitably leads to suppressed photosynthesis and ultimately to chronic photoinhibition (Cirocco et al., 2018).
Water and Nutrient Transmissions
Water and nutrient transmissions are key issues leading to an understanding of parasite–host interactions (Bell and Adams, 2011). The essential feature of water movement from soil to plants, and from host species to parasites, is a gradient of decreasing water potential (Ehleringer and Marshall, 1995). The water potential is usually more negative and stomatal conductance and transpiration rate are greater for aerial hemiparasites than for host species (Ehleringer and Marshall, 1995). However, water relations of Cassytha–host associations are generally sparse and have produced inconsistent results. Cassytha parasitism has been found to have no effect (Cirocco et al., 2016a, 2020) or negative effects (Cirocco et al., 2021b) on the water potential of the alien host U. europaeus. It has been further reported that the parasite’s water potential was significantly lower than the host, and the parasite had significantly lower water potential under low water than high water conditions (Cirocco et al., 2021b). Additionally, stomatal conductance and transpiration rates of the host C. scoparius infected by C. pubescens were significantly decreased when compared with uninfected plants (Shen et al., 2010).
Some angiosperm parasites can become a sink for host-produced photosynthates (Watling and Press, 2001). The facultative root parasite Rhinanthus minor, the obligate root hemiparasites Striga spp. and the root holoparasite Orobanche spp. obtain approximately 10, 30, and 100% of the carbon requirements from their hosts, respectively (Irving and Cameron, 2009). Stem hemiparasitic mistletoes have obtained around 40–80% heterotrophic carbon from hosts and it has been reported that the proportion of heterotrophic carbon gained by mistletoes depends on different host species and life history stages (Těšitel et al., 2010). The proportion of heterotrophic carbon obtained by Cassytha species, which subsists on xylem-derived solutes such as amino acids, sugars, and organic acids is not known. This is an area that requires further studies to understand the physiological implications of Cassytha infestation on different hosts.
Parasite resource removal from the host may be the primary mechanism for decreases in host biomass (Cirocco et al., 2021a). Cassytha primarily absorbs nutrients via xylem-xylem contact with the host species (Li and Yao, 1992). Studies conducted in South Australia have shown that N and K contents in infected plants of the alien invasive leguminous host U. europaeus by the parasite C. pubescens decreased by 17.6 and 22.4% compared with the uninfected plants, but the Al and Fe contents increased 140.5 and 40.5% due to rhizosphere acidification induced by parasitism (Cirocco et al., 2018). Additionally, the N, P, and K concentration of the parasite C. pubescens is higher when infecting small host plants of U. europaeus than in large ones (Cirocco et al., 2020).
Metabolites and Molecules Translocation
Metabolites usually change and translocate between parasites and host plants prior to and after parasitism, usually from the former to the latter (Birschwilks et al., 2007). The energy charge calculated from ATP (adenosine triphosphate), ADP (adenosine diphosphate), and AMP (adenosine monophosphate) of C. filiformis seedlings were low prior to parasitism and greatly increased after parasitizing Ipomoea pes-caprae due to effective energy production, thus resulting in further elongation and development of Cassytha seedlings (Furuhashi et al., 2016). However, the energy charge of the host species I. pes-caprae did not change due to parasitism, which indicates that I. pes-caprae was relatively tolerant to water and metabolites loss to Cassytha. The profiled steroid pattern was not affected by parasitism in both C. filiformis and I. pes-caprae, but the absolute abundance of these steroids tended to decrease after parasitism. Similarly, the absolute abundance of most polar metabolites (such as fructose, glucose, sucrose, and galactitol) of C. filiformis decreased after parasitism thus attributing to water absorption from the host and the lignification process that induces fresh weight increase. However, for the host I. pes-caprae, the absolute amount of fructose, glucose, and sucrose decreased, whilst that of galactitol increased and pinitol, quinate, and organic acids did not change after Cassytha parasitism (Furuhashi et al., 2016). These results indicate that parasitism did not cause severe pathogenic responses in I. pes-caprae, although the growth and reproductive traits were negatively affected. It is not known if these patterns can be generalized for other Cassytha–host associations. Further studies are called for in this discipline. Cassytha can also absorb and accumulate secondary metabolites from host species. For example, gelsemium alkaloids were detected in C. filiformis when it was grown in association with the poisonous “heartbreak grass” Gelsemium elegans and absorbed the gelsemium toxins present in cell sap (Cheung et al., 2018). This implies the importance of assessing the hosts parasitized by Cassytha when they are harvested for medicinal preparations.
Parasite plants acquire various macromolecules such as mRNA, viruses, protein, and phytoplasmas from their hosts (LeBlanc et al., 2012). Some reports are available for parasites such as Cuscuta, Cytinus, Convolvulaceae, and Santalales (LeBlanc et al., 2012) showing them acquiring macromolecules from their hosts. For example, Cuscuta has the ability to transmit viruses and phytoplasmas between different hosts as a vector, being commonly called a Cuscuta “bridge” (Marcone et al., 1997; Birschwilks et al., 2006). Horizontal gene transfer is another example of parasite–host macromolecule exchange. We do not know if Cassytha can acquire viruses or other macromolecules from their hosts, but some evidence is available to demonstrate Cassytha–host associations involving horizontal gene transfer (Davis and Xi, 2015).
Comparison of Cassytha Infection on Different Hosts
Host plants may show different resistance/tolerance levels to Cassytha parasitism. In a study conducted by Facelli et al. (2020), the native Cassytha is shown to have greater impacts on their exotic hosts than the native host plants in South Australia. There have been several case studies done in Australia under both field and glasshouse conditions to assess the impacts of Cassytha in this regard. For example, C. pubescens infection had a significant negative effect on the transpiration rates and biomass production of the alien invasive shrub host C. scoparius compared with that of native shrub host Leptospermum myrsinoides. Intense C. pubescens infection can even induce death for the host C. scoparius (Prider et al., 2009). C. pubescens parasitism has also been shown to significantly decrease the total biomass of the alien invasive host U. europaeus, but not the native host A. paradoxa (Cirocco et al., 2017). In addition, C. pubescens had higher photosynthetic rates, growth rates and biomass when parasitizing introduced hosts than the native hosts (Prider et al., 2009). These variations may be attributed to the greater level of resources (as higher nutrient contents) that the introduced host can provide and/or the greater resistance made by the native hosts against the successful Cassytha haustorial establishment. In order to understand the level of resistance exhibited by different hosts, Facelli et al. (2020) investigated the flow of nutrients between hosts and parasites. It was found that the connections of the haustorium with the vascular system of the native host A. myrtifolia were not successfully developed despite being morphologically alike to those formed on the alien invasive hosts C. scoparius and U. europaeus. They further demonstrated that radiolabeled phosphorus (32P) was not transferred from the native host A. myrtifolia to the parasite C. pubescens due to the incompatibility of haustorial connections. No definitive studies are available on the resistance exhibited by different hosts toward Cassytha infection.
Impacts of Environmental Change on Cassytha–Host Interactions
In this section, we aim to test whether global environmental changes favor the hemiparasitic Cassytha or their hosts, specifically under elevated temperature and CO2 concentrations, and fluctuating water and soil nutrient conditions.
Impacts of Temperature and Elevated CO2 Levels on Cassytha–Host Interactions
Both biotic factors and abiotic factors can alter parasite performance and its impact on host species, leading to compounded parasite–host behavior. Temperature is the prevailing environmental factor that influences plant growth, and also affects angiosperm parasite–host interactions. A case study involving the interaction between the hemiparasite Castilleja sulphurea and its host Bouteloua gracilis under circumstances of changing environment has found that a 3°C temperature increase in summer exacerbated the adverse effects on host species due to the production of more haustoria and aboveground biomass of the hemiparasite (Rafferty et al., 2019). Bell et al. (2020) have also found increased effects of the dwarf mistletoe (Arceuthobium tsugense) on hemlock Tsuga heterophylla under warmer and drier conditions. Similarly, the proportion of mistletoe Viscum album infection on Pinus nigra and Pinus sylvestris declined with the elevational increase (viz. temperature decrease) (Zamora and Mellado, 2019). The frequency of C. pubescens in Mediterranean climate healthy woodlands in South Australia has decreased from 1986 to 2010 due to a mean temperature increase of 4°C in those habitats (Guerin and Lowe, 2013). However, the exact impacts of temperature fluctuations on the overall dynamics of Cassytha–host associations are yet unknown.
Elevated CO2 alleviated the effects of the root holoparasite Orobanche minor on host species Trifolium repens by stimulating the host growth (Dale and Press, 1998). Similar results were found in the facultative hemiparasite R. minor and its host Poa pratensis, the root hemiparasitic angiosperm Striga hermonthica, and its host Oryza sativa and the aerial hemiparasitic plant Dendrophthoe curvata and its host species Andira inermis, M. indica, and Vitex pinnata under elevated CO2 (Watling and Press, 2000; Hwangbo et al., 2003; Le et al., 2016). No studies are reported about the influence of elevated CO2 on Cassytha–host associations.
Impacts of Water Availability on Cassytha–Host Interactions
Water availability is another important environmental factor that influences angiosperm parasite–host plant interactions. Drought has been shown to decrease hosts’ growth rate and resource availability, thus indirectly influencing parasites (Zagorchev et al., 2021). It has been shown that the success of mistletoe establishment is related to host water status and the proportion of mistletoe infection decreased with the increase of water stress experienced by hosts (Miller et al., 2003). Cirocco et al. (2016a) have found that high water availability increased the negative effects of C. pubescens when parasitizing U. europaeus, with significantly lower host total biomass and parasite grew better at high water availability than in low water availability conditions. The predawn PSII efficiency of U. europaeus parasitized by C. pubescens was relatively low in wettest sites than in drier habitats (Cirocco et al., 2018). The physiological basis of this result is that the parasite C. pubescens had higher water potential, stomatal conductance, and growth rate at high water availability, leading to a higher demand of host-derived resources from the host U. europaeus, thus making it perform rather poorly.
Light Effects on Cassytha–Host Interactions
The angiosperm aerial parasites decrease the photosynthesis of host species (Bell and Adams, 2011) and affect host PSII efficiency and the use of available light (Cameron et al., 2008). It has been shown that the parasite C. pubescens significantly decreased the foliar pigment concentration of the host species L. myrsinoides under both high and low photosynthetically active radiation (PAR) levels (Cirocco et al., 2015). However, infected L. myrsinoides plants have also maintained a similar photoprotective capacity similar to those uninfected plants irrespective of exposure to different light levels, thus preventing photodamage and demonstrating tolerance to Cassytha parasitism. Additionally, it has been found that a larger parasite growing on a larger host in high light had the same negative effect on host growth as a smaller parasite growing on a smaller host in low light (Cirocco et al., 2016b). Further in-depth studies are required to exactly understand the influence of different light conditions (e.g., light intensity and quality) on the overall behavior of Cassytha–host associations.
Effects of Nitrogen and Phosphorus Availability on Cassytha-Host Interactions
Global atmospheric nitrogen (N) deposition is increasing due to human activities (Kanakidou et al., 2016), and these N effects on angiosperm parasite–host associations have long been recognized. A high external N supply has been found to reduce the effects of holoparasite on host growth due to less influence of infection on root biomass of the hosts (Shen et al., 2013) and negative effect on the early growth of parasite (Cechin and Press, 1993) compared with low external N supply. However, such influences were found to be different in Cassytha–native legume host and Cassytha–introduced legume host associations (Cirocco et al., 2017). High external N supply reduced the negative effects of C. pubescens infection on root biomass of the native legume species A. paradoxa, but it significantly increased the negative effects of C. pubescens infection on root biomass of the introduced legume species U. europaeus, compared with low external N supply. This is attributed to the reduction of nodule biomass of infected U. europaeus at a high external N supply when compared with the native host A. paradoxa. When native and introduced legume hosts are not parasitized by C. pubescens, external N supply had insignificant effects on their biomass (Cirocco et al., 2017). These results together with higher foliar N concentration present in native A. paradoxa than the exotic host U. europaeus indicate that the native host has adapted well by fixing more N to supply both its own and Cassytha growth. Other physiological aspects such as the photosynthetic efficiency of Cassytha infected hosts under N supplements, especially of that non-nitrogen fixing hosts are yet unknown. Cirocco et al. (2021b) further investigated the combined effects of water and nitrogen availability on C. pubescens–U. europaeus association, but did not find additive or antagonistic effects of water and nitrogen on parasite–host interaction. However, it was found that C. pubescens can absorb more nitrogen from the host U. europaeus at high water availability conditions.
Phosphorus is another essential nutrient that limits plant growth, which can influence angiosperm parasite–host associations (Davies and Graves, 2000). Cirocco et al. (2021a) conducted an experiment to assess the effects of external P supply on C. pubescens and a native legume A. paradoxa association. They found that external high P supply did not significantly influence the biomass of both the parasite C. pubescens and the host A. paradoxa compared with low P supply. However, the host A. paradoxa had significant lower foliar N and P concentration under low external P supply than high P supplements, resulting in lower stem phosphorus of C. pubescens in low external P supply than in high P supply treatment. The authors concluded that soil P conditions may have little or no impact on the overall performance of Cassytha–host associations in nature (Cirocco et al., 2021a). However, it may evidence three possibilities; first, different host species have divergent P sensitivities. Thus, the result may depend on different parasite–host combinations. Second, a 3-month experimental period is not long enough to detect P impact on the parasite–host association. Third, the effect of P supply on parasite-host association could be co-limited by N, because N and P are proportionally acquired by plants (Maistry et al., 2015). This topic clearly needs further study.
The major components of global environmental change are increasing CO2 concentrations, increasing temperatures, increasing N, and increasing or decreasing precipitation (Liu et al., 2017). Based on this literature review, the negative effects of the aerial hemiparasite Cassytha on their hosts increased under increasing water availability and N supply scenarios (Figure 3). Most previous studies on Cassytha–host associations have investigated the impacts of only one environmental factor. The intricacies involved with the cumulative impact of two or more of these factors on Cassytha–host associations have been investigated recently (e.g., Cirocco et al., 2021b) but still call for more research. A coordinated series of long-term studies are required to predict the performance of Cassytha–host associations under scenarios of climate change. It remains to be ascertained whether the presently documented evidence of the influence of factors such as temperature, water, sunlight, and nutrient (e.g., N and P) availability on Cassytha–host associations becomes more intense or mild until such complex studies are undertaken to assess the combined effects.
Benefits and Harms of Cassytha–Host Interactions
In this section, we address the question of whether Cassytha–host interactions are generally beneficial or harmful for natural ecosystems and humans.
Damage and Control of Cassytha as a Parasitic or Invasive Weed
Weeds act as significant biological constraints that can affect crop productivity (MacLaren et al., 2020). In this respect, the aerial hemiparasitic Cassytha species are a type of weed species (Musselman, 1996). For example, in tropical regions, parasite C. filiformis affect important economic crops such as Acacia, Azadirachta, Mangifera, Myrtaceae, and Theaceae (Li et al., 1991; Mythili et al., 2011). It has been found that 20% of cashew trees and 16% of orange trees were affected by C. filiformis in Tanzania, where 30–40% of total crop production is lost due to crop pests and diseases (Buriyo et al., 2015). The incidence of attacks on the forestry industry of southeastern China due to C. filiformis infestation exceeded 15%, reaching 50–60% in young Camellia oleosa forest in Guangxi Province (Gong, 1986).
Numerous parasitic plants including those of Cassytha have dramatic impacts on plant communities despite being less than 5% of the community biomass proportion, affecting community biomass, community diversity, vegetation cycling, and zonation aspects (Press and Phoenix, 2005). It has been found that C. filiformis invasion decreased the evenness and biomass but increased the density and species richness of aboveground plant communities in the forest of the Paracel Islands in the northern South China Sea (Cai et al., 2020). It also changed soil fauna and microbial community structure (Cai et al., 2020). Cassytha invasion may have both positive and negative influences on natural ecosystems and they might be keystone species. For example, C. ciliolata does well where there is a diverse range of hosts, e.g., in the Cunonia community of Cape floristic community (Meek et al., 2013).
Parasitic weed control is important for the protection of infected crops. In lightly infected regions, C. filiformis can be manually removed by hand-pulling, this being very efficient, especially at the seedling stage or when young stems are in the initial twining stages before producing flowers and fruits. In extensively infected regions, the application of suitable concentrations of selective herbicides such as Bentazon can be used to remove C. filiformis (Li et al., 1991). On the whole, invasive properties of Cassytha and their impacts on agricultural and natural communities and ecosystems need further study.
Use of Cassytha as a Biocontrol Agent
Allelopathy is a biological phenomenon by which one plant can release chemicals that influence the survival and growth of plants in the same vicinity (Zhang et al., 2021). C. filiformis was shown to have negative allelopathic effects on three indicator plants O. sativa, Echinochloa crus-galli (Barnyardgrass), and Vigna radiata. Specific allelopathic effects of Cassytha on these plants were confirmed by applying Cassytha extracts in powder and water forms for bioassays, plant house studies, and field experiments (Thang et al., 2021). The dry weight of barnyardgrass was suppressed by 76.7 and 42.7% when C. filiformis extracts were applied in powder form in both net house and field trials. This study provided useful evidence about the potential of using C. filiformis as a natural herbicide to control weeds in non-paddy crop cultivation, but it is not known if other weed species can also be inhibited by C. filiformis.
Like Cuscuta, native Cassytha species can be used as a biocontrol agent to control plant invasion (Li et al., 2012; Těšitel et al., 2020). For example, the native parasite C. filiformis in Florida was recognized as a component of an integrated approach to managing the introduced and invasive tree Schinus terebinthifolius. C. filiformis combined with the leaflet rolling moth Episimus unguiculus herbivory greatly decreased the performance of S. terebinthifolius for at least 2 months after the removal of the moths (Manrique et al., 2009). The native hemiparasite C. pubescens in Australia also implied serious effects on the growth and biomass of the introduced legume species U. europaeus and C. scoparius, but not the native legume A. paradoxa and L. myrsinoides (Myrtaceae), under both glass house and field conditions (Prider et al., 2009; Cirocco et al., 2017). C. pubescens parasitism and seed predator Bruchidius villosus (Bruchidae) are found to have a sub-additive effect on the invasive species C. scoparius, which can be used as a good combination of biocontrol agents (Prider et al., 2011).
Conclusion and Future Directions
Cassytha clearly demonstrates parasitism-related habits, such as flowering all year round for many species in this genus, autotrophy, stem twining, and producing haustoria. However, coordinated studies are required to precisely understand its seed biology and the duration of autotropism in order that its impact on the functioning of associated hosts in both agricultural and natural settings can be assessed. Cassytha tends to parasitize woody plants and species from certain families such as Fabaceae and Myrtaceae. However, it is not clear why Cassytha has varying levels of infection on different hosts. It may be due to factors such as less resources that a particular host provides, relatively high level of natural host resistance to parasitism, and incompatible host size or the anatomy of the host bark that can resist successful haustorial establishments. Cassytha absorbs water, N, P, and K nutrients, and possibly metabolites and macromolecules from host plants via haustoria to promote growth and increase its own biomass. While the growth, photosynthesis, reproduction, and biomass of some host plants were dramatically decreased. We are still far from understanding the underlying physiological and molecular level mechanisms of the extensive Cassytha–host interactions. For example, what roles do microorganisms play in parasitism of the Cassytha–host associations? Global environmental changes may increase the severity of Cassytha parasitism on host plants from increasing water and N availability perspectives. More studies are needed to ascertain the effects of multiple environmental factors on Cassytha–host associations, such as global warming, drought and N interactions and the influences of biological factors such as pollinators, predators, and microbes. Cassytha itself can be a harmful weed under heavy infestations, whilst it could be a biocontrol agent that can be used to reduce the spread of exotic weeds/invasive plants, and also a keystone species in natural ecosystems. Long-term community and ecosystem level field studies on Cassytha–host associations clearly need to be explored in a coordinated manner. Results of such studies would further improve our understanding of this aerial hemiparasite and will enable us to predict the trends of future spread of Cassytha under environmental change scenarios.
Author Contributions
KT and SF developed the project. HZ and KT wrote the manuscript. SF reviewed the manuscript by rewriting, discussing, and commenting. All authors contributed to the manuscript and approved the submitted version.
Funding
This work was partly supported by the Biodiversity, People, and the Changing Environment (BioPeCE) multidisciplinary research group funding allocated through the School of Science, Psychology and Sport of Federation University Australia and HZ was supported by the Strategic Priority Research Program of the Chinese Academy of Sciences (XDA23080401) and the National Natural Science Foundation of China (41971069).
Conflict of Interest
The authors declare that the research was conducted in the absence of any commercial or financial relationships that could be construed as a potential conflict of interest.
Publisher’s Note
All claims expressed in this article are solely those of the authors and do not necessarily represent those of their affiliated organizations, or those of the publisher, the editors and the reviewers. Any product that may be evaluated in this article, or claim that may be made by its manufacturer, is not guaranteed or endorsed by the publisher.
Acknowledgments
We are grateful to Michael Mcbain and Zhaogang Liu for extending their expertise to develop Cassytha global distribution map (Figure 2).
Supplementary Material
The Supplementary Material for this article can be found online at: https://www.frontiersin.org/articles/10.3389/fpls.2022.864110/full#supplementary-material
Footnotes
- ^ http://www.theplantlist.org/
- ^ https://www.gbif.org/zh/
- ^ https://profiles.ala.org.au/opus/foa
- ^ https://biodiversity.org.au/nsl/services/search/taxonomy
References
Ambi, A. A., Nuru, G. F., Mora, A. T., and Ahmad, A. (2017). Pharmacognostic studies and elemental analysis of Cassytha filiformis Linn. J. Pharmacogn. Phytother. 9, 131–137. doi: 10.5897/JPP2017.0448
Ara, H., Mia, M. M. K., and Khan, B. (2007). An annotated checklist of Lauraceae in Bangladesh. Bangladesh J. Plant Taxon. 14, 147–162. doi: 10.3329/bjpt.v14i2.533
Archer, W. (2012). Cassytha melantha-Large Dodder Laurel in Esperance Wildflowers-Flora Observations Within 160 km (100 miles) of Esperance, Western Australia. Available online at: http://esperancewildflowers.blogspot.com/search/label/Lauraceae%20-%20Cassytha (accessed April 18, 2022).
Awang, K., Conran, J. G., and Waycott, M. (2018). Cuticular and Ultrastructure Characters on Cassytha L. (Lauraceae) Stem. Available online at: https://www.researchgate.net/publication/325870821_Cuticular_and_Ultrastructure_Characters_on_Cassytha_L_Lauraceae_Stem/citations (accessed April 18, 2022).
Balasubramanian, D., Lingakumar, K., and Arunachalam, A. (2014). Characterization of anatomical and physiological adaptations in Casssytha filiformis L.-an advanced obligate hemiparasite on Morinda tinctoria Roxb. Taiwania 59, 98–105. doi: 10.6165/tai.2014.59.98
Bardgett, R. D., Smith, R. S., Shiel, R. S., Peacock, S., Simkin, J. M., Quirk, H., et al. (2006). Parasitic plants indirectly regulate below-ground properties in grassland ecosystems. Nature 439, 969–972. doi: 10.1038/nature04197
Beaman, B. G. (1971). Comparative Systematic Anatomy of the Parasite Cassytha (Lauraceae). Ph.D. thesis. College Park, MD: University of Maryland.
Bell, D. M., Pabst, R. J., and Shaw, D. C. (2020). Tree growth declines and mortality were associated with a parasitic plant during warm and dry climatic conditions in a temperate coniferous forest ecosystem. Glob. Change Biol. 26, 1714–1724. doi: 10.1111/gcb.14834
Bell, T. L., and Adams, M. A. (2011). Attack on all fronts: functional relationships between aerial and root parasitic plants and their woody hosts and consequences for ecosystems. Tree Physiol. 31, 3–15. doi: 10.1093/treephys/tpq108
Birschwilks, M., Haupt, S., Hofifius, D., and Neumann, S. (2006). Transfer of phloem-mobile substances from the host plants to the holoparasite Cuscuta sp. J. Exp. Bot. 57, 911–921. doi: 10.1093/jxb/erj076
Birschwilks, M., Sauer, N., Scheel, D., and Neumann, S. (2007). Arabidopsis thaliana is a susceptible host plant for the holoparasite Cuscuta spec. Planta 226, 1231–1241. doi: 10.1007/s00425-007-0571-6
Brophy, J. J., Goldsack, R. J., and Forster, P. I. (2009). The essential oils of some Australian Cassytha species (Lauraceae). J. Essent. Oil Res. 21, 543–546. doi: 10.1080/10412905.2009.9700239
Burch, J. N. (1997). Interaction of the Parasitic Angiosperm Cassytha filiformis L. (Lauraceae) with the Exotic Schinus terebinthifolius Raddi (Anacardiaceae) in Southern Florida. PhD Dissertation. Miami, FL: Florida International University.
Buriyo, A. S., Kasuga, L., Moshi, H. N., and Nene, W. A. (2015). Ecological distribution and abundance of the parasitic weed, Cassytha filiformis L. (Lauraceae) in major cashew, Anacardium occidentale L. growing regions in Tanzania. Int. J. Basic Appl. Sci. 5, 109–116.
Cai, H., Lu, H., Tian, Y., Liu, Z., Huang, Y., and Jian, S. (2020). Effects of invasive plants on the health of forest ecosystems on small tropical coral islands. Ecol. Indic. 117:106656. doi: 10.1016/j.ecolind.2020.106656
Cameron, D. D., Geniez, J. M., Seel, W. E., and Irving, L. J. (2008). Suppression of host photosynthesis by the parasitic plant Rhinanthus minor. Ann. Bot. 101, 573–578. doi: 10.1093/aob/mcm324
Caraballo-Ortiz, M. A. (2019). Parasitic plants: important components of biodiversity. Plant Press 22. Available online at: https://nmnh.typepad.com/the_plant_press/2019/10/parasitic-plants-important-components-of-biodiversity.html (accessed April 18, 2022).
Carnegie, A. J., Bi, H., Arnold, S., Li, Y., and Binns, D. (2009). Distribution, host preference, and impact of parasitic mistletoes (Loranthaceae) in young eucalypt plantations in New South wales, Australia. Botany 87, 49–63. doi: 10.1139/B08-127
Cechin, I., and Press, M. C. (1993). Nitrogen relationship of the sorghum-Striga hermonthica host-parasite association: growth and photosynthesis. Plant Cell Environ. 16, 237–247. doi: 10.1111/j.1365-3040.1993.tb00866.x
Cheung, W. L., Law, C. Y., Lee, H. C. H., Tang, C. O., Lam, Y. H., Ng, S. W., et al. (2018). Gelsemium poisoning mediated by the non-toxic plant Cassytha filiformis parasitizing Gelsemium elegans. Toxicon 154, 42–49. doi: 10.1016/j.toxicon.2018.09.009
Cirocco, R. M., Facelli, E., Delean, S., and Facelli, J. M. (2021a). Does phosphorus influence performance of a native hemiparasite and its impact on a native legume? Physiol. Plant. 173, 1889–1900. doi: 10.1111/ppl.13530
Cirocco, R. M., Watling, J. R., and Facelli, J. M. (2021b). The combined effects of water and nitrogen on the relationship between a native hemiparasite and its invasive host. New Phytol. 229, 1728–1739. doi: 10.1111/nph.16944
Cirocco, R. M., Facelli, J. M., and Watling, J. R. (2016a). High water availability increases the negative impact of a native hemiparasite on its non-native host. J. Exp. Bot. 67, 1567–1575. doi: 10.1093/jxb/erv548
Cirocco, R. M., Facelli, J. M., and Watling, J. R. (2016b). Does light influence the relationship between a native stem hemiparasite and a native introduced host? Ann. Bot. 117, 521–531. doi: 10.1093/aob/mcv193
Cirocco, R. M., Facelli, J. M., and Watling, J. R. (2017). Does nitrogen affect the interaction between a native hemiparasite and its native or introduced leguminous hosts? New Phytol. 213, 812–821. doi: 10.1111/nph.14181
Cirocco, R. M., Facelli, J. M., and Watling, J. R. (2018). A native parasitic plant affects the performance of an introduced host regardless of environmental variation across field sites. Funct. Plant Biol. 45, 1128–1137. doi: 10.1071/FP17358
Cirocco, R. M., Facelli, J. M., and Watling, J. R. (2020). The impact of a native hemiparasite on a major invasive shrub is affected by host size at time of infection. J. Exp. Bot. 71, 3725–3734. doi: 10.1093/jxb/eraa140
Cirocco, R. M., Waterman, M. J., Robinson, S. A., Facelli, J. M., and Watling, J. R. (2015). Native hemiparasite and light effects on photoprotection and photodamage in a native host. Funct. Plant Biol. 42, 1168–1178. doi: 10.1071/FP15132
Clarke, C. R., Timko, M. P., Yoder, J. I., Axtell, M. J., and Westwood, J. H. (2019). Molecular dialog between parasitic plants and their hosts. Annu. Rev. Phytopathol. 57, 279–299. doi: 10.1146/annurev-phyto-082718-100043
Close, D. C., Davidson, N. J., and Davies, N. W. (2006). Seasonal fluctuations in pigment chemistry of co-occuring plant hemi-parasites of distinct form and function. Environ. Exp. Bot. 58, 41–46. doi: 10.1016/j.envexpbot.2005.06.013
Collins, D. J., Culveor, C. C. J., Lamberton, J. A., Loder, J. W., and Price, J. R. (1990). Plants for Medicines. Melbourne, VIC: CSIRO.
Combes, C. (2001). Parasitism: The Ecology and Evolution of Intimate Interactions. Chicago, IL: The University of Chicago Press.
Dale, H., and Press, M. C. (1998). Elevated atmospheric CO2 influences the interaction between the parasitic angiosperm Orobanche minor and its host Trifolium repens. New Phytol. 140, 65–73. doi: 10.1046/j.1469-8137.1998.00247.x
Davies, D. M., and Graves, J. D. (2000). The impact of phosphorus on interactions of the hemiparasitic angiosperm Rhinanthus minor and its host Lolium perenne. Oecologia 124, 100–106. doi: 10.1007/s004420050029
Davis, C. C., and Xi, Z. (2015). Horizontal gene transfer in parasitic plants. Curr. Opin. Plant Biol. 26, 14–19. doi: 10.1016/j.pbi.2015.05.008
De La Harpe, A. C., Grobbelaar, N., and Visser, J. H. (1980). The ultrastructure of the chloroplast and the chlorophyll content of various South African parasitic flowering plants. Z. Pflanzenphysiol. Bd. 100, 85–90. doi: 10.1016/S0044-328X(80)80189-9
De La Harpe, A. C., Visser, J. H., and Grobbelaar, N. (1979). The chlorophyll concentration and photosynthetic activity of some parasitic flowering plants. Z. Pflanzenphysiol. Bd. 93, 83–87. doi: 10.1016/S0044-328X(79)80144-0
De La Harpe, A. C., Visser, J. H., and Grobbelaar, N. (1981). Photosynthetic characteristics of some South African parasitic flowering plants. Z. Pflanzenphysiol. Bd. 103, 265–275. doi: 10.1016/S0044-328X(81)80159-6
Debabrata, D. (2018). Cassytha filiformis in forests of Jhargram district of West Bengal. GSC Biol. Pharm. Sci. 4, 1–7. doi: 10.30574/gscbps.2018.4.1.0023
Dueholm, B., Bruce, D., Weinstein, P., Semple, S., Møller, B. L., and Weiner, J. (2017). Spatial analysis of root hemiparasitic shrubs and their hosts: a search for spatial signatures of above- and below-ground interactions. Plant Ecol. 218, 185–196. doi: 10.1007/s11258-016-0676-8
Ehleringer, J. R., and Marshall, J. D. (1995). “Water relations,” in Parasitic Flowering Plants, eds M. C. Press and J. D. Graves (London: Chapman and Hall), 125–140.
Facelli, E., Wynn, N., Tsand, H. T., Watling, J. R., and Facelli, J. M. (2020). Defence responses of native and invasive plants to the native generalist vine parasite Cassytha pubescens – anatomical and functional studies. Aust. J. Bot. 68, 300–309. doi: 10.1071/BT19136
French, K., and Westoby, M. (1996). Vertebrate-dispersed species in a fire-prone environment. Aust. J. Ecol. 21, 379–385. doi: 10.1111/j.1442-9993.1996.tb00624.x
Furuhashi, K., Iwase, K., and Furuhashi, T. (2021). Role of light and plant hormones in stem parasitic plant (Cuscuta and Cassytha) twining and haustoria induction. Photochem. Photobiol. 97, 1054–1062. doi: 10.1111/php.13441
Furuhashi, T., Furuhashi, K., and Weckwerth, W. (2011). The parasitic mechanism of the holostemparasitic plant Cuscuta. J. Plant Interact. 6, 207–219. doi: 10.1080/17429145.2010.541945
Furuhashi, T., Nakamura, T., and Iwase, K. (2016). Analysis of metabolites in stem parasitic plant interactions: interaction of Cuscuta-Momordica versus Cassytha-Ipomoea. Plants 5:43. doi: 10.3390/plants5040043
Giannerini, A. C., Quinet, A., and Andreata, R. H. P. (2015). Lauraceae no parque Nacional do Itatiaia, Brasil. Rodriguésia 66, 863–880. doi: 10.1590/2175-7860201566314
Glatzel, G., and Geils, B. W. (2009). Mistletoe ecophysiology: host–parasite interactions. Botany 87, 10–15. doi: 10.1139/B08-096
Gong, M. (1986). Preliminary study on biological characteristics and its harm of Cassytha filiformis. Trop. For. Technol. 2, 7–13.
Groom, P. K., and Lamont, B. B. (2015). “Parasitic plants in southwestern Australia,” in Plant Life of Southwestern Australia Adaptation of Survival, eds P. K. Groom and B. Lamont (Warsaw: DeGruyter Open). doi: 10.1007/s00442-004-1506-6
Guerin, G. R., and Lowe, A. J. (2013). Systematic monitoring of heathy woodlands in a Mediterranean climate-a practical assessment of methods. Environ. Monit. Assess. 185, 3959–3975. doi: 10.1007/s10661-012-2842-3
Heide-Jørgensen, H. S. (1991). Anatomy and ultrastructure of the haustorium of Cassytha pubescens R. BR. I. the adhesive disk. Bot. Gaz. 152, 321–334.
Huang, C., Zhang, L., Dong, L., Zhang, X., Han, L., and Zhang, C. (2021). Research progress of Cassytha filiformis L. J. Hainan Med. Univ. doi: 10.13210/j.cnki.jhmu.20210303.003 [Epub ahead of print].
Hunter, J. H., and Sheringham, P. (2008). Vegetation and floristic diversity in Gibraltar Range and part of Washpool National Parks, New South Wales. Cunninghamia 10, 439–474.
Hwangbo, J.-K., Seel, W. E., and Woodin, S. J. (2003). Short-term exposure to elevated atmospheric CO2 benefits the growth of a facultative annual root hemiparasite, Rhinanthus minor (L.), more than that of its host, Poa pratensis (L.). J. Exp. Bot. 54, 1951–1955. doi: 10.1093/jxb/erg194
Irving, L. J., and Cameron, D. D. (2009). You are what you eat: interactions between root parasitic plants and their hosts. Adv. Bot. Rev. 50, 87–138. doi: 10.1016/S0065-2296(08)00803-3
Jones, C. G., Lawton, J. H., and Shachak, M. (1994). Organisms as ecosystem engineers. Oikos 69, 373–386. doi: 10.1353/pbm.2003.0003
Kanakidou, M., Myriokefalitakis, S., Daskalakis, N., and Fanourgakis, G. (2016). Past, present, and future atmospheric nitrogen deposition. J. Atmos. Sci. 73, 2039–2047. doi: 10.1175/JAS-D-15-0278.1
Key to Tasmanian Vascular Plants (2019). Cassytha melantha (Lauraceae) 3: 597. Hobart, TAS: University of Tasmania.
Kokubugata, G., Nakamura, K., Forster, P. I., Wilson, G. W., Holland, A. E., Hirayama, Y., et al. (2012). Cassytha pubescens and C. glabella (Lauraceae) are not disjunctly distributed between Australia and the Ryukyu Archipelago of Japan – evidence from morphological and molecular data. Aust. Syst. Bot. 25, 364–373. doi: 10.1071/SB10040
Kokubugata, G., and Yokota, M. (2012). Host specificity of Cassytha filiformis and C. pergracilis (Lauraceae) in the Ryukyu Archipelago. Bull. Natl. Mus. Nat. Sci. Ser. B 38, 47–53.
Krasylenko, Y., Těšitel, J., Ceccantini, G., Oliveira-da-Silva, M., Dvorák, V., Steele, D., et al. (2021). Parasites on parasites: hyper–, epi–, and autoparasitism among flowering plants. Am. J. Bot. 108, 8–21. doi: 10.1002/ajb2.1590
Kuijt, J. (1969). The Biology of Parasitic Flowering Plants. Berkeley, CA: University of California Press.
Lanini, W. T., and Kogan, M. (2005). Biology and management of Cuscuta in crops. Cienc. Invest. Agric. 32, 127–141.
Le, Q. V., Tennakoon, K. U., Metali, F., Lim, L. B. L., and Bolin, J. F. (2016). Ecophysiological responses of mistletoe Dendrophthoe curvata (Loranthaceae) to varying environmental parameters. J. Trop. For. Sci. 28, 59–67.
LeBlanc, M., Kim, G., and Westwood, J. H. (2012). RNA trafficking in parasitic plant systems. Front. Plant Sci. 3:203. doi: 10.3389/fpls.2012.00203
Levins, R., and Heatwole, H. (1973). Biogeography of the Puerto Rican bank: introduction of species onto Palominitos Island. Ecology 54, 1056–1064. doi: 10.2307/1935571
Li, J., Jin, Z., and Song, W. (2012). Do native parasitic plants cause more damage to exotic invasive hosts than native non-invasive hosts? An implication for biocontrol. PLoS One 7:e34577. doi: 10.1371/journal.pone.0034577
Li, Q., Yao, D., Cai, J., and Huang, L. (1992). Host range investigation of Cassytha filiformis. Guangxi Plant Prot. 4, 21–24.
Li, Y., and Yao, D. (1992). Anatomical and histochemical studies of haustrial development of Cassytha filiformis L. Acta Bot. Sin. 34, 753–757.
Li, Y., Yao, D., and Huang, J. (1991). Characteristics, hazard and prevention of the parasitic weed Cassytha filiformis. Weed Sci. 3, 4–5.
Liu, Y., Oduor, A. M., Zhang, Z., Manea, A., Tooth, I. M., Leishman, M. R., et al. (2017). Do invasive alien plants benefit more from global environmental change than native plants? Glob. Change Biol. 23, 3363–3370. doi: 10.1111/gcb.13579
Liu, Z. F., Ma, H., Ci, X. Q., Li, L., Song, Y., Liu, B., et al. (2021). Can plastid genome sequencing be used for species identification in Lauraceae? Bot. J. Linn. Soc. 197, 1–14. doi: 10.1093/botlinnean/boab018
Maciunas, E. C., Watling, J. R., Facelli, J. M., and Packer, J. G. (2022). Seed traits and fate support probable primary dispersal of a native hemi-parasitic vine Cassytha pubescens (Lauraceae) by Isoodon obesulus, an endangered marsupial, in southern Australia. Trans. R. Soc. S. Aust. doi: 10.1080/03721426.2022.2050507 [Epub ahead of print].
MacLaren, C., Storkey, J., Menegat, A., Metcalfe, H., and Dehnen-Schmutz, K. (2020). An ecological future for weed science to sustain crop production and the environment. a review. Agron. Sustain. Dev. 40:24. doi: 10.1007/s13593-020-00631-6
Mahadevan, N., and Jayasuriya, K. M. G. G. (2013). Water-impermeable fruits of the parasitic angiosperm Cassytha filiformis (Lauraceae): confirmation of physical dormancy in Magnoliidae and evolutionary considerations. Aust. J. Bot. 61, 322–329. doi: 10.1071/BT12275
Maistry, P. M., Muasya, A. M., and Valentine, A. J. (2015). Balanced allocation of organic acids and biomass for phophorus and nitrogen demand in the fynbos legume Podalyria calyptrata. J. Plant Physiol. 174, 16–25. doi: 10.1016/j.jplph.2014.10.005
Manrique, V., Cuda, J. P., Overholt, W. A., and Ewe, S. M. L. (2009). Synergistic effect of insect herbivory and plant parasitism on the performance of the invasive tree Schinus terebinthifolius. Entomol. Exp. Appl. 132, 118–125. doi: 10.1111/j.1570-7458.2009.00875.x
Marcone, C., Ragozzino, A., and Seemuller, E. (1997). Dodder transmission of alder yellows phytoplasma to the experimental host Catharanthus roseus (periwinkle). Eur. J. For. Pathol. 27, 347–350. doi: 10.1111/j.1439-0329.1997.tb01449.x
McLuckie, J. (1924). Studies in parasitism. I. A contribution to the physiology of the genus Cassytha, Part 1. Proc. Linn. Soc. N. S. W. 49, 55–78.
Meek, C. S., Richardson, D. M., and Mucina, L. (2013). Plant communities along the Eerste River, Western Cape, South Africa: community descriptions and implications for restoration. Koedoe 55:14. doi: 10.4102/koedoe.v55i1.1099
Miller, A. C., Watling, J. R., Overton, I. C., and Sinclair, R. (2003). Does water status of Eucalyptus largiflorens (Myrtaceae) affect infection by the mistletoe Amyema miquelii (Loranthaceae)? Funct. Plant Biol. 30, 1239–1247. doi: 10.1071/FP03117
Musselman, L. J., and Press, M. C. (1995). “Introduction to parasitic plants,” in Parasitic Plants, eds M. C. Press and J. D. Graves (London: Chapman & Hall), 1–13.
Mythili, S., Gajalakshmi, S., Sathiavelu, A., and Sridharan, T. B. (2011). Pharmacological activities of Cassytha filiformis: a review. Asian J. Plant Sci. Res. 1, 77–83.
Nayar, B. K., and Nayar, P. N. (1952). On the range of the hosts of Cassytha filiformis Linn. Sci. Cult. 17, 383–384.
Nickrent, D. L. (2020). Parasitic angiosperms: how often and how many? Taxon 69, 5–27. doi: 10.1002/tax.12195
Nickrent, D. L., and Musselman, L. J. (2004). Introduction to parasitic flowering plants. Plant Health Instr. doi: 10.1094/PHI-I-2004-0330-01
Novotna, B., Polesny, Z., Pinto-Basto, M. F., Damme, P. V., Pudil, P., Mazancova, J., et al. (2020). Medicinal plants used by ‘root doctors’, local traditional healers in Bié province, Angola. J. Ethnopharmacol. 260:112662. doi: 10.1016/j.jep.2020.112662
Nugraha, A. S., Triatmoko, B., Wangchuk, P., and Keller, P. A. (2020). Vascular epiphytic medicinal plants as sources of therapeutic agents: their ethnopharmacological uses, chemical composition, and biological activities. Biomolecules 10:181. doi: 10.3390/biom10020181
Olson, D. M., and Dinerstein, E. (2002). The Global 200: priority ecoregions for global conservation. Ann. Mo. Bot. Gard. 89, 125–126. doi: 10.2307/3298564
Paine, R. T. (1969). A note on trophic complexity and community stability. Am. Nat. 103, 91–93. doi: 10.1086/282586
Pederick, L. A., and Zimmer, W. J. (1961). The Parasitic Forest Dodder-Laurel Cassytha melantha. Bulletin No. 12. Melbourne, VIC: Forests Commission of Victoria.
Phoenix, G. K., and Press, M. C. (2005). Linking physiological traits to impacts on community structure and function: the role of root hemiparasitic Orobanchaceae (ex-Scrophulariaceae). J. Ecol. 93, 67–78. doi: 10.1111/j.1365-2745.2004.00950.x
Press, M. C., and Phoenix, G. K. (2005). Impacts of parasitic plants on natural communities. New Phytol. 166, 737–751. doi: 10.1111/j.1469-8137.2005.01358.x
Prider, J., Watling, J., and Facelli, J. M. (2009). Impacts of a native parasitic plant on an introduced and a native host species: implications for the control of an invasive weed. Ann. Bot. 103, 107–115. doi: 10.1093/aob/mcn214
Prider, J. N., Facelli, J. M., and Watling, J. R. (2011). Multispecies interactions among a plant parasite, a pollinator and a seed predator affect the reproductive output of an invasive plant, Cytisus scoparius. Aust. Ecol. 36, 167–175. doi: 10.1111/j.1442-9993.2010.02132.x
Quetin-Leclercq, J., Hoet, S., Block, S., Wautier, M. C., and Stevigny, C. (2004). “Studies on Cassytha filiformis from Benin: isolation, biological activities and quantification of aporphines,” in Proceedings of Bioresources Towards Drug Discovery and Development. (Réduit: University of Mauritius), 81–106.
Rafferty, N. E., Agnew, L., and Nabity, P. D. (2019). Parasitism modifies the direct effects of warming on a hemiparasite and its host. PLoS One 14:e0224482. doi: 10.1371/journal.pone.0224482
Rajanna, L., and Shivamurthy, G. R. (2001). Occurrence of graniferous tracheary elements in the haustorium of Cassytha filiformis Linn., a stem parasite of Lauraceae. Tanwania 46, 40–48.
Schroeder, C. A. (1967). The stem parasite Cassytha filiformis a botanical relative of avocado. Calif. Avocado Soc. Yearb. 51, 159–160.
Shen, H., Prider, J. N., Facelli, J. M., and Watling, J. R. (2010). The influence of the hemiparasitic angiosperm Cassytha pubescens on photosynthesis of its host Cytisus scoparius. Funct. Plant Biol. 37, 14–21. doi: 10.1071/FP09135
Shen, H., Xu, S.-J., Hong, L., Wang, Z.-M., and Ye, W.-H. (2013). Growth but not photosynthesis response of a host plant to infection by a holoparasitic plant depends on nitrogen supply. PLoS One 8:e75555. doi: 10.1371/journal.pone.0075555
Shen, H., Ye, W., Hong, L., Huang, H., Wang, Z., Deng, X., et al. (2006). Progress in parasitic plant biology: host selection and nutrient transfer. Plant Biol. 8, 175–185. doi: 10.1055/s-2006-923796
Song, Y., Yu, W. B., Tan, Y., Liu, B., Yao, X., Jin, J., et al. (2017). Evolutionary comparisons of the chloroplast genome in Lauraceae and insights into loss events in the magnoliids. Genome Biol. Evol. 9, 2354–2364. doi: 10.1093/gbe/evx180
Spasojevic, M. J., and Suding, K. N. (2011). Contrasting effects of hemiparasites on ecosystem processes: can positive litter effects offset the negative effects of parasitism? Oecologia 165, 193–200. doi: 10.1007/s00442-010-1726-x
Teixeira-Costa, L., and Davis, C. C. (2021). Life history, diversity, and distribution in parasitic flowering plants. Plant Physiol. 187, 32–51. doi: 10.1093/plphys/kiab279
Tennakoon, K. U., Pate, J. S., and Arthur, D. (1997). Ecophysiological aspects of the woody root hemiparasite Santalum acuminatum (R. Br.) A. DC and its common hosts in South Western Australia. Ann. Bot. 80, 245–256. doi: 10.1006/anbo.1997.0432
Tennakoon, K. U., Rosli, R., and Le, Q. V. (2016). Biology of aerial parasitic vines in Brunei Darussalam: Cuscuta and Cassytha. Sci. Bruneiana 15, 58–64.
Těšitel, J. (2016). Functional biology of parasitic plants: a review. Plant Ecol. Evol. 149, 5–20. doi: 10.5091/plecevo.2016.1097
Těšitel, J., Cirocco, R. M., Facelli, J. M., and Watling, J. R. (2020). Native parasitic plants: biological control for plant invasions? Appl. Veg. Sci. 23, 464–469. doi: 10.1111/avsc.12498
Těšitel, J., Li, A. R., Knotková, K., McLellan, R., Bandaranayake, P. C. G., and Watson, D. M. (2021). The bright side of parasitic plants: what are they good for? Plant Physiol. 185, 1309–1324. doi: 10.1093/plphys/kiaa069
Těšitel, J., Plavcová, L., and Cameron, D. D. (2010). Interactions between hemiparasitic plants and their hosts. Plant Signal. Behav. 5, 1072–1076. doi: 10.4161/psb.5.9.12563
Thang, P. T., Vien, N. V., and Khanh, T. D. (2021). Allelopathic potential of an invasive plant (Cassytha filiformis L.) under different assessing conditions. Plant Cell Biotechnol. Mol. Biol. 22, 82–94.
Tsang, H. T. (2010). Cassytha pubescens: Germination Biology and Interactions with Native and Introduced Hosts. Master thesis. Adelaide, SA: University of Adelaide.
Wapstra, M., Schahinger, R., and Larcombe, L. (2009). Threatened Flora Extension Surveys, King Island 23-26 March 2009. A Report to the Cradle Coast Natural Resource Management Committee. Hobart, TAS: Threatened Species Section, Department of Primary Industries and Water.
Watling, J. R., and Press, M. C. (2000). Infection with the parasitic angiosperm Striga hermonthica influences the response of the C3 cereal Oryza sativa to elevated CO2. Glob. Change Biol. 6, 919–930. doi: 10.1046/j.1365-2486.2000.00366.x
Watling, J. R., and Press, M. C. (2001). Impacts of infection by parasitic angiosperms on host photosynthesis. Plant Biol. 3, 244–250. doi: 10.1055/s-2001-15195
Weber, J. Z. (1981). A taxonomic revision of Cassytha (Lauraceae) in Australia. J. Adelaide Bot. Gard. 3, 187–262.
Weber, J. Z. (2007). “Cassytha,” in Flora of Australia 2, ed. A. G. Wilson (Melbourne, VIC: CSIRO Publishing), 117–136.
Werth, C. R., Pusateri, W. P., Eshbaugh, W. H., and Wilson, T. K. (1979). “Field observations on the natural history of Cassytha filiformis L. (Lauraceae) in the Bahamas,” in The 2nd International Symposium on Parasitic Weeds, eds L. J. Musselman, A. D. Worsham, and R. E. Eplee (Raleigh, NC: North Carolina State University), 94–102.
Yao, D., Zheng, X., Huang, J., and Li, Y. (1994). Changes of acid phosphatase and cytokinins during haustorial development on the parasitic plant Cassytha filiformis L. Acta Bot. Sin. 36, 170–174.
Zagorchev, L., Stöggl, W., Teofanova, D., Li, J., and Kranner, I. (2021). Plant parasites under pressure: effects of abiotic stress on the interactions between parasitic plants and their hosts. Int. J. Mol. Sci. 22, 7418. doi: 10.3390/ijms22147418
Zamora, R., and Mellado, A. (2019). Identifying the abiotic and biotic drivers behind the elevational distribution shift of a parasitic plant. Plant Biol. 21, 307–317. doi: 10.1111/plb.12934
Zhang, Z., Liu, Y., Yuan, L., Weber, E., and van Kleunen, M. (2021). Effect of allelopathy on plant performance: a meta-analysis. Ecol. Lett. 24, 348–362. doi: 10.1111/ele.13627
Keywords: aerial parasite, Cassytha filiformis, Cassytha pubescens, environmental change, haustorium, plant infection, nutrient transfer
Citation: Zhang H, Florentine S and Tennakoon KU (2022) The Angiosperm Stem Hemiparasitic Genus Cassytha (Lauraceae) and Its Host Interactions: A Review. Front. Plant Sci. 13:864110. doi: 10.3389/fpls.2022.864110
Received: 28 January 2022; Accepted: 29 April 2022;
Published: 06 June 2022.
Edited by:
Victoria Borowicz, Illinois State University, United StatesReviewed by:
Junmin Li, Taizhou University, ChinaMuthama Muasya, University of Cape Town, South Africa
Robert Cirocco, The University of Adelaide, Australia
Copyright © 2022 Zhang, Florentine and Tennakoon. This is an open-access article distributed under the terms of the Creative Commons Attribution License (CC BY). The use, distribution or reproduction in other forums is permitted, provided the original author(s) and the copyright owner(s) are credited and that the original publication in this journal is cited, in accordance with accepted academic practice. No use, distribution or reproduction is permitted which does not comply with these terms.
*Correspondence: Kushan U. Tennakoon, k.tennakoon@federation.edu.au
†ORCID: Hongxiang Zhang, orcid.org/0000-0003-0907-843X; Singarayer Florentine, orcid.org/0000-0002-5734-3421; Kushan U. Tennakoon, orcid.org/0000-0001-9019-968X