- 1Faculty of Forestry and Wood Sciences, Czech University of Life Sciences Prague, Prague, Czechia
- 2Department of Immunotechnology, Lund University, Lund, Sweden
- 3National Bioinformatics Infrastructure Sweden (NBIS), Science for Life Laboratory, Lund University, Lund, Sweden
The bark beetle, Ips typographus (L.), is a major pest of Norway spruce, Picea abies (L.), causing enormous economic losses globally. The adult stage of the I. typographus has a complex life cycle (callow and sclerotized); the callow beetles feed ferociously, whereas sclerotized male beetles are more aggressive and pioneers in establishing new colonies. We conducted a comparative proteomics study to understand male and female digestion and detoxification processes in callow and sclerotized beetles. Proteome profiling was performed using high-throughput liquid chromatography-mass spectrometry. A total of >3000 proteins were identified from the bark beetle gut, and among them, 539 were differentially abundant (fold change ±2, FDR <0.05) between callow and sclerotized beetles. The differentially abundant proteins (DAPs) mainly engage with binding, catalytic activity, anatomical activity, hydrolase activity, metabolic process, and carbohydrate metabolism, and hence may be crucial for growth, digestion, detoxification, and signalling. We validated selected DAPs with RT-qPCR. Gut enzymes such as NADPH-cytochrome P450 reductase (CYC), glutathione S-transferase (GST), and esterase (EST) play a crucial role in the I. typographus for detoxification and digesting of host allelochemicals. We conducted enzyme activity assays with them and observed a positive correlation of CYC and GST activities with the proteomic results, whereas EST activity was not fully correlated. Furthermore, our investigation revealed that callow beetles had an upregulation of proteins associated with juvenile hormone (JH) biosynthesis and chitin metabolism, whereas sclerotized beetles exhibited an upregulation of proteins linked to fatty acid metabolism and the TCA cycle. These distinctive patterns of protein regulation in metabolic and functional processes are specific to each developmental stage, underscoring the adaptive responses of I. typographicus in overcoming conifer defences and facilitating their survival. Taken together, it is the first gut proteomic study comparing males and females of callow and sclerotized I. typographus, shedding light on the adaptive ecology at the molecular level. Furthermore, the information about bark beetle handling of nutritionally limiting and defence-rich spruce phloem diet can be utilized to formulate RNAi-mediated beetle management.
Introduction
Tree-killing bark beetles are a significant disturbance in biomes and temperate climates, impacting the function, composition, and structure of forests (Vindstad et al., 2019; Vindstad et al., 2020; Hlásny et al., 2021b). In Europe, Ips typographus (L.) is a significant insect pest of Norway spruce Picea abies (L.) H. Karst trees, causing substantial economic losses (Christiansen and Bakke, 1988; Hlásny et al., 2021a). The Norway spruce is critical species for the wood and timber industry because the damage caused by I. typographus outbreaks can have a cascade effect on forest economies and the global timber market, as well as environmental impacts, carbon sink, and well-being of humans (Seidl et al., 2014; Grégoire et al., 2015; Aranda et al., 2017; Morris et al., 2017; Montagné-Huck and Brunette, 2018; Hlásny et al., 2019; Schuldt et al., 2020; Senf et al., 2020). The bark beetle epidemic was reported in various European nations, including Germany, France, and Austria, but the Czech Republic became the epicentre of the bark beetle attack (Hlásny et al., 2019; Gao et al., 2020; Jandl, 2020; Toth et al., 2020; Hlásny et al., 2021a; Senf and Seidl, 2021; Sommerfeld et al., 2021). In 2015, a severe outbreak started in Czech Republic, and the losses have exceeded over the last two centuries. This outbreak can be called ecological collapse or failure of spruce-oriented management. Adult host colonization and overwintering may be critical in increasing the number of bark beetle attacks and their economic impact (Senf and Seidl, 2021). Superior knowledge of the molecular mechanisms underlying its adaptation to host allelochemicals can facilitate its management via RNAi (Joga et al., 2021; Mogilicherla and Roy, 2023).
Researchers have applied multiple approaches to explore insect resistance and host adaptation mechanisms, such as measuring biochemical and genetic differences, altering protein expression, etc. Biochemical techniques, including colourimetry, successfully identify and quantify detoxification enzymes such as esterase, glutathione S-transferase, cytochrome, etc. (Bosch-Serra et al., 2021). Transcriptomic approaches reflect organism biology because they allow the investigation of genes that are expressed in a tissue or organism under certain conditions. However, levels of proteins and enzymes are sometimes hard to predict from corresponding mRNA expression, whereas proteomics can deliver more reliable information about the physiological state of an organism (Huber, 2003). In the mountain pine beetle (Dendroctonus ponderosae Hopkins), the integrated analysis of expressed sequence tag (EST) and proteomics data showed several proteins were to be involved in the reproduction and stress physiology of insect survival during the early stages of the host colonization phase of their life cycle (Pitt et al., 2014). The proteomics and transcriptomics approaches were used to study the overwintering physiology (insects living under the tree’s bark must endure protracted periods of extreme cold) and early host colonization (insects must surpass host defences to procreate) of the mountain pine beetle (D. ponderosae Hopkins) (Huber and Robert, 2016). Quantitative metabolome, proteome, and transcriptome analysis of juvenile hormone III treated male/female midgut and fat body tissues of mountain pine beetle (D. ponderosae Hopkins) revealed JH III stimulates frontalin biosynthesis in males and trans-verbenol biosynthesis in females (Keeling et al., 2016). Recently, our group performed a thorough analysis of the I. typographus transcriptome data to comprehend physiology and adaptation and unravel the dynamics of gene expression in various life stages [larvae (L1, L2, and L3), pupa, callow, and sclerotized adult] and male/female tissues (gut, fat body, and head) from callow and sclerotized beetles (Naseer et al., 2023). Unfortunately, no proteomic study on the Eurasian tree-killing bark beetle delineates the digestive and adaptive physiology during the adult stage. We are interested in the protein expression dynamics in the guts of I. typographus males and females of callow (freshly emerged from the pupa and appeared yellow) and sclerotized (fully matured and appeared black) beetles to follow up existing transcriptomics studies and understand beetle adaptive physiology better. Callow beetles perform maturation feeding and need to digests tons of plant materials whereas sclerotized beetles (males for I. typographus) are pioneers in finding a new host for colonization and are later accompained by females to produce the next generation of beetles. Hence, these two stages are the focal point of the current proteomic study. The present study identified differentially abundant proteins (DAPs) in the guts of male and female adult beetles at callow and sclerotized stages. It will aid in understanding detoxification, digestion, signalling, and growth mechanisms in this economically significant forest pest of spruce and serve as a foundation for future studies.
Materials and methods
Insect collection, rearing, and sample collection
Naturally infested spruce stems with I. typographus were collected from the forest (Kostelec nad Černými lesy, 50°00’07.2”N 14°50’56.3”E, Czech Republic, under School forest enterprise, semi-warm and dry area) and kept in the university insect breeding room at the Czech university of life sciences Prague, Czech Republic. After emerging, beetles were reared on spruce logs in plastic boxes under laboratory conditions of 25 ± 2°C and 70% relative humidity. Logs were peeled, and callow beetles were collected from spruce logs after 5 weeks of infestation, whereas sclerotized beetles were collected after emerging from spruce logs, and both stages were sexed into males and females based on pronotum hair density (Schlyter and Cederholm, 1981). A total of 500 males and females of callow and sclerotized beetles were collected and stored at -80°C for further study.
Dissection and beetle gut collection
The frozen beetles were thawed and dissected. The guts were collected in an ice-cold 1× phosphate buffer solution (PBS) with a protease inhibitor cocktail. Fifteen beetle guts were pooled for each replicate, and four replicates were prepared for each sample.
Protein extraction, quantification, and SDS PAGE
Gut samples were homogenized in 50 µL of lysis buffer (100 mM PBS, 5mM DTT, and protease inhibitor cocktail) and centrifuged at 13000 rpm at room temperature for 15 minutes. The supernatant was separated, the protein concentration was determined using the Bradford assay (Bradford, 1976), and the standard was a serial dilution of bovine serum albumin (R2 = 0.942). Each biological replicate contains 50 µg of total gut protein mixed with 2× loading buffer and boiled for 5 minutes before loading onto a 5% SDS PAGE stacking gel as per preoptimized protocol (Roy et al., 2014; Roy and Das, 2015). The SDS-PAGE gel was run for 10 mins until the total protein band reached the resolving gel (10%). Subsequently, protein gels were stained using Coomassie brilliant blue R250. The protein bands from the gel were cut into 1mm pieces and stored in 0.5x destaining solution (ethanol, glacial acetic acid, and proteomic-graded water) at 4°C after separation for 10 mins at a constant 50V (Supplementary Figure 1).
In-gel digestion
Each gel lane containing a single unseparated total protein (50 µg) band was cut and washed twice with acetonitrile (ACN) and ammonium bicarbonate (AMBIC) (Supplementary Figure 1). Gel pieces were then reduced and alkylated by 10 mM DL-dithiothreitol (DTT) and 55 mM iodoacetamide (IAA) correspondingly. Proteins were digested by porcine-modified trypsin in a 1:50 w/w ratio with incubation overnight at 37°C. Finally, peptides were extracted from gel pieces with 70% ACN/5% formic acid (FA) and dried out in the SpeedVac (Thermo Fisher Scientific) (Shevchenko et al., 2006; Roy et al., 2014).
Mass spectrometry analysis
The dried peptides were dissolved in 0.1% FA and quantified using nanodrop (Thermo Fisher Scientific). Approximately 400 ng peptides per sample were separated by liquid chromatography on an Evosep One system (Evosep, Odense), connected to a QExactive HF-X mass spectrometer (Thermo Fisher Scientific). A 15 cm long fused silica capillary (75 μm* 16 cm Pico Tip Emitter, New Objective) was utilized as the analytical column, packed in-house with C18 material ReproSil-Pur 1.9 μm (Dr Maisch, GmbH, Germany). Peptides were separated using the Whisper 58 min gradient pre-programmed for 20 samples per day. The mass spectrometer was operated in positive ion mode using data-dependent acquisition with an automated gain control (AGC) target value of 3×106 ions and a maximum fill time of 50 ms in a scan range of 375 to 1500 m/z for the entire MS scans. A top-20 method was used for fragmentation (collision energy: 40V) by higher energy collision-induced dissociation (HCD) using an isolation window of 1.2 m/z and a target value of 1×105 ions with a maximum injection period of 20 ms. MS/MS spectra were collected at a resolution of 15,000 full-width half-maximum (FWHM).
Mass spectrometry data processing
The resulting RAW files were processed for label-free quantification using MaxQuant, version 1.6.17.0 (www.maxquant.org). The bark beetle fasta file created in-house from the published genome of I. typographus (Powell et al., 2021) was used as the search database. Cysteine carbamidomethylation was set as a fixed modification, whereas methionine oxidation was selected as a variable modification. Default settings were used, including protein level filtering at FDR < 0.01. The search results and raw data have been deposited at ProteomeXchange via the PRIDE partner repository with protein accession PXD035407 (Project Name: Bark beetle gut proteomics study). The search parameters are available with the deposited data.
Proteomics data analysis
Protein group quantity values from MaxQuant were normalized using Cyclic Loess-normalization in NormalyzerDE version 1.10.0 (Willforss et al., 2018). The log2-transformed normalized data were utilized for visualization and statistical tests to identify differentially abundant peptides (Bengtsson et al., 2014). The differential abundance analysis was performed using LIMMA Empirical Bayes statistics in NormalyzerDE. We filtered DAPs with an Adjusted p-value <0.05 and a fold difference ±2 times and sorted them for further analysis and verification (Supplementary Excel File 1). Functional analysis of DAPs was carried out using gene ontology (GO) analysis, and the DAPs were grouped according to the biological process, molecular function, and cellular component. Further, functional annotations of DAPs were classified through KEGG ontology assignments using the Blast2GO platform to study the biological functions and pathways using preoptimized pipeline (Conesa et al., 2005; Roy et al., 2016).
Protein preparation and measurement of enzyme activity
Adult beetles (sclerotized and callow) were collected from logs and sexed into male and female. Four individual replicates were prepared, and for each replicate, 10 beetles were dissected, extracted in the guts, and homogenized into 100 µL sodium phosphate buffer (50 mM, pH 7) depending on the requirement of the enzymatic concentration (Smagghe and Tirry, 2001). Initial homogenization was performed manually with the help of a homogenizer at 4°C in the Eppendorf tube. Supernatants were separated by centrifuging homogenized extracts for 15 mins at 12000 g and 4°C (Z32 HK, Hermle, Newyork, USA) (Berrada et al., 1994). The supernatant was collected individually and stored at -20°C before further analysis. Protein quantification was done using the Bradford method (Bradford, 1976). The colorimetric method and substrates for each enzymatic assay were used to analyze the activity of enzymes related to detoxification and digestion (GST, CYC, and EST) in beetles. All the enzymatic activities were studied based on changes in absorbance at 30°C using a VICTOR3™ Multilabel microplate reader (PerkinElmer Life and Analytical Sciences, Madrid, Spain) (Lehninger, 1950). To evaluate the impact of protein concentration on enzyme activity, we conducted an enzymatic experiment using different concentrations of enzyme sources (0.5, 1, 2, and 4 g of total protein) at varying time intervals (1 to 10 minutes) following the addition of the substrate.
To measure glutathione S-transferase (GST) activity, 20 µl of enzyme source and 170 µl of 5mM GSH (dissolved in 50 mM sodium phosphate buffer) were added to a 96-well transparent plate. The plate was preincubated at 30°C for 60 minutes, followed by the addition of 10 µl of 30mM CDNB (dissolved in 100% ethanol); the colour development was monitored at 340 nm every minute for a 30-minute incubation, maintaining a temperature of 25-30°C (Habig and Jakoby, 1981; Bosch-Serra et al., 2021). The enzyme activity was quantified as a nmol substrate conjugated.min-1.mg-1 protein, utilizing a CDNB molar extinction coefficient of 9.6 mM-1 × cm-1 (Habig and Jakoby, 1981).
To assess Cytochrome P450 Reductase (CYC) activity, 20 µL of enzyme extract was combined with 160 µL of NADPH solution (containing 0.6 mM NADP, 2.8 mM G6P, and 0.28 units of G6PD) in a 96-well plate, followed by a preincubation at 30°C for 30 minutes. Subsequently, 20 µL of 1 mM cytochrome C solution (dissolved in 50 mM sodium phosphate buffer, pH 7) was added, and the resulting colour change was monitored at 550 nm at one-minute intervals for a duration of up to 25 minutes (Ortego et al., 1999; Bosch-Serra et al., 2021). The activity of CYC was quantified as a nmol substrate conjugated.min -1.mg-1 protein, employing a cytochrome C molar extinction coefficient of 27.6 mM-1 × cm-1 (Margoliash and Frohwirt, 1959). The enzymatic activity was measured in cytochrome P450 equivalent unit min–1 mg–1 of the total extracted protein.
The esterase (EST) activity was performed at 25°C in a total volume of 200 μl, comprising 2 μl of 100 mM substrate (p-nitrophenyl acetate), 10 μl of enzyme source, 8 μl of ethanol (99%), and 180 μl of Tris–HCl buffer (50 mM, pH 8.0). The reaction mixture without the addition of any enzyme served as the control. The experiment was carried out at 37°C for 5 minutes, and the assessment was performed using a colourimetric assay by measuring the absorbance at 410 nm (Li et al., 2020). Enzymatic activity was defined as one unit when 1 µmol of p-nitrophenol was released min -1. mg-1 protein, determined by referring to the α-naphthol standard curve (R2 = 0.952).
Linking gene expression at transcriptomics and protein level
Differentially expressed transcripts (DET) from our previously published data (Naseer et al., 2023) and DAPs (from the current study) above fold change ±2 and adjusted P-value ≤0.05 cutoff values were used to determine the correlation between proteomic and transcriptomic level expression of (Supplementary Excel File 2) on I. typographus gut genes. The Venn diagram was designed to find the genes commonly expressed between transcriptome and proteome analyses. A pie chart was also made with commonly expressed genes to identify the percentage of genes associated with detoxification, digestion, defence, signalling, and growth.
RNA extraction and cDNA synthesis
Four replicates from each male and female of callow and sclerotized beetles were prepared following preoptimized protocol (Sellamuthu et al., 2022), and each replicate contains five beetle guts. Total RNA was isolated from the males and females of the callow and sclerotized beetle’s guts using TRIzol® (Invitrogen, Carlsbad, CA) according to the manufacturer’s instructions. Further, to remove the DNA, extracted RNA was treated with TURBO™ DNase (Ambion, USA). The cDNA was synthesized from 1 μg RNA using High-Capacity cDNA Reverse Transcription kits (Applied Biosystems-Life Technologies) following the manufacturer’s instructions and stored at -20°C. Before the RT-qPCR reaction, the cDNA samples were diluted ten times with nuclease-free water.
Validation of DAPs by RT- qPCR
RT-qPCR reaction was performed in StepOnePlus™ Real-Time PCR System (Applied Biosystems, USA) with four independent biological replicates from guts of callow and sclerotized beetles. The master mix contains 5 µl SYBR® Green PCR Master Mix (Applied Biosystems, USA), 1 µl of diluted cDNA, 1 µl of gene-specific primers, and makes the final volume of 10 µl with nuclease-free water (Invitrogen, USA). All the samples were amplified under the following conditions: pre-degeneration at 95°C for 10 min, followed by 40 cycles of denaturation for 15 s at 95°C, and 60°C for 1 min. The primer’s specificity was confirmed by melt curve analysis using default parameters by a gradual increase in temperature from 60°C to 95°C. The 40S ribosomal protein S3-A was employed for data normalization as an internal control gene (Sellamuthu et al., 2022). The 2-ΔΔCt method was used for qRT-PCR data validation (Livak and Schmittgen, 2001).
Results
Proteomic analysis
The I. typographus males and females of callow and sclerotized beetle gut samples were used for the proteomic study (Supplementary Figure 1). Total proteins were prepared, digested, and analyzed through LC-MS analysis, identifying 3061 proteins. 539 DAPs were identified after deploying the cutoff (fold change ± 2 and FDR p-value < 0.05) (Figures 1A, B). Furthermore, 281 commonly regulated genes were discovered by comparing the differentially abundant proteins with pre-existing gut transcriptome data (Figure 2), signifying their physiological importance in the bark beetle gut. Most proteins were found to be differentially regulated between callow and sclerotized stages rather than between males and females of the same stages (Figures 3A, B), which is understandable. Furthermore, based on Gene Ontology (GO) classification, the DAPs were classified into three functional categories: biological process, molecular function, and cellular components (Supplementary Figure 2).
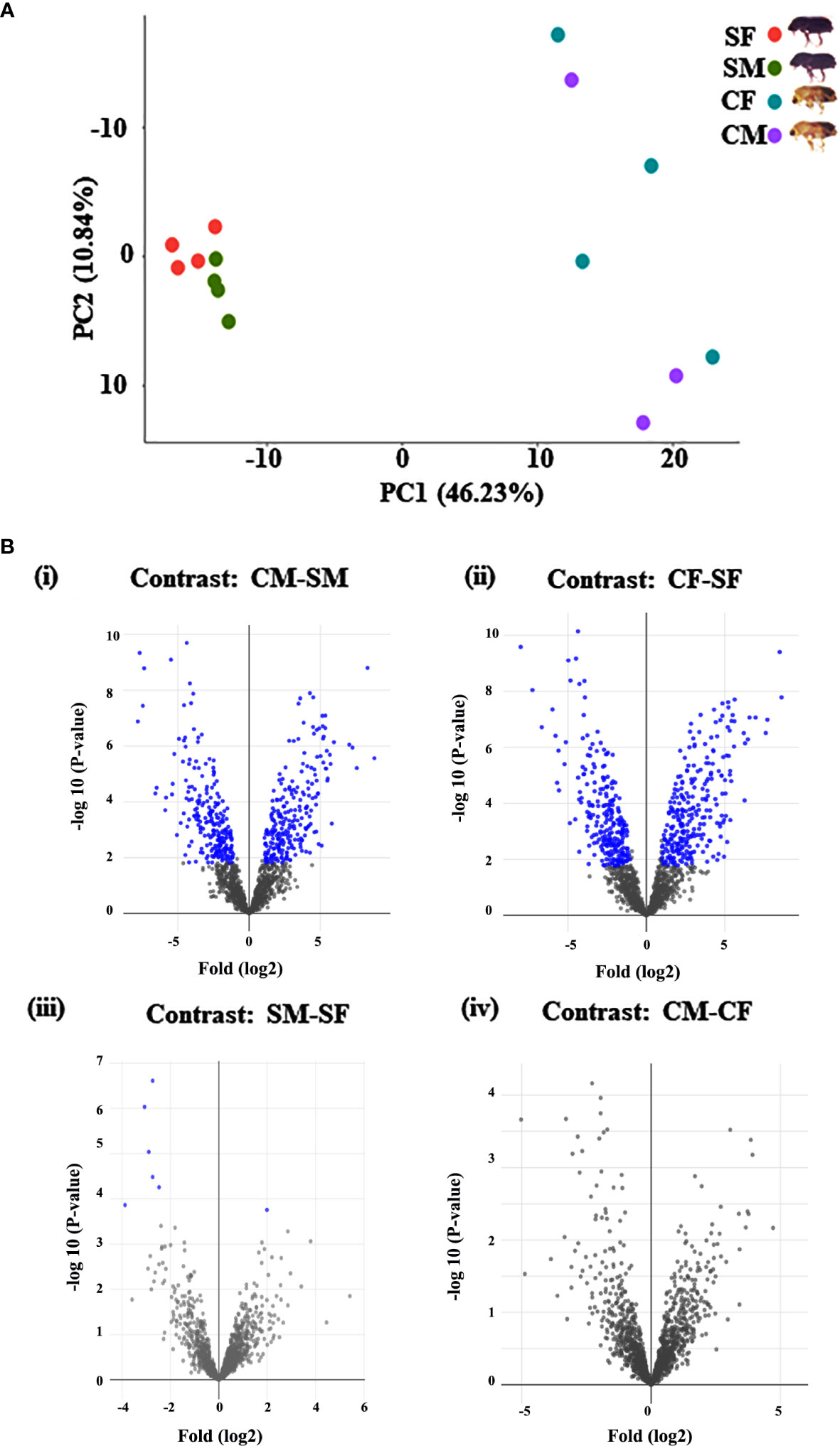
Figure 1 Graphical representation of quantitative proteomics data. (A) The PCA plot represents the uniformity of each group of samples of callow and sclerotized stages. Percentages indicate the total variance explained by the first and second PC. Dots of the same colour show the four biological replicates of each sample (*callow males have only three replicates). (B) Proteins are ranked in a volcano plot according to their statistical P-value (y-axis) and their relative abundance ratio (log2 fold change) between four comparisons (x-axis). Off-centred spots are those that vary the most between the groups. Blue dots represent the differentially abundant proteins based on Log2FC 1 (fold change 2) and FDR corrected p value <0.05 cut-offs. CM, callow male; CF, callow female; SM, sclerotized male; SF, sclerotized female.
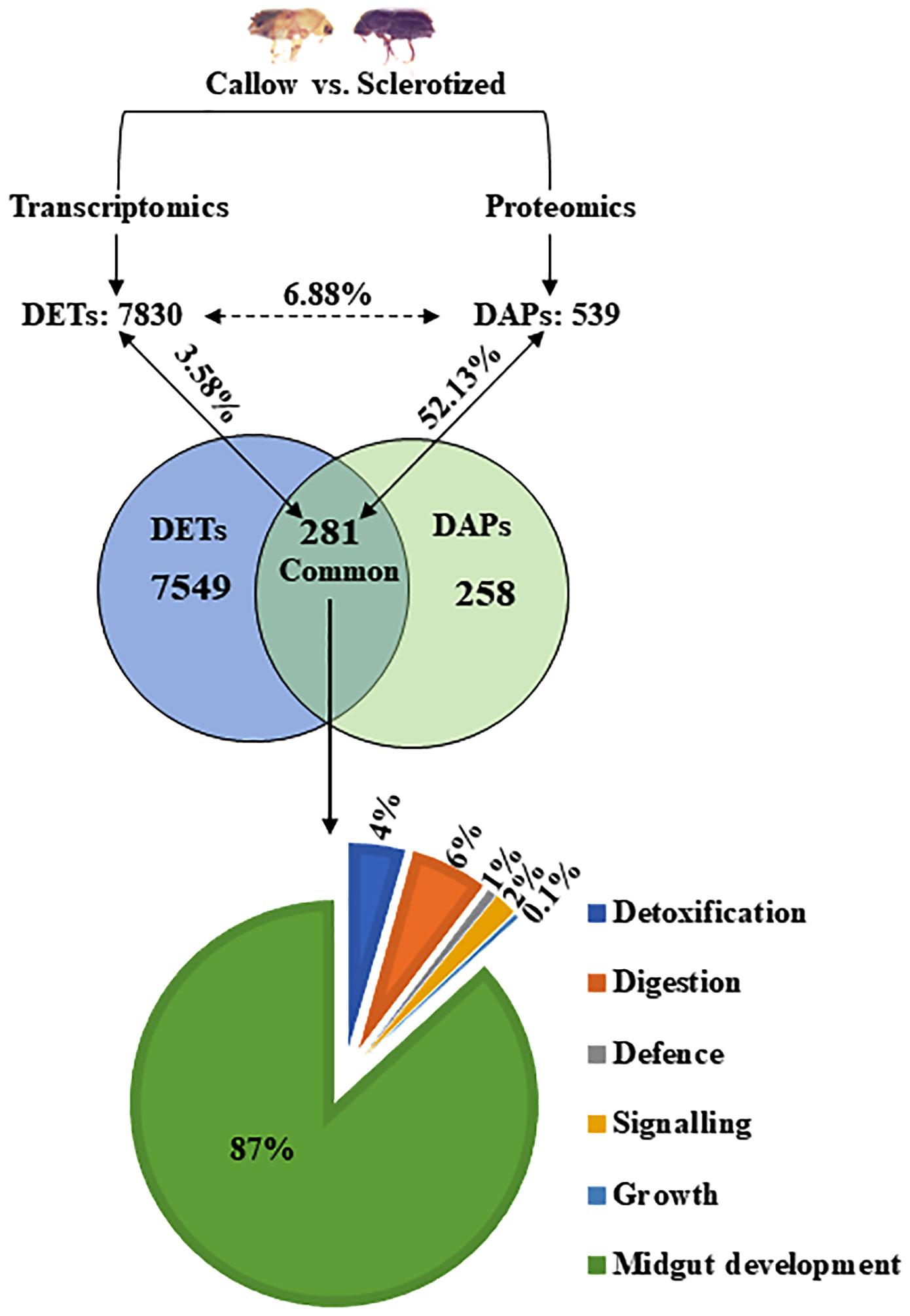
Figure 2 Correlation between DAPs and DETs in the gut (callow vs. sclerotized). The numerical value in each circle represents the number of transcripts or proteins, including identified transcripts and proteins related to gut expression and transcripts/proteins related to gut expression, respectively. DAPs, differentially abundant proteins; DETs, differentially expressed transcripts.
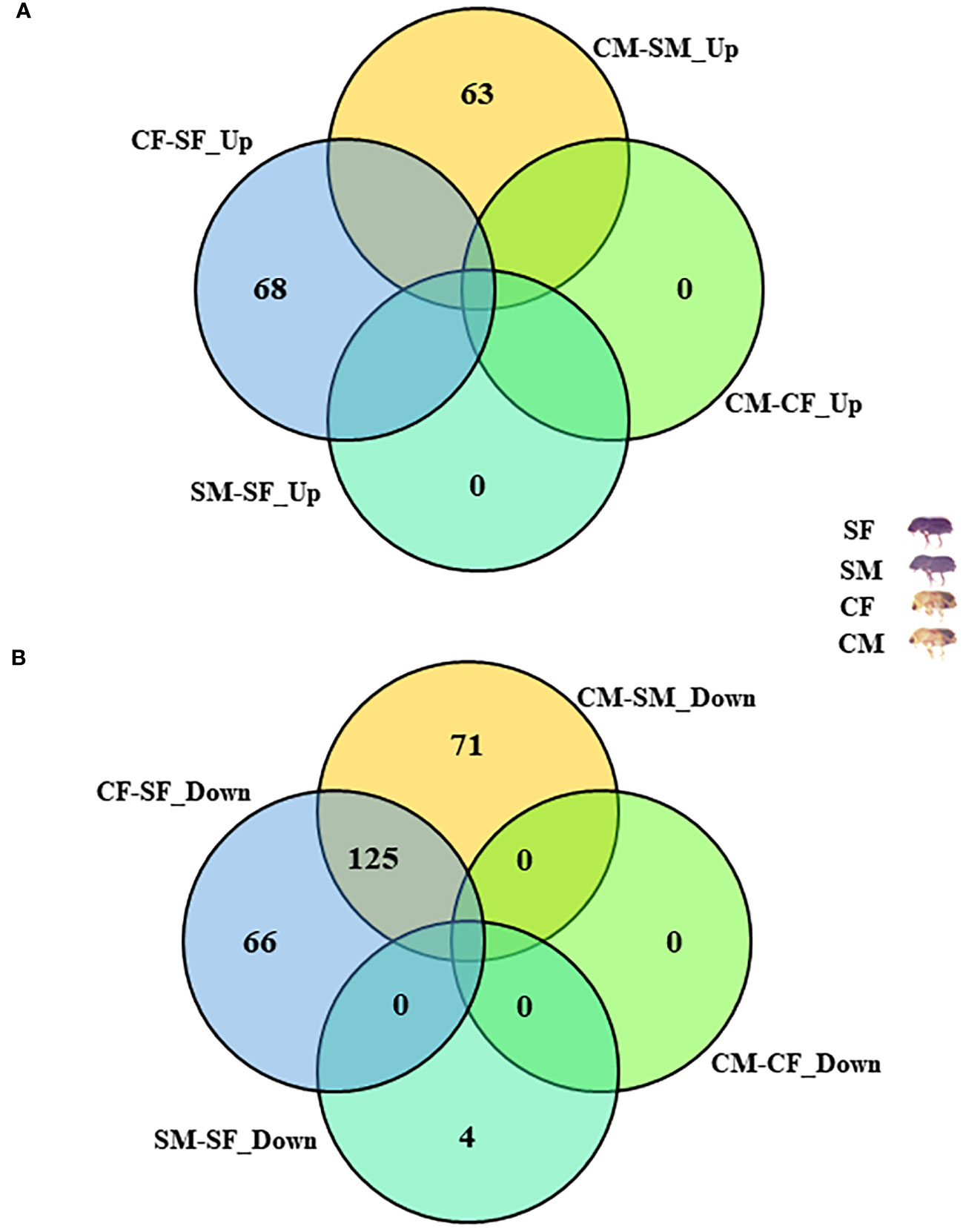
Figure 3 Comparative analysis with differentially abundant proteins (DAPs). (A) Distribution of common upregulated DAPs between four comparisons. (B) Distribution of common downregulated DAPs between four comparisons. CM, callow male; CF, callow female; SM, sclerotized male; SF, sclerotized female; DAPs, differentially abundant proteins.
Functional clustering for DAPs
We performed hierarchical clustering to identify the expression of DAPs between males and females of callow and sclerotized beetles. Hierarchical clustering was carried out using the average linkage approach with Euclidean distance based on log fold change data using Cluster 3.0 to depict the expression pattern of differentially abundant peptides. (Eisen et al., 1998). Significant up and down-regulation was observed in all DAPs between callow and sclerotized females (CF vs SF) and callow and sclerotized males (CM vs SM) comparisons (Figure 4). Although the DAPs almost overlapped, fold enrichment significantly differed between callow and sclerotized beetles, indicating that the magnitude of protein expression is sex-specific in response to ecological role and environmental challenges.
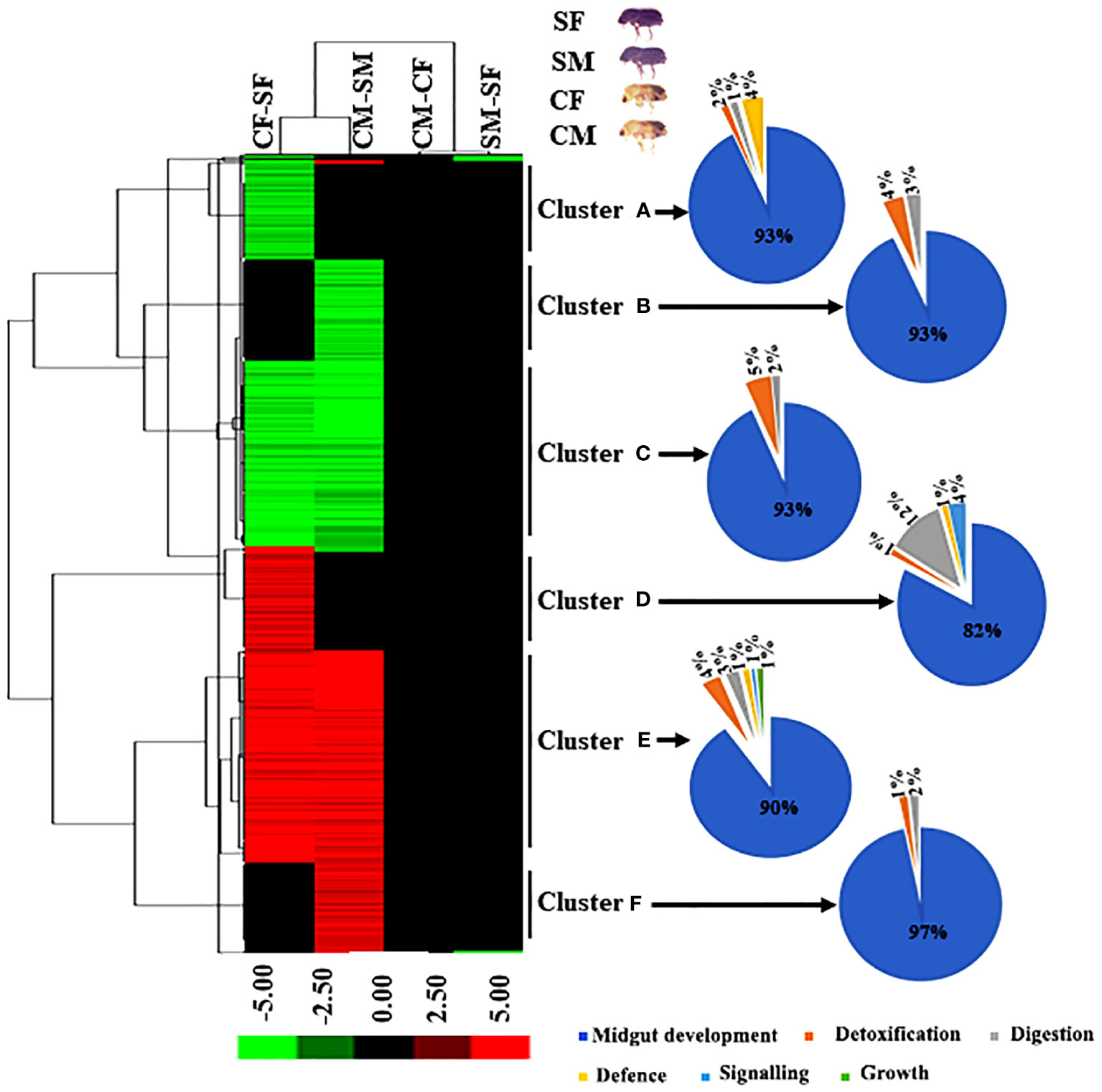
Figure 4 Hierarchical cluster analysis of DAPs in four comparisons. The letters (A-F) indicate major groups identified by cluster analysis. Red colour indicates upregulation (>2.0 fold), green colour indicates downregulation (<-2.0 fold), and black indicates no change as compared to respective controls. The Venn diagram represents the cluster-wise percentage of proteins related to detoxification, digestion, defence, signalling, and growth. CM, callow male; CF, callow female; SM, sclerotized male; SF, sclerotized female; DAPs, differentially abundant proteins.
DAPs vs. DETs comparison
539 DAPs from the proteomic study and 7830 DETs (differentially expressed transcripts) from the transcriptomic study (Naseer et al., 2023) were compared to relate the gene expression in callow vs. sclerotized beetle gut. The DAPs (539) and DETs (7830) were chosen to build a Venn diagram leading to the identification of 281 commonly expressed transcripts/proteins (Figure 2). The percentage of commonly expressed DETs was 3.58% (281 out of 7830), and DAPs were 52.13% (281 out of 539). Out of the identified 281 common ones, genes associated with detoxification (4%), digesting (6%), defence (1%), signalling (2%), and growth (0.1) were also represented on a pie chart (Figure 2).
DAPs validation by RT-qPCR and enzymatic assay
Twenty physiologically important DAPs connected to detoxification and digestion were chosen for RT-qPCR to validate the proteomic results (Supplementary Table 1; Figure 5). There was adequate agreement between the RT-qPCR and proteomic data regarding the expression patterns of the twenty selected DAPs, even if some differences were not significant in the RT-qPCR data. The outcomes demonstrated the robustness of the proteomic findings in the current investigation. CYC, GST, and EST enzyme activities were analyzed in the male and female guts of callow and sclerotized beetles (Figure 6). Different CYC, GST, and EST enzyme concentrations successfully converted substrate in a concentration-dependent manner (Supplementary Figure 3). The activity of CYC and GST-enzyme data was positively correlated with proteomic results, whereas EST-enzymatic data was not fully correlated and demanded further investigation.
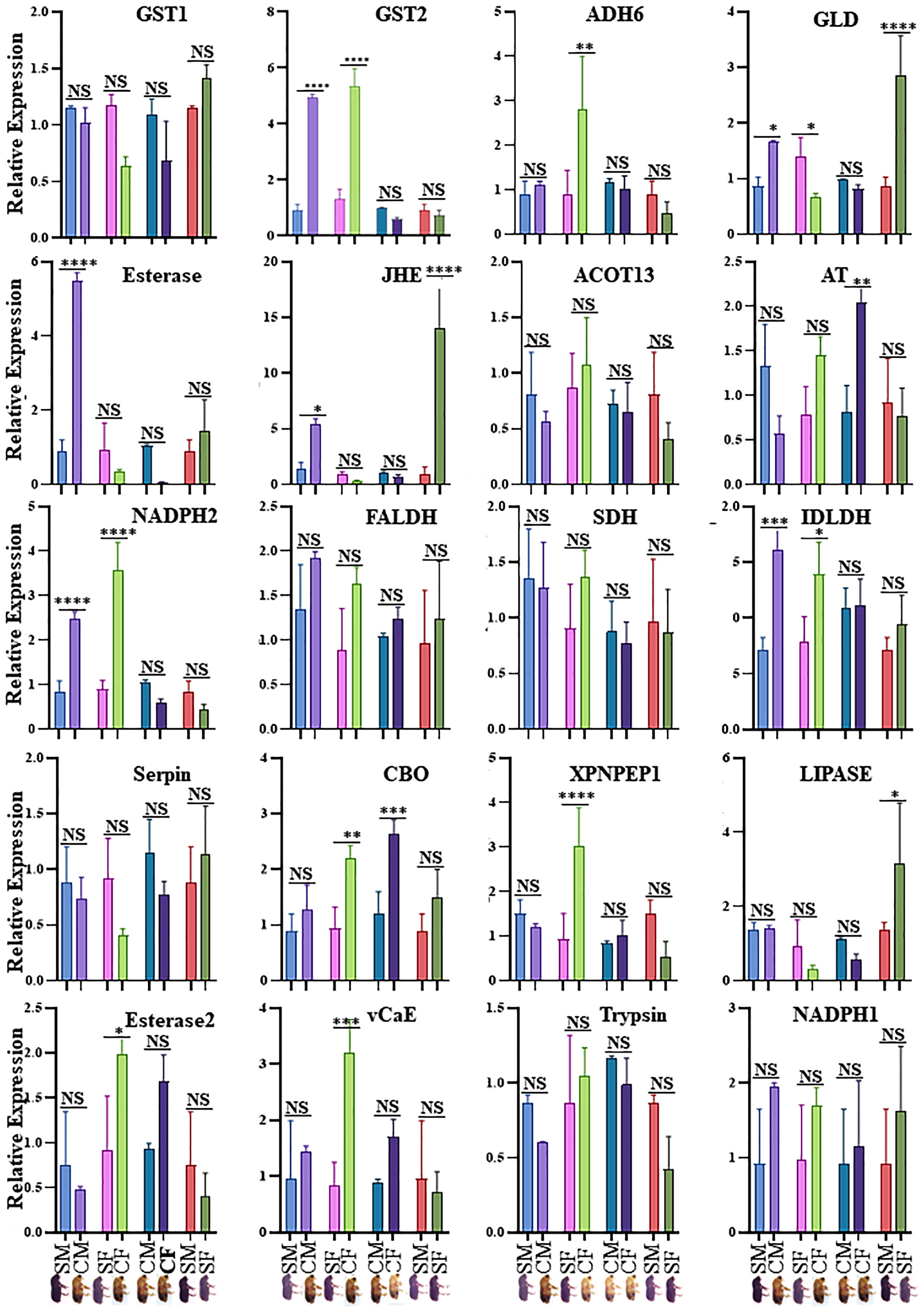
Figure 5 Validation of expression of genes obtained from proteomics analysis in four comparisons. SM- sclerotized male; CM-callow male; SF- sclerotized female; CF-callow female. The X-axis represents the sample names, and the Y-axis represents the relative expression. * P < 0.01; ** P < 0.001; *** P < 0.0006; **** P < 0.0001, NS- non-significant. GST1, Glutathione S-transferase, GST2, Glutathione S-transferase-like, ADH6, Alcohol dehydrogenase [NADP (+)]-like, GLD, Glucose dehydrogenase [acceptor], Esterase: Esterase B1-like, JHE, Juvenile hormone esterase, ACOT13, Acyl-coenzyme A thioesterase 13-like, AT, Antitrypsin-like isoform X4, NADPH2, NADH-ubiquinone oxidoreductase 75 kDa subunit, mitochondrial, FALDH, Fatty aldehyde dehydrogenase-like, SDH, Hypothetical protein D910_04066, IDLDH, IDLDH_IPSPIRecName, Full= Ipsdienol dehydrogenase, Serpin, Serine protease inhibitor 27A-like, CBQ, Carboxypeptidase Q-like, XPNPEP1, Xaa-Pro aminopeptidase 1, LIPASE, Lipase 3-like, Esterase2, Esterase E4-like, vCaE, Venom carboxylesterase-6-like, Trypsin, Trypsin precursor, NADPH1, NADPH, adrenodoxin oxidoreductase, mitochondrial. CM, callow male; CF, callow female; SM, sclerotized male; SF, sclerotized female.
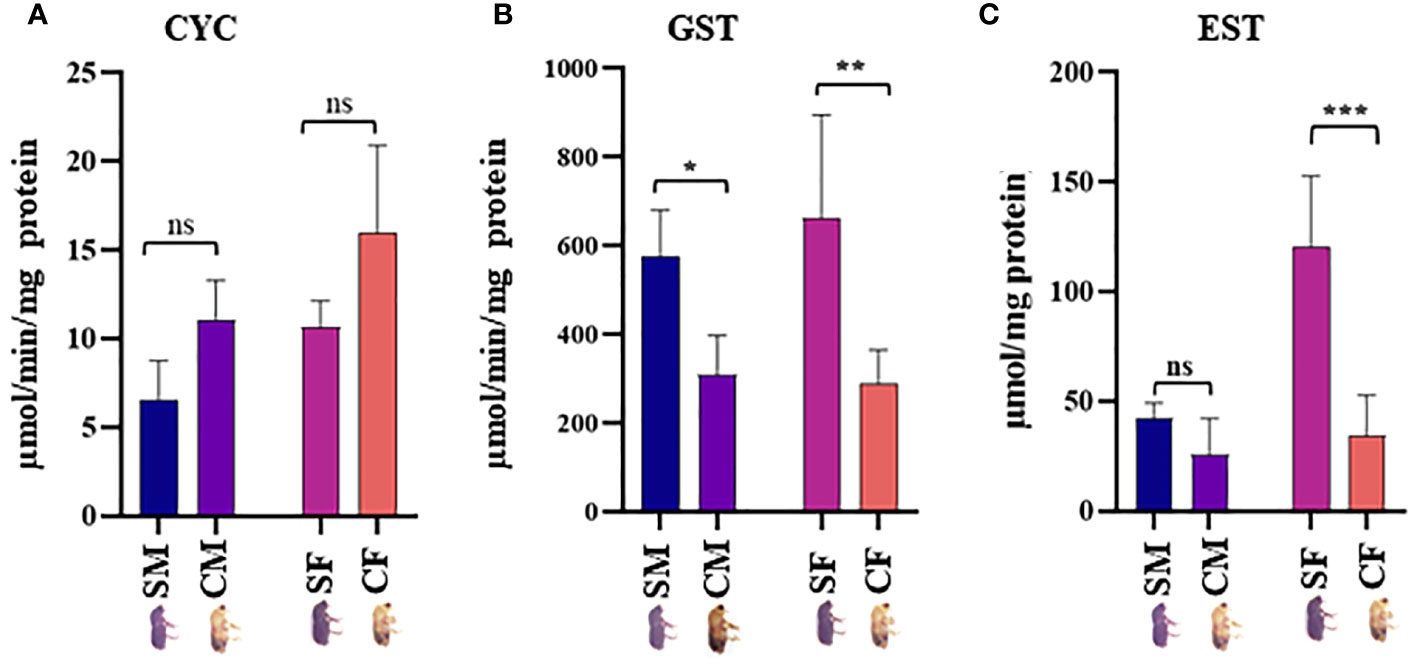
Figure 6 Enzymatic assay. (A) Expression levels of NADPH-cytochrome P450 reductase (CYC), (B) glutathione-S-transferase (GST), and (C) Esterases (EST) in males and females gut tissues of callow and sclerotized beetles (Mean± SE; P < 0.05). CM, callow male; CF, callow female; SM, sclerotized male; SF, sclerotized female. * denotes a significant difference between values from each other at * p < 0.05, ** p < 0.01, *** p < 0.001, and "ns" denotes non-significant.
Gene ontology enrichment analysis
The protein sequences were searched against the NCBI, InterPro database using Blast2GO, and the resulting biological process, cellular components, and molecular function of the proteins were examined (Figure 7; Supplementary Figure 2). Most proteins have at least one GO term annotated (Supplementary Figure 2). The differentially abundant proteins were separated into two categories to determine which GO term was more prevalent in males or females of both callow and sclerotized I. typographus: 1) CF vs. SF and 2) CM vs. SM. The comparison proteins between CF and SF were primarily distributed in the following processes: binding, ion binding, catalytic activity, cellular anatomical entity, intracellular anatomical structure, organelle, intracellular organelle, hydrolase activity, metabolic process, primary metabolic process, organic substance metabolic process, and carbohydrate metabolic process (Figure 7; Supplementary Figure 2). Besides, the comparison proteins between CM and SM had similar results. However, compared to CF vs. SF, the percentage of each GO term in CM vs. SM was lower.
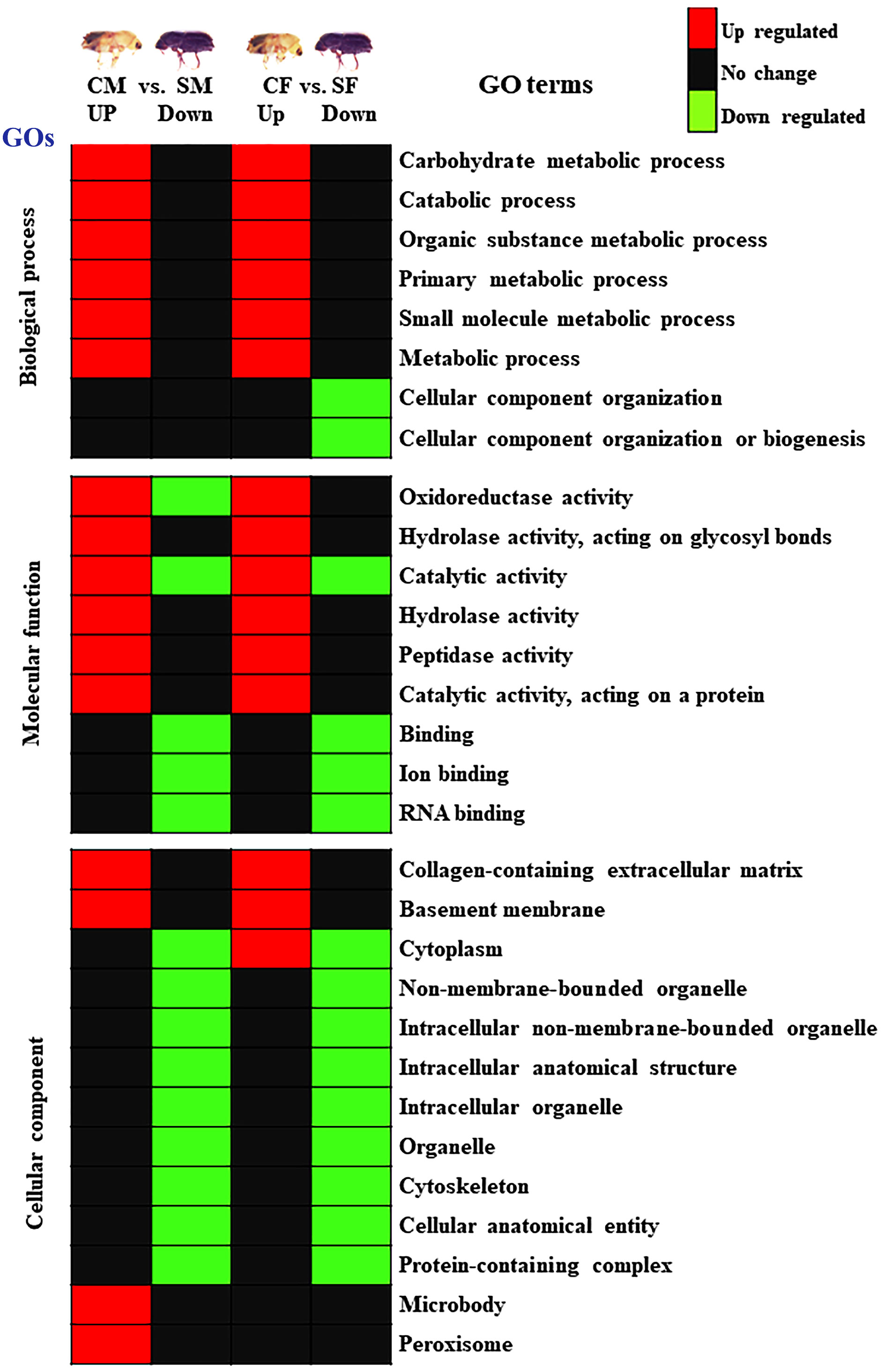
Figure 7 Summary of GO enrichment analysis. Red colour indicates upregulation (>2.0 fold), green colour indicates downregulation (<-2.0 fold), and black indicates no change. CM, callow male; CF, callow female; SM, sclerotized male; SF, sclerotized female.
DAPs related to metabolisms, detoxification, defence, digestion, cellular signalling, and growth
Based on protein expression and analysis, we identified and divided DAP-associated metabolisms into detoxification, digestion, signalling, and growth in the male and female guts of callow and sclerotized beetles (Figures 8, 9). The detoxification and defence-related proteins like venom carboxylesterase-6-like, short/branched chain specific acyl-CoA dehydrogenase, 15-hydroxyprostaglandin dehydrogenase [NAD(+)]-like, ipsdienol dehydrogenase, farnesol dehydrogenase-like are up-regulated and inosine-5’-monophosphate dehydrogenase, trans-1,2-dihydrobenzene-1,2-diol dehydrogenase, alcohol dehydrogenase [NADP(+)]-like, glucose dehydrogenase [acceptor], D-arabinitol dehydrogenase 1-like, sarcosine dehydrogenase, fatty aldehyde dehydrogenase-like, proline dehydrogenase 1, succinate dehydrogenase [ubiquinone] iron-sulfur subunit were down-regulated, whereas glutathione S-transferase-like was differentially regulated in callow compared to sclerotized beetles (Figure 8A). The digestion-related proteins like lysosomal alpha-mannosidase, lysosomal alpha-glucosidase-like, carboxypeptidase Q-like, zinc carboxypeptidase-like, aminopeptidase N-like, trypsin precursor, serine protease inhibitor 27A-like, Xaa-Pro aminopeptidase 1, trypsin-1-like, antichymotrypsin-2-like isoform XII, esterase B1-like, esterase E4-like, pancreatic triacylglycerol lipase-like, and antitrypsin-like isoform X4 were up-regulated. Glucosidase 2 subunit beta, lipase 3-like, inter-alpha-trypsin inhibitor heavy chain H4-like isoform X2, and acyl-coenzyme A thioesterase 13-like were down-regulated in callow compared to sclerotized beetles (Figure 8B). The signalling-related proteins like prostatic acid phosphatase, serine/threonine-protein phosphatase 2A catalytic subunit beta isoform, uridine diphosphate glucose pyrophosphatase-like, glycerol-3-phosphate phosphatase, venom acid phosphatase Acph-1-like, and trehalose-phosphatase phosphatase 4 were up-regulated. In contrast, integrin-linked protein kinase, alpha, alpha-trehalose-phosphate synthase (UDP-forming), ATP-dependent 6-phosphofructokinase isoform X5, and mitogen-activated protein kinase 1 were down-regulated in callow compared to sclerotized beetles (Figure 9A). The defence-related proteins like NADPH: adrenodoxin oxidoreductase and acidic mammalian chitinase-like were up-regulated, whereas NADH-ubiquinone oxidoreductase 75 kDa subunit is down-regulated in callow compared to sclerotized beetles (Figure 9B). The growth-related proteins like juvenile hormone esterase, protein krasavietz, and xylulose kinase were up-regulated, whereas calmodulin is down-regulated in callow compared to sclerotized beetles (Figure 9C).
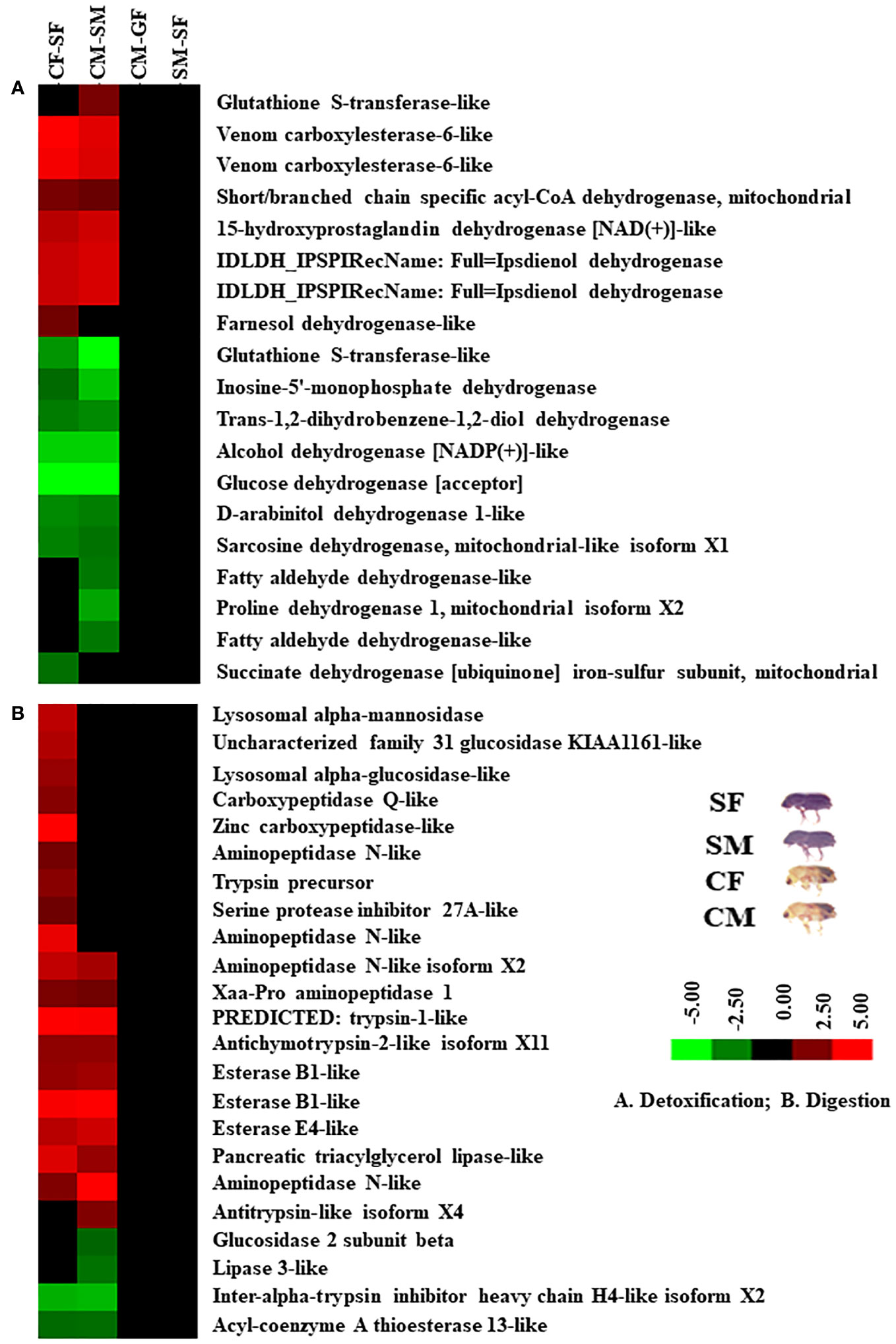
Figure 8 Cluster analysis of DAPs among gut samples of males and females of callow and sclerotized beetles. (A) Proteins related to detoxification; (B) Proteins related to digestion. Red colour indicates upregulation (>2.0 fold), green colour indicates downregulation (<-2.0 fold), and black indicates no change as compared to respective controls. CM, callow male; CF, callow female; SM, sclerotized male; SF, sclerotized female.
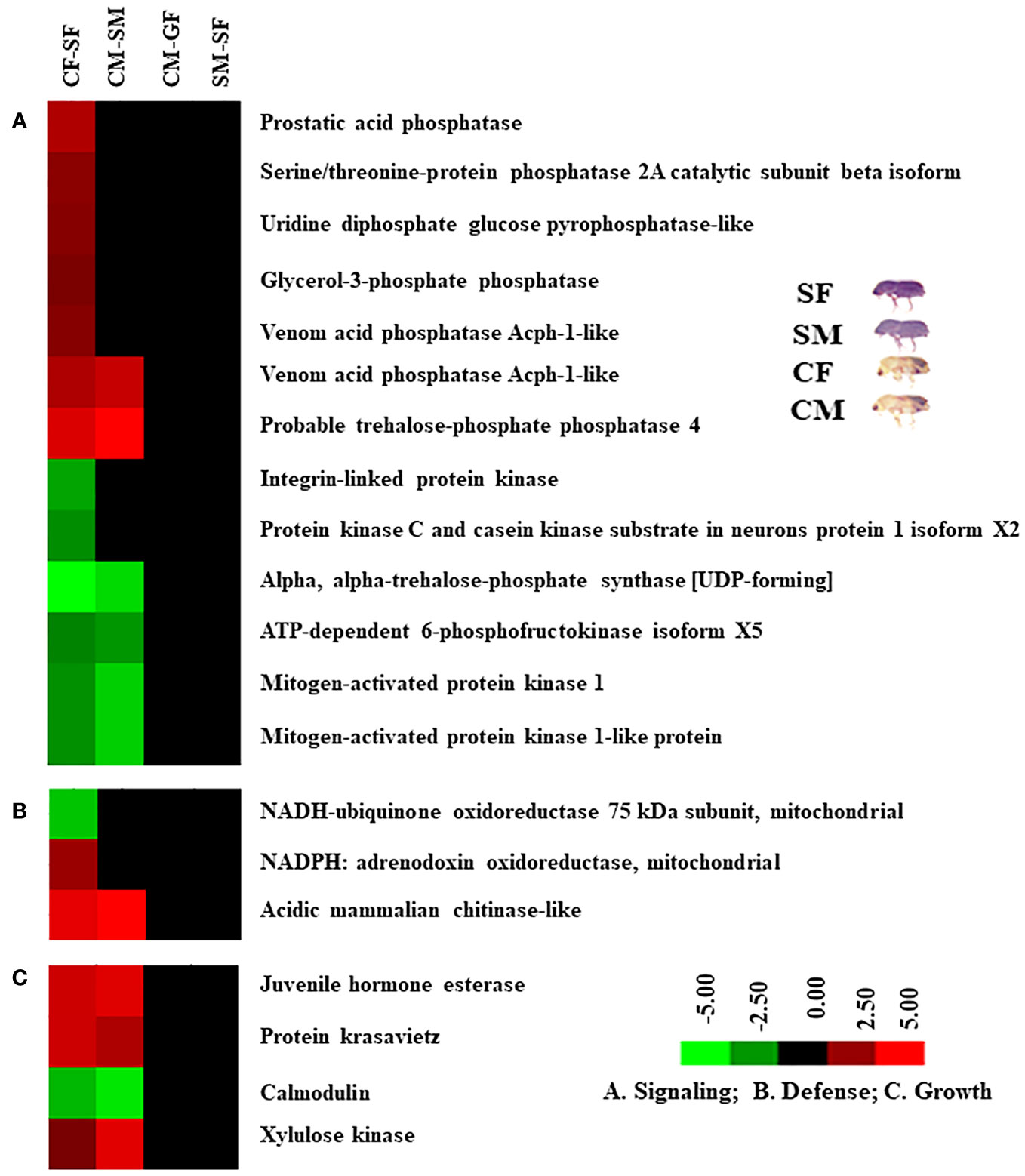
Figure 9 Cluster analysis of DAPs among gut samples of males and females of callow and sclerotized beetles. (A) Proteins related to signalling; (B) Proteins related to defence; (C) Proteins related to growth. Red colour indicates upregulation (>2.0 fold), green colour indicates downregulation (<-2.0 fold), and black indicates no change as compared to respective controls. CM, callow male; CF, callow female; SM, sclerotized male; SF, sclerotized female; DAPs, differentially abundant proteins.
The upregulated protein-coding enzymes in callow beetles are mainly related to JH biosynthesis (farnesol dehydrogenase-like, venom carboxylesterase-6-like, juvenile hormone esterase, and esterase E4-like), amino acid (ornithine aminotransferase, proline dehydrogenase 1, prolyl 4-hydroxylase subunit alpha-1-like isoform X1, carboxypeptidase Q-like, and aminopeptidase N-like), and pyridoxine metabolism (pyridoxal kinase and pyridoxine-5’-phosphate oxidase) (Figure 10). The overexpression of these proteins may aid in converting callow to sclerotized beetles. The upregulated proteins in sclerotized beetles are mostly connected to carbohydrates (glucosidase 2 subunit beta, glucose 1,6-bisphosphate synthase, uridine diphosphate glucose pyrophosphatase-like, alpha,alpha-trehalose-phosphate synthase [UDP-forming], probable trehalose-phosphate phosphatase 4, ATP-dependent 6-phosphofructokinase isoform X5, pyruvate carboxylase, and succinate dehydrogenase [ubiquinone] iron-sulfur subunit), fatty acid (acetyl-CoA carboxylase, acyl-CoA synthetase family member 3, mitochondrial-like fatty acyl-CoA reductase wat-like isoform X2, fatty aldehyde dehydrogenase-like, fatty acyl-CoA reductase wat-like isoform X2, alcohol dehydrogenase [NADP(+)]-like, short/branched chain specific acyl-CoA dehydrogenase, glycerol-3-phosphate phosphatase, and pancreatic triacylglycerol lipase-like), chitin (acidic mammalian chitinase-like), trypsin (inter-alpha-trypsin inhibitor heavy chain H4-like isoform X2), and glutathione metabolisms (glutathione synthetase-like isoform X2, probable phospholipid hydroperoxide glutathione peroxidase isoform X3, glutathione S-transferase) (Figure 10).
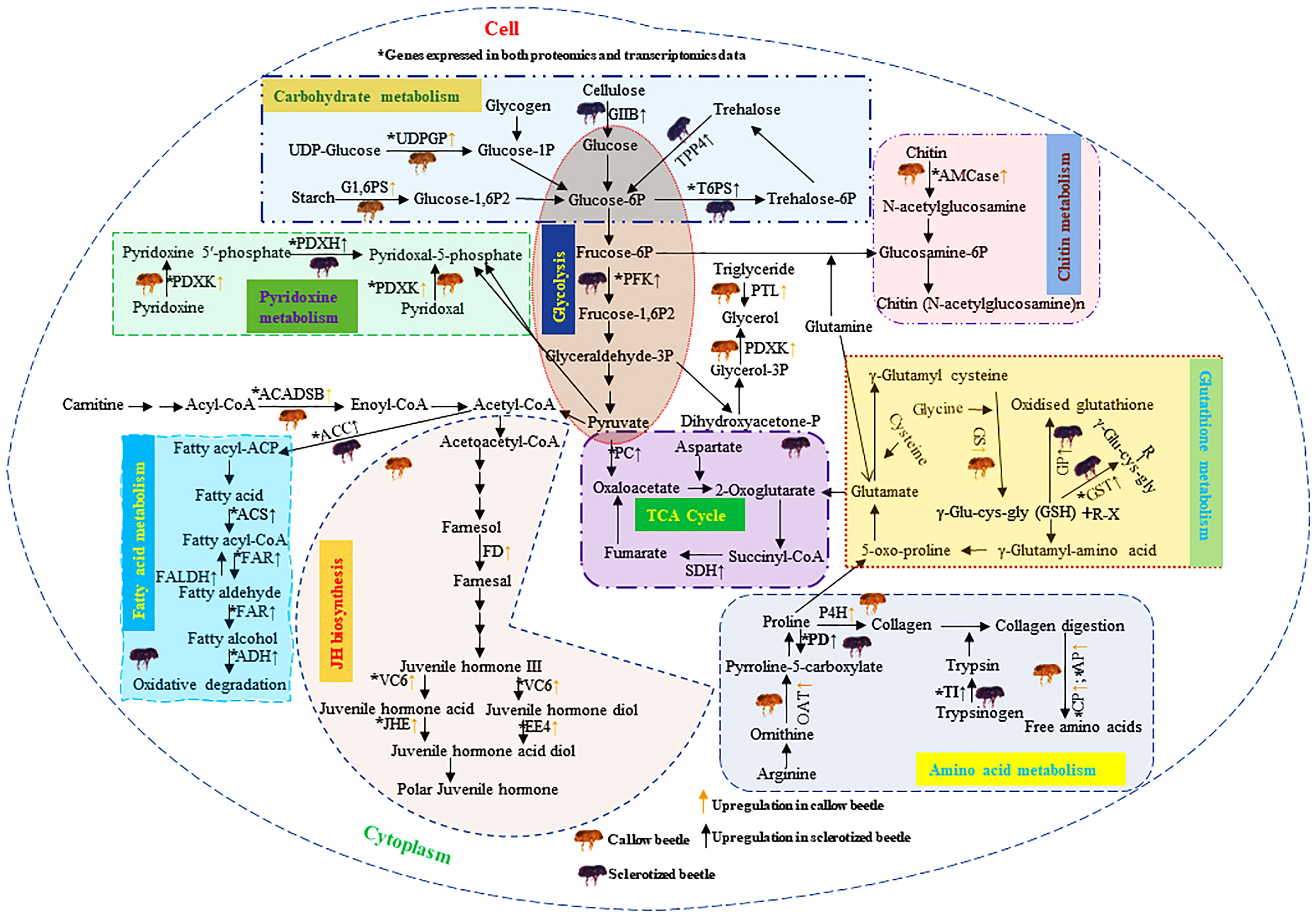
Figure 10 Diagrammatic representation of DAPs analysis in the callow and sclerotized males and females compared to respective controls. The yellow (↑) and black (↑) arrows represent the upregulated genes (FDR corrected p-value<0.05) in callow and sclerotized beetles, respectively. The upregulated protein-coding enzymes in callow beetles are mainly related to JH biosynthesis, gluconeogenesis, amino acid, and pyridoxine metabolism. The overexpression of these proteins may aid in converting callow to sclerotized beetles. The upregulated proteins in sclerotized beetles are mostly connected to carbohydrates, fatty acid, chitin, trypsin, and glutathione metabolisms. It is possible that sclerotized beetles are the pioneer for starting a new colony and may aid energy production and detoxification of host allelochemicals. Carbohydrate metabolism: Glucosidase 2 subunit beta (GIIB), glucose 1,6-bisphosphate synthase (G1,6PS), uridine diphosphate glucose pyrophosphatase-like (UDPGP), alpha,alpha-trehalose-phosphate synthase [UDP-forming] (T6PS), probable trehalose-phosphate phosphatase 4 (TPP4), ATP-dependent 6-phosphofructokinase isoform X5 (PFK), pyruvate carboxylase, mitochondrial (PC), and succinate dehydrogenase [ubiquinone] iron-sulfur subunit, mitochondrial (SDH). Fatty acid metabolism: Acetyl-CoA carboxylase (ACC), acyl-CoA synthetase family member 3 (ACS), mitochondrial-like fatty acyl-CoA reductase wat-like isoform X2 (FAR), Fatty aldehyde dehydrogenase-like (FALDH), fatty acyl-CoA reductase wat-like isoform X2 (FAR), Alcohol dehydrogenase [NADP(+)]-like (ADH), Short/branched chain specific acyl-CoA dehydrogenase, mitochondrial (ACADSB), glycerol-3-phosphate phosphatase (G3PP), and pancreatic triacylglycerol lipase-like (PTL). JH biosynthesis: Farnesol dehydrogenase-like (FD), Venom carboxylesterase-6-like (VC6), Juvenile hormone esterase (JHE), and esterase E4-like (EE4). Glutathione metabolism: Glutathione synthetase-like isoform X2 (GS), probable phospholipid hydroperoxide glutathione peroxidase isoform X3 (GP), Glutathione S-transferase (GST). Amino acid metabolism: Ornithine aminotransferase, mitochondrial (OAT), proline dehydrogenase 1, mitochondrial isoform X2 (PD), prolyl 4-hydroxylase subunit alpha-1-like isoform X1 (P4H), inter-alpha-trypsin inhibitor heavy chain H4-like isoform X2 (TI), carboxypeptidase Q-like (CP), and aminopeptidase N-like (AP). Chitin metabolism: Acidic mammalian chitinase-like (AMCase). Pyridoxin metabolism: Pyridoxal kinase (PDXK) and Pyridoxine-5’-phosphate oxidase (PDXH), Glutathione (GSH), Xenobiotics (R-X). DAPs: differentially abundant proteins.
Discussion
We find minimal differences in sex-specific protein expression between the two adult beetle stages. Instead, the protein expression difference between callow and sclerotized beetle gut tissues is much more noticeable, supporting their different ecological roles and life-stage-specific requirements. The differential protein expression between callow and sclerotized beetles may be a sign of different amounts of the feeding of host tissues. Callow beetles feed rigorously to attain the mature stage, hence investing more resources in the digestion and detoxification of food to prepare themselves for new host finding. Alternatively, sclerotized beetles stored enough energy for flight and host finding for colonization.
Functional prediction of DAPs: reflecting key physiological processes in beetle adult stages
Ecdysteroid and sesquiterpenoid pathways regulate embryogenesis, developmental change, and growth in insects (Adhitama et al., 2020). In this study, GO terms related to the juvenile hormone catabolic process, juvenile hormone metabolic process, sesquiterpenoid catabolic process, and sesquiterpenoid metabolic process under biological processes were upregulated in the callow males and females (Supplementary Figure 2), suggesting that the proteins may help callow beetles converting to become fully mature sclerotized beetles. Genetic, molecular, and cytological investigations on Drosophila have provided information on the precise roles played by the cytoskeleton during oogenesis (Cooley and Theurkauf, 1994). In this study, the biological process GO terms related to cellular component organization and cellular component biogenesis were upregulated in sclerotized females, suggesting that these proteins may have a role in cytoskeleton development and reproduction (Figure 7).
The RNA helicases Hlc, hel, eIF-4a, vasa, and mle have all been discovered in Drosophila melanogaster. These helicases appear to be engaged in various cellular functions, including the development of the embryonic body plan, the association of salivary gland chromosomes and embryonic cell nuclei, and male viability (Lasko and Ashburner, 1988; Kuroda et al., 1991; Dorn et al., 1993; de Couet et al., 1995; Eberl et al., 1997). RNA interference (RNAi)-mediated suppression of the Na+/K+-ATPase gene in the migrating locust results in patency losses, inhibiting Vg absorption, egg maturation and hindering ovarian development (Jing et al., 2018). The molecular function GO terms related to ATP-dependent activity, cytoskeletal protein binding, helicase activity, and pyrophosphatase activity were downregulated in callow females and upregulated in sclerotized females (Supplementary Figure 2), suggesting that they may help sclerotized female beetles for patency formation, Vg absorption, egg maturation, and ovarian development.
Collagen is ubiquitous throughout the animal kingdom, comprising some 28 diverse molecules forming the extracellular matrix within organisms (Sutherland et al., 2013; Halliwell and Gutteridge, 2015). In this study, the cellular component GO terms related to collagen-containing extracellular matrix and basement membrane proteins are upregulated (Figure 7) in male and female callow beetles, suggesting they may be involved in beetle metamorphosis.
DAPs contributed to beetle metabolisms, detoxification, and defence
Plants produce various allelochemicals to defend themselves against herbivory (Johnson, 2011; Saxena et al., 2023; Yadav et al., 2023). The resin in conifers is the essential component of chemical defence against the attacks of bark beetles and can inhibit beetle invasion, slow the invasion speed, and even repel the beetles (Erbilgin et al., 2003; Erbilgin et al., 2007). Moreover, the monoterpene in the resin is entomotoxic and causes debility or death through the respiratory system (Smith, 1965; Fernanda López et al., 2011). Our results are consistent with previous studies comparing transcriptional responses between generalist and specialist species (phytophagous insects) and with notable variations in the expression of genes associated with either direct or indirect xenobiotic detoxification between the two behaviours (Ali and Agrawal, 2012; Roy et al., 2016; Schweizer et al., 2017). The gut transcriptome of two Dendroctonus bark beetle species (D. rhizophagus and D. valens) stimulated by the same kairomones reveals molecular variations in detoxification pathways, such as up-regulated unigenes in D. rhizophagus (P450s and some COEs) that were different from those in D. valens (COEs, GSTs, and ABC transporters) (Torres-Banda et al., 2022). The glutathione S-transferases (GSTs) are detoxification enzymes that conjugate toxins with glutathione and play a role in excretion. To better understand the interaction between monoterpenes and beetles, sixteen full-length sequences were identified from four GST categories (delta, epsilon, sigma, and theta) and compared at four developmental stages (larvae, pupae, teneral adults, and adults), three different tissues (antennae, gut, and reproductive organs), and under various levels of terpenoid stimuli during feeding in D. armandi (Gao et al., 2020). While the numbers of cytosolic GSTs were similar among the beetle species D. valens, D. rhizophagus, D. ponderosae, L. decemlineata, and A. glabripennis but observed noteworthy differences in the Sigma, Delta, and Epsilon classes (Torres-Banda et al., 2022). The Zeta GSTs have been linked to the breakdown of tyrosine and phenylalanine following the completion of the cuticle sclerotization process, as well as being connected to the detoxification of xenobiotics containing chloride compounds (BOARD et al., 1997; Yamamoto et al., 2009). The existence of Omega GSTs in Dendroctonus species, Apis cerana, and Rhopalosiphum padi implies that these GSTs could have a significant function in helping these species adapt to their toxic-laden environment (Zhang et al., 2013; Dai et al., 2016; Balakrishnan et al., 2018). Furthermore, the presence of Sigma GSTs in Dendroctonus species points to a potential role for these proteins in oxidative stress defence through conjugation activity with lipid peroxidation products and carbaryl insecticides, which may have enabled these bark beetles to evolve survival strategies in toxin-rich subcortical environments (Fernanda López et al., 2011; Krokene, 2015; Gao et al., 2020; Dai et al., 2021). As bark beetles thrive on conifer host tissues in all life stages except egg and pupae, the Delta, Epsilon, and unclassified classes of GSTs are probably associated with beetle resistance to host secondary metabolites and terpenes (Liu et al., 2021). In the current study, the glutathione S-transferase genes are differentially regulated between callow and sclerotized males (Figures 6, 8A). Sclerotized beetles are attacking and drilling the bark for colony establishment; hence, they may require more GSTs to deal with the initial spruce defence response. However, the major spruce monoterpenes lack the electrophilic sites to react directly with glutathione. Hence, we speculate that the fungal symbionts of bark beetle species metabolize spruce resin monoterpenes and alter the profile of spruce bark volatiles by converting the major monoterpenes into an attractive blend of oxygenated derivatives, which might act as the GST substrate (Kandasamy et al., 2023). Alternatively, the monoterpenes stimulate ROS production, causing the production of oxidized metabolites, such as hydroperoxides, which could also be GST substrates. Furthermore, toxic piperidine alkaloids of spruce can also be the substrate for GST due to the presence of electrophilic sites (Tawara et al., 1993; Stermitz et al., 2000). However, such possibilities demand further experimental corroboration.
Members of the carboxylesterases (COEs) clades A and C are found in the dietary and detoxification functions class, which is broadly represented in all insects, whereas clade A is unique and diverse in the phylogeny of bark beetles and is primarily involved in the metabolism of xenobiotics (Lü et al., 2015; Sood et al., 2016). The up-regulation of COEs in D. valens, D. armandi, and D. ponderosae following exposure to the kairomone blend and their phloem feeding demonstrates the essential role of these COEs in terpene metabolism (Robert et al., 2013; Dai et al., 2019; Dai et al., 2021). A recent study identified the potential insecticide resistance marker in the venom carboxylesterase-6-like gene through genomic-level comparison of the current field and 1976 year collected Bemisia tabaci (Gennadius) lines, suggests that venom carboxylesterase-6-like gene play a role in the insecticide resistance mechanism (Wang et al., 2022). In Aphidius gifuensis, the antennae- and sex-specific transcriptome study, phylogenic analysis, and tissue-expression patterns identified venom carboxylesterase-6-like as a critical ODE involved in the chemical breakdown (Kang et al., 2021). In the present study, compared to the sclerotized beetles, the venom carboxylesterase-6-like gene shows higher expression (3.57 to 5.5 folds) in both males and females of callow beetles (Figure 8A), suggesting its putative involvement in the detoxification of plant chemicals during maturation feeding.
Alcohol dehydrogenases (ADHs) are required for xenobiotics detoxification, especially for the oxidation of permethrin (Choi et al., 2002). In S. zeamais, the upregulation of ADHs shows improved critical substrate conservation in the carbohydrate metabolism, consequently increasing the xenobiotics metabolism (Huang et al., 2019). Prior research has demonstrated the presence of ethanol in both xylem and phloem tissues, with its concentration increasing in plant tissues during bark beetle attacks (Kelsey et al., 2014; Lehenberger et al., 2021). High concentrations of ethanol are hazardous to bark beetles, and alcohol dehydrogenase is responsible for metabolizing the majority of the ethanol absorbed as part of the diet, and its action may help to quickly eliminate the ethanol from the bark beetle hemolymph (Dettner, 2023). As ethanol is also toxic to pathogens or fungal antagonists of bark beetle nutritional fungal mutualists, thriving on the eatnol-rich host substrate using ADH may give them a selective survival advantage (Lehenberger et al., 2021). Compared to the callow I. typographus, the alcohol dehydrogenase [NADP(+)]-like gene has higher expression (4 fold up) in both male and female sclerotized beetles (Figure 8A), implying its requirement while establishing a new colony and making the galleries for oviposition. The dietary alkaloid-fed honeybee proteomic and metabolomic analysis showed upregulation of succinate dehydrogenase and glucose dehydrogenase, rate-limiting enzymes in the TCA cycle, and pentose phosphate pathway, signifying that they may be involved in NADH and NADPH production required for the P450 functioning and cellular redox state maintenance (Esther et al., 2017). In I. typographus, glucose dehydrogenase [acceptor] (-7 fold) and succinate dehydrogenase [ubiquinone] (-2.22 fold) were downregulated in callow males compared to the sclerotized males (Figure 8A), implying that they may require continuous energy production during new host finding. In male I. pini beetles, the ipsdienol dehydrogenase (IDOLDH) is an essential enzyme in pheromone biosynthesis that oxidizes explicitly (−)-ipsdienol and (−)-ipsenol to ipsdienone and ipsenone, respectively, and is thought to “tune” ipsdienol enantiomeric ratios (Figueroa-Teran et al., 2012; Figueroa-Teran et al., 2016). Ipsdienol dehydrogenase (IDLDH) is upregulated (3.87 to 4.19 folds) in the male and female callow beetles compared to sclerotized ones (Figure 8A), suggesting that host feeding (i.e., including alpha-pinene) induces pheromone production for future chemical communication during host colonization in I. typographus.
Insect life, moulting, and development depend on at least some chitinases belonging to various families (Arakane and Muthukrishnan, 2010). Also, bacterial associates of bark beetles may be involved in the production of metabolites such as chitinases to serve as a protective barrier against pathogens, as well as boosting the metabolic capacity to fulfil its nutritional requirements through the hydrolysis of complex sugar polymers that are typically indigestible into simpler, more readily assimilated sugars (García‐Fraile, 2018; Chakraborty et al., 2020; Chakraborty et al., 2023; Peral-Aranega et al., 2023). In this present study, the acidic mammalian chitinase-like gene was upregulated (4.16 to 5.36 folds) in males and females of callow beetles compared to sclerotized beetles (Figure 9B), referring to its necessity during the transition to sclerotized beetles. In Drosophila melanogaster, adrenodoxin reductase (ADXR) is encoded by the nuclear gene dare and plays a crucial role in ecdysteroid production when mutant larvae are fed the insect steroid hormone 20-hydroxyecdysone, null mutants of the dare gene experience developmental arrest. The NADPH: adrenodoxin oxidoreductase (mitochondrial) gene was found to be significantly expressed (2.54 fold) in the callow females in the current study (Figure 9B), indicating that it may aid in the storage of ecdysteroids and their use in the conversion of callow females to sclerotized females in addition to their putative role in the detoxification mechanism (Freeman et al., 1999). Further functional studies may delineate the functional role of such crucial enzymes in the bark beetle life cycle.
DAPs involved in beetle digestion
Insect digestive proteins are mainly produced and secreted into the lumen after being directed by midgut epithelial cells, and through the lumen, food particles pass (Hegedus et al., 2009; Napoleão et al., 2019). In lepidopteran insects, aminopeptidase N (APN) isoforms play a role in the mode of action of Bacillus thuringiensis insecticidal Cry proteins (Crava et al., 2013). The Aminopeptidase-N is also found in the midgut of coleopterans and is a protein that binds to Cry3Aa toxins (Guo et al., 2020). In this study, aminopeptidases (aminopeptidase N-like, aminopeptidase N-like isoform X2, and xaa-Pro aminopeptidase 1) are significantly upregulated (2.22 to 5.35 folds) in male and female callow beetles compared to the sclerotized beetles (Figure 8B), suggesting its involvement in digestion of plant materials during the rigorous maturation feeding. Trypsin-like enzymes are involved in moulting, tissue remodelling, innate immunity, diapause, fertilization, female postmating behaviour regulation, and activation of enzyme precursors of trypsin, chymotrypsin, chitinase, phenoloxidase, and Bt-toxin degradation (Friedländer et al., 2001; Royer et al., 2002; Wagner et al., 2002; Kanost et al., 2004; Chen et al., 2005; Peng et al., 2005; Wei et al., 2007; Liu et al., 2009; Parde et al., 2012). In the current study, antichymotrypsin-2-like isoform X11 and trypsin-1-like genes are upregulated (2.78 to 5.01 folds) in both male and female callow beetles (Figure 8B), implying their involvement in the spruce tissue digestion. Trypsin precursor and antitrypsin-like isoform X4 were upregulated (2.67 and 2.52 folds) in callow females and males, suggesting they may contribute to development, reproductive regulations, and tissue remodelling (Figure 8B).
An RNAi study in Nilaparvata lugens recently showed that injected dsRNA against inter-alpha-trypsin inhibitor heavy chain 4 (ITIH4) reduced survival, egg production, egg hatching, and delayed ovarian development. These results indicate that ITIH4 plays a vital role in development and reproduction (Ji et al., 2022). In I. typographus, inter-alpha-trypsin inhibitor heavy chain H4-like isoform X2 was downregulated in both male (-3.56 fold) and female (-3.43 fold) callow beetles compared to the sclerotized ones, suggesting that it may involve in the development and reproduction in sclerotized beetles (Figure 8B). In Culex pipiens quinquefasciatus, high expression of the esterase B1 gene shows insecticide resistance (Mouches et al., 1990). Esterase B1-like shows high expression (2.90 to 5.51 folds) in both males and females of callow beetles compared to the sclerotized beetles, indicating their involvement in coping with allelochemicals during active host feeding (Figure 8B). The pancreatic triglyceride lipase (PTL) gene knockdown brown planthopper (Nilaparvata lugens), insects fed to insect-resistant rice varieties showed less food intake, lipid content, survival rate, and fecundity (Yuan et al., 2020). The pancreatic triacylglycerol lipase-like gene is upregulated in both male (2.93 fold) and female (4.28 fold) callow beetles to aid the digestion of food materials and reproductive development (Figure 8B). Carboxypeptidase Q may play an essential role in the hydrolysis of the circulating peptides and catalyzes unsuitable terminals into amino acids (Gatehouse, 2013). The zinc carboxypeptidase belongs to the B type and prepared COOH-terminal residue. Zinc carboxypeptidase expression rapidly increased in teneral flies after 1-hour post-bloodmeal feeding compared to unfed (Yan et al., 2002). In I. typographus, carboxypeptidase Q-like, and zinc carboxypeptidase-like genes are upregulated (2.64 and 5.35 folds) in female callow beetles compared to sclerotized female beetles, suggesting their putative requirement for initiating the ovary development for egg formation during the callow to sclerotized beetle transition (Figure 8B). However, such possibilities need functional endorsements.
In Drosophila, serine protease inhibitor 27A regulates the melanization cascade by explicitly inhibiting the terminal protease prophenoloxidase activating enzyme. Serine protease inhibitor 27A-like gene was upregulated (2.19 fold) in female callow compared to the sclerotized female beetles, endorsing their similar role in melanization in beetles (Figure 8B). The xylulose kinase phosphorylates the D-xylulose to produce D-xylulose-5-phosphate and is essential in regulating glucose metabolism and lipogenesis (Bunker et al., 2013). In the present study, the xylulose kinase is upregulated (4.06 and 2.01 folds) in both callow males and females to support the digestion of plant carbohydrates during maturation feeding (Figure 9C).
DAPs involved in beetle cellular signalling
RNAi studies in the Cabbage Beetle (Colaphellus bowringi) revealed that the MAPK signalling pathway is essential for insect female reproductive regulation (Huang et al., 2022). Mitogen-activated protein kinase 1 (MAPK 1), also known as an extracellular signal-regulated kinase 2 (ERK2) or MAPK p38, is involved in insect defence against Bt Cry toxins (Cancino-Rodezno et al., 2010). In this study, the mitogen-activated protein kinase 1 and mitogen-activated protein kinase 1-like protein expression is higher in sclerotized male and female beetles than the callow beetles, plausibly aiding in mitigating challenges by pathogens during establishing a new colony (Figure 9A). The trehalose-phosphate phosphatase 4 is involved in the pathway trehalose biosynthesis, which removes the phosphate from trehalose 6-phosphate to produce free trehalose. The alpha, alpha-trehalose-phosphate synthase [UDP-forming], also known as trehalose phosphate-UDP glucosyltransferase, is a catalytic subunit of the trehalose synthase complex that catalyzes the production of trehalose from glucose-6-phosphate and UDP-alpha-D-glucose in a two-step process. In I. typographus, alpha-trehalose-phosphate synthase [UDP-forming] and ATP-dependent 6-phosphofructokinase isoform X5 were upregulated (3.87 to 4.97 folds, and 2.48 and 2.16 folds) in male and female sclerotized beetles (Figure 9A). These results suggest that sclerotized beetles need energy for flying, especially sclerotized male beetles, which are the pioneers in establishing the colony, and these genes are mainly involved in trehalose metabolism and provide energy for flying.
DAPs involved in beetle growth
Juvenile hormone (JH) is a factor produced by the corpora allata glands that prevent immature insects from moulting into adults, and rates of its biosynthesis and degradation regulate the JH (Kamita et al., 2003). In lepidopteran larvae, inhibition of Juvenile hormone esterases (JHE) reduces the rate of JH degradation, leading to abnormally large larvae and delayed pupation (Hammock et al., 1990). In I. pini, an analysis of expressed sequence tags (ESTs) revealed the upregulation of seven mevalonate genes in midgut tissues, indicating their putative responsiveness to JHIII (Eigenheer et al., 2003). In the present study, the juvenile hormone esterase is upregulated (4.0 and 3.57 folds) in male and female callow beetles compared to the sclerotize group, suggesting their putative involvement in the metamorphic process of callow beetles (Figure 9C). Calmodulin (CaM) is one of the most evolutionarily conserved Ca2+ sensors in organisms and plays a role in the stress response of insects to the diamide insecticide cyantraniliprole (Guo et al., 2021). In S. frugiperda, calmodulin plays a role in the midgut microapocrine secretory process of digestive enzymes (Ferreira et al., 2007; Silva et al., 2013). Calmodulin was upregulated (2.88 and 3.53 folds) in the male and female callow beetles compared to the sclerotized group, plausibly to induce the secretion of digestive enzymes for conifer food digestion during maturation feeding (Figure 9C).
DAPs and DETs correlation: unveiling conserved responses
Through our investigation between gut proteome and transcriptome of both callow and sclerotized beetles, we identified numerous DAPs specific to each adult stage, and these findings illuminate the multifaceted roles of these proteins and how they contribute to the life histories, ecological strategies, and adaptive physiological responses of I. typographus in effectively mitigating the toxicity of host allelochemicals. A subset of transcripts and proteins were expressed with the same pattern (Figure 2). Most shared proteins are engaged in conserved processes such as gut development, detoxification, digestion, defence, signalling, and growth, respectively. A similar correlation trend was found in the study of the heat stress-accumulated transcriptome and proteome in Neoseiulus barkeri (Tian et al., 2020). The ecological relevance of such DAPs or DETs with conserved expression can be an exciting research avenue for better understanding the beetle adaptive physiology and may provide some valuable target genes for management purposes.
Conclusion
This is the first study investigating the regulatory mechanisms of digestion, detoxification, and growth in callow and sclerotized beetles from the I. typographus gut protein expression perspective. DAP analysis, DAP and DET comparison, enzymatic assays, and RT-qPCR validation results indicated that the I. typographs gut protein expression pattern played a significant role in digestion, detoxification, and growth in callow or sclerotized stages (Figure 10). The current study sheds light on how the fine-tuning of protein expression in the gut is based on the physiological requirements of the beetle adult stages (Figure 10). This aspect is not considered before in other bark beetles. In this study, we found that proteins related to JH biosynthesis and chitin metabolism are upregulated in callow beetles, whereas proteins related to fatty acid metabolism and the TCA cycle are upregulated in sclerotized beetles. These differential protein regulation patterns may aid I. typographus in fulfilling their life-stage-specific ecological duties (Figure 10) and create new avenues for future functional studies. Nevertheless, current data will facilitate further protein-level studies in this beetle or other coleopteran forest pests, creating the possibility of formulating superior forest pest management strategies targeting essential proteins using RNAi with superior delivery methods (i.e., nanoparticle or microbe-mediated delivery) applicable to forestry (Joga et al., 2021; Gupta et al., 2023; Mogilicherla and Roy, 2023; Sandal et al., 2023).
Data availability statement
The mass spectrometry proteomics data have been deposited to the ProteomeXchange Consortium via the PRIDE [1] partner repository with the dataset identifier PXD035407.
Ethics statement
The manuscript presents research on animals that do not require ethical approval for their study.
Author contributions
AR conceptualization. MA, GS sample collection, experimental work. VS, FL proteomics data generation and processing. KM, MA, GS biological interpretation, figure preparation, and first draft wiring. AR and KM prepared the final draft. All authors read and approved the final version of the draft.
Funding
AR, KM financed by EVA 4.0,” No. CZ.02.1.01/0.0/0.0/16 019/0000803 supported by OP RDE. GS, AR, and MA are supported by EXTEMIT – K,” No. CZ.02.1.01/0.0/0.0/15_003/0000433 financed by OP RDE. The project is also funded by “EXTEMIT – K,” No. CZ.02.1.01/0.0/0.0/15_003/0000433 financed by OP RDE. AR, KM, and GS are also supported by “Excellent Team Grants” 2023-2024 from FLD, CZU.
Acknowledgments
We acknowledge the support from the Faculty for Forestry and Wood Sciences (FLD), CZU, for conducting the present study. We also acknowledge Dr Roman Modlinger and Dr Jiri Synek, FLD, CZU, for beetle collection and general rearing assistance during the EXTEMIT-K project tenure. We appreciate the helpful feedback provided by the reviewers and handling editor. We also acknowledge Prof. Ladislav Kokoška, FTZ, CZU, for providing the instrument facility for enzymatic studies. Special thanks to Prof. Fredrik Schylter for his constructive comments and encouragement for proteomics work.
Conflict of interest
The authors declare that the research was conducted in the absence of any commercial or financial relationships that could be construed as a potential conflict of interest.
Publisher’s note
All claims expressed in this article are solely those of the authors and do not necessarily represent those of their affiliated organizations, or those of the publisher, the editors and the reviewers. Any product that may be evaluated in this article, or claim that may be made by its manufacturer, is not guaranteed or endorsed by the publisher.
Supplementary material
The Supplementary Material for this article can be found online at: https://www.frontiersin.org/articles/10.3389/fpls.2023.1157455/full#supplementary-material
Supplementary Figure 1 | Standardization of total protein isolation and quality checking. (A). Total protein was isolated from six different gut samples, run on the SDS-PAGE gel, and checked the protein quality. (B). After reviewing the protein quality and loading on stacking gel, run up to the separating gel, cut the protein band, and carry on the in-gel digestion process. M. Protein marker; 1 to 6: Biological replicates of gut protein. *Six biological replicates were utilized from each sample for total protein isolation, followed by four high-quality samples for protein sequencing.
Supplementary Figure 2 | Bar graphs showing gene ontology classification between four comparisons. Category and functional distribution of the 539 DAPs between four comparisons according to the significant classifications of gene ontology: Biological Process, Molecular Function, and Cellular Components. (A). GF vs SF Upregulated; (B). GF vs SF Downregulated; (C). GM vs SM Upregulated; (D). GM vs SM Downregulated. CM: callow male; CF: callow female; SM: sclerotized male; SF: sclerotized female; DAPs: differentially abundant proteins.
Supplementary Figure 3 | CYC (A), GST (B), and EST (C)- influence of enzyme source concentration (0.5, 1, 2, and 4 µg) and time course (30min) on reaction rates (ΔAbs (u)/min). (A) sclerotized male (SM), (B) sclerotized female (SF), (C) callow male (CM), and (D) callow female (CF). Error bars correspond to standard error (total number of guts tested (n = 48).
Supplementary Excel File 1 | Supporting information for differentially abundant proteins in various comparisons.
Supplementary Excel File 2 | Supporting information for gene expression at transcriptomics and proteomics level.
Supplementary Table 1 | Genes and their primer sequences used for RT-qPCR.
References
Adhitama, N., Kato, Y., Matsuura, T., Watanabe, H. (2020). Roles of and cross-talk between ecdysteroid and sesquiterpenoid pathways in embryogenesis of branchiopod crustacean Daphnia magna. PloS One 15 (10), e0239893. doi: 10.1371/journal.pone.0239893
Ali, J. G., Agrawal, A. A. (2012). Specialist versus generalist insect herbivores and plant defense. Trends Plant Sci. 17 (5), 293–302. doi: 10.1016/j.tplants.2012.02.006
Arakane, Y., Muthukrishnan, S. (2010). Insect chitinase and chitinase-like proteins. Cell. Mol. Life Sci. 67 (2), 201–216. doi: 10.1007/s00018-009-0161-9
Aranda, G. P., Hinojos, S. J., Sabandal, P. R., Evans, P. D., Han, K.-A. (2017). Behavioral sensitization to the disinhibition effect of ethanol requires the dopamine/ecdysone receptor in Drosophila. Front. Syst. Neurosci. 11, 56. doi: 10.3389/fnsys.2017.00056
Balakrishnan, B., Su, S., Wang, K., Tian, R., Chen, M. (2018). Identification, expression, and regulation of an omega class glutathione S-transferase in Rhopalosiphum padi (L.)(Hemiptera: Aphididae) under insecticide stress. Front. Physiol. 9, 427. doi: 10.3389/fphys.2018.00427
Bengtsson, T., Weighill, D., Proux-Wéra, E., Levander, F., Resjö, S., Burra, D. D., et al. (2014). Proteomics and transcriptomics of the BABA-induced resistance response in potato using a novel functional annotation approach. BMC Genomics 15 (1), 1–19. doi: 10.1186/1471-2164-15-315
Berrada, S., Fournier, D., Cuany, A., Nguyen, T. (1994). Identification of resistance mechanisms in a selected laboratory strain of Cacopsylla pyri (Homoptera: Psyllidae): altered acetylcholinesterase and detoxifying oxidases. Pesticide Biochem. Physiol. 48 (1), 41–47. doi: 10.1006/pest.1994.1005
Board, G. P., Baker, T. R., Chelvanayagam, G., Jermiin, S. L. (1997). Zeta, a novel class of glutathione transferases in a range of species from plants to humans. Biochem. J. 328 (3), 929–935. doi: 10.1042/bj3280929
Bosch-Serra, D., Rodríguez, M. A., Avilla, J., Sarasúa, M. J., Miarnau, X. (2021). Esterase, glutathione S-transferase and NADPH-cytochrome P450 reductase activity evaluation in cacopsylla pyri L.(Hemiptera: psyllidae) individual adults. Insects 12 (4), 329. doi: 10.3390/insects12040329
Bradford, M. M. (1976). A rapid and sensitive method for the quantitation of microgram quantities of protein utilizing the principle of protein-dye binding. Analytical Biochem. 72 (1-2), 248–254. doi: 10.1016/0003-2697(76)90527-3
Bunker, R. D., Bulloch, E. M., Dickson, J. M., Loomes, K. M., Baker, E. N. (2013). Structure and function of human xylulokinase, an enzyme with important roles in carbohydrate metabolism. J. Biol. Chem. 288 (3), 1643–1652. doi: 10.1074/jbc.M112.427997
Cancino-Rodezno, A., Alexander, C., Villaseñor, R., Pacheco, S., Porta, H., Pauchet, Y., et al. (2010). The mitogen-activated protein kinase p38 is involved in insect defense against Cry toxins from Bacillus thuringiensis. Insect Biochem. Mol. Biol. 40 (1), 58–63. doi: 10.1016/j.ibmb.2009.12.010
Chakraborty, A., Ashraf, M. Z., Modlinger, R., Synek, J., Schlyter, F., Roy, A. (2020). Unravelling the gut bacteriome of Ips (Coleoptera: Curculionidae: Scolytinae): identifying core bacterial assemblage and their ecological relevance. Sci. Rep. 10 (1), 18572. doi: 10.1038/s41598-020-75203-5
Chakraborty, A., Purohit, A., Khara, A., Modlinger, R., Roy, A. (2023). Life-stage and geographic location determine the microbial assemblage in Eurasian spruce bark beetle, Ips typographus L.(Coleoptera: Curculionidae). Front. Forests Global Change 6, 1176160. doi: 10.3389/ffgc.2023.1176160
Chen, B., Kayukawa, T., Jiang, H., Monteiro, A., Hoshizaki, S., Ishikawa, Y. (2005). DaTrypsin, a novel clip-domain serine proteinase gene up-regulated during winter and summer diapauses of the onion maggot, Delia antiqua. Gene 347 (1), 115–123. doi: 10.1016/j.gene.2004.12.026
Choi, J., Rose, R. L., Hodgson, E. (2002). In vitro human metabolism of permethrin: the role of human alcohol and aldehyde dehydrogenases. Pesticide Biochem. Physiol. 74 (3), 117–128. doi: 10.1016/S0048-3575(02)00154-2
Christiansen, E., Bakke, A. (1988). “"The spruce bark beetle of Eurasia,",” in Dynamics of forest insect populations (Boston, MA: Springer US), 479–503. doi: 10.1007/978-1-4899-0789-9_23
Conesa, A., Götz, S., García-Gómez, J. M., Terol, J., Talón, M., Robles, M. (2005). Blast2GO: a universal tool for annotation, visualization and analysis in functional genomics research. Bioinformatics 21 (18), 3674–3676. doi: 10.1093/bioinformatics/bti610
Cooley, L., Theurkauf, W. E. (1994). Cytoskeletal functions during Drosophila oogenesis. Science 266 (5185), 590–596. doi: 10.1126/science.7939713
Crava, C. M., Bel, Y., Jakubowska, A. K., Ferré, J., Escriche, B. (2013). Midgut aminopeptidase N isoforms from Ostrinia nubilalis: activity characterization and differential binding to Cry1Ab and Cry1Fa proteins from Bacillus thuringiensis. Insect Biochem. Mol. Biol. 43 (10), 924–935. doi: 10.1016/j.ibmb.2013.07.009
Dai, L., Gao, H., Chen, H. (2021). Expression levels of detoxification enzyme genes from Dendroctonus armandi (Coleoptera: Curculionidae) fed on a solid diet containing pine phloem and terpenoids. Insects 12 (10), 926. doi: 10.3390/insects12100926
Dai, L., Gao, H., Ye, J., Fu, D., Sun, Y., Chen, H. (2019). Isolation of CarE genes from the Chinese white pine beetle Dendroctonus armandi (Curculionidae: Scolytinae) and their response to host chemical defense. Pest Manage. Sci. 75 (4), 986–997. doi: 10.1002/ps.5205
Dai, L., Ma, J., Ma, M., Zhang, H., Shi, Q., Zhang, R., et al. (2016). Characterisation of GST genes from the Chinese white pine beetle Dendroctonus armandi (Curculionidae: Scolytinae) and their response to host chemical defence. Pest Manage. Sci. 72 (4), 816–827. doi: 10.1002/ps.4059
de Couet, H. G., Fong, K., Weeds, A., McLaughlin, P., Miklos, G. (1995). Molecular and mutational analysis of a gelsolin-family member encoded by the flightless I gene of Drosophila melanogaster. Genetics 141 (3), 1049–1059. doi: 10.1093/genetics/141.3.1049
Dettner, K. (2023). “Chemical ecology and biochemistry of Dytiscidae,” in Ecology, systematics, and the natural history of predaceous diving beetles (Coleoptera: Dytiscidae) (Cham: Springer), 253–341. doi: 10.1007/978-3-031-01245-7_6
Dorn, R., Morawietz, H., Reuters, G., Saumweber, H. (1993). Identification of an essential Drosophila gene that is homologous to the translation initiation factor eIF-4A of yeast and mouse. Mol. Gen. Genet. MGG 237 (1), 233–240. doi: 10.1007/BF00282805
Eberl, D. F., Lorenz, L. J., Melnick, M. B., Sood, V., Lasko, P., Perrimon, N. (1997). A new enhancer of position-effect variegation in Drosophila melanogaster encodes a putative RNA helicase that binds chromosomes and is regulated by the cell cycle. Genetics 146 (3), 951–963. doi: 10.1093/genetics/146.3.951
Eigenheer, A. L., Keeling, C. I., Young, S., Tittiger, C. (2003). Comparison of gene representation in midguts from two phytophagous insects, Bombyx mori and Ips pini, using expressed sequence tags. Gene 316, 127–136. doi: 10.1016/S0378-1119(03)00749-2
Eisen, M. B., Spellman, P. T., Brown, P. O., Botstein, D. (1998). Cluster analysis and display of genome-wide expression patterns. Proc. Natl. Acad. Sci. 95 (25), 14863–14868. doi: 10.1073/pnas.95.25.14863
Erbilgin, N., Krokene, P., Kvamme, T., Christiansen, E. (2007). A host monoterpene influences Ips typographus (Coleoptera: Curculionidae, Scolytinae) responses to its aggregation pheromone. Agric. For. Entomology 9 (2), 135–140. doi: 10.1111/j.1461-9563.2007.00329.x
Erbilgin, N., Powell, J. S., Raffa, K. F. (2003). Effect of varying monoterpene concentrations on the response of Ips pini (Coleoptera: Scolytidae) to its aggregation pheromone: implications for pest management and ecology of bark beetles. Agric. For. Entomology 5 (4), 269–274. doi: 10.1046/j.1461-9563.2003.00186.x
Esther, E., Human, H., Smit, S., Beukes, M., Apostolides, Z., Nicolson, S. W., et al. (2017). Proteomic and metabolomic analysis reveals rapid and extensive nicotine detoxification ability in honey bee larvae. Insect Biochem. Mol. Biol. 82, 41–51. doi: 10.1016/j.ibmb.2017.01.011
Fernanda López, M., Cano-Ramírez, C., Shibayama, M., Zúñiga, G. (2011). α-Pinene and myrcene induce ultrastructural changes in the midgut of Dendroctonus valens (Coleoptera: Curculionidae: Scolytinae). Ann. Entomological Soc. America 104 (3), 553–561. doi: 10.1603/AN10023
Ferreira, A. H. P., Cristofoletti, P. T., Lorenzini, D. M., Guerra, L. O., Paiva, P. B., Briones, M. R. D. S., et al. (2007). Identification of midgut microvillar proteins from Tenebrio molitor and Spodoptera frugiperda by cDNA library screenings with antibodies. J. Insect Physiol. 53 (11), 1112–1124. doi: 10.1016/j.jinsphys.2007.06.007
Figueroa-Teran, R., Pak, H., Blomquist, G. J., Tittiger, C. (2016). High substrate specificity of ipsdienol dehydrogenase (IDOLDH), a short-chain dehydrogenase from Ips pini bark beetles. J. Biochem. 160 (3), 141–151. doi: 10.1093/jb/mvw019
Figueroa-Teran, R., Welch, W. H., Blomquist, G. J., Tittiger, C. (2012). Ipsdienol dehydrogenase (IDOLDH): a novel oxidoreductase important for Ips pini pheromone production. Insect Biochem. Mol. Biol. 42 (2), 81–90. doi: 10.1016/j.ibmb.2011.10.009
Freeman, M. R., Dobritsa, A., Gaines, P., Segraves, W. A., Carlson, J. R. (1999). The dare gene: steroid hormone production, olfactory behavior, and neural degeneration in Drosophila. Development 126 (20), 4591–4602. doi: 10.1242/dev.126.20.4591
Friedländer, M., Jeshtadi, A., Reynolds, S. E. (2001). The structural mechanism of trypsin-induced intrinsic motility in Manduca sexta spermatozoa in vitro. J. Insect Physiol. 47 (3), 245–255. doi: 10.1016/S0022-1910(00)00109-8
Gao, H., Dai, L., Fu, D., Sun, Y., Chen, H. (2020). Isolation, Expression Profiling, and Regulation via Host Allelochemicals of 16 Glutathione S-Transferases in the Chinese White Pine Beetle, Dendroctonus armandi. Front. Physiol. 11, 546592. doi: 10.3389/fphys.2020.546592
García‐Fraile, P. (2018). Roles of bacteria in the bark beetle holobiont–how do they shape this forest pest? Ann. Appl. Biol. 172 (2), 111–125. doi: 10.1111/aab.12406
Gatehouse, J. A. (2013). Insect Carboxypeptidases. In Rawlings, N., Salvesen, G. (Eds.), Handbook of proteolytic enzymes (1366-1370). 3rd ed. (Elsevier), 1366–1370. doi: 10.1016/b978-0-12-382219-2.00306-9
Grégoire, J.-C., Raffa, K. F., Lindgren, B. S. (2015). “ Economics and politics of bark beetles,” in Vega, F. E., Hofstetter, R. W. (Eds.), Bark beetles: Biology and ecology of native and invasive species (Amsterdam, The Netherlands: Elsevier), pp. 585–613. doi: 10.1016/B978-0-12-417156-5.00015-0
Guo, Y., Carballar-Lejarazú, R., Sheng, L., Fang, Y., Wang, S., Liang, G., et al. (2020). Identification and characterization of aminopeptidase-N as a binding protein for Cry3Aa in the midgut of Monochamus alternatus (Coleoptera: Cerambycidae). J. Economic Entomology 113 (5), 2259–2268. doi: 10.1093/jee/toaa130
Guo, L., Li, C., Coupland, G., Liang, P., Chu, D. (2021). Up-regulation of calmodulin involved in the stress response to cyantraniliprole in the whitefly, Bemisia tabaci (Hemiptera: Aleyrodidae). Insect Sci. 28 (6), 1745–1755. doi: 10.1111/1744-7917.12887
Gupta, S., Chakraborty, A., Roy, A. (2023). Prospects for deploying microbes against tree-killing beetles (Coleoptera) in Anthropocene. Front. Forests Global Change 6, 1182834. doi: 10.3389/ffgc.2023.1182834
Habig, W. H., Jakoby, W. B. (1981). Assays for differentiation of glutathione S-transferase. Methods Enzymol 77, 398–405. doi: 10.1016/S0076-6879(81)77053-8
Halliwell, B., Gutteridge, J.M. (2015). Free Radicals in Biology and Medicine. Oxford University Press, Oxford.
Hammock, B. D., Bonning, B. C., Possee, R. D., Hanzlik, T. N., Maeda, S. (1990). Expression and effects of the juvenile hormone esterase in a baculovirus vector. Nature 344 (6265), 458–461. doi: 10.1038/344458a0
Hegedus, D., Erlandson, M., Gillott, C., Toprak, U. (2009). New insights into peritrophic matrix synthesis, architecture, and function. Annu. Rev. entomology 54 (1), 285–302. doi: 10.1146/annurev.ento.54.110807.090559
Hlásny, T., König, L., Krokene, P., Lindner, M., Montagné-Huck, C., Müller, J., et al. (2021a). Bark beetle outbreaks in Europe: State of knowledge and ways forward for management. Curr. Forestry Rep. 7 (3), 138–165. doi: 10.1007/s40725-021-00142-x
Hlásny, T., Krokene, P., Liebhold, A., Montagné-Huck, C., Müller, J., Qin, H., et al. (2019). Living with bark beetles: impacts, outlook and management options. From Science to Policy 8. European Forest Institute.
Hlásny, T., Zimová, S., Merganičová, K., Štěpánek, P., Modlinger, R., Turčáni, M. (2021b). Devastating outbreak of bark beetles in the Czech Republic: Drivers, impacts, and management implications. For. Ecol. Manage. 490, 119075. doi: 10.1016/j.foreco.2021.119075
Huang, Y., Liao, M., Yang, Q., Xiao, J., Hu, Z., Cao, H. (2019). iTRAQ-based quantitative proteome revealed metabolic changes of Sitophilus zeamais in response to terpinen-4-ol fumigation. Pest Manage. Sci. 75 (2), 444–451. doi: 10.1002/ps.5135
Huang, Z., Tian, Z., Zhao, Y., Zhu, F., Liu, W., Wang, X. (2022). MAPK signaling pathway is essential for female reproductive regulation in the cabbage beetle, colaphellus bowringi. Cells 11 (10), 1602. doi: 10.3390/cells11101602
Huber, L. A. (2003). Is proteomics heading in the wrong direction? Nat. Rev. Mol. Cell Biol. 4 (1), 74–80. doi: 10.1038/nrm1007
Huber, D., Robert, J. (2016). The proteomics and transcriptomics of early host colonization and overwintering physiology in the mountain pine beetle, Dendroctonus ponderosae Hopkins (Coleoptera: Curculionidae). Adv. Insect Physiol. 50, 101–128.
Jandl, R. (2020). Climate-induced challenges of Norway spruce in Northern Austria. Trees forests People 1, 100008. doi: 10.1016/j.tfp.2020.100008
Ji, J.-L., Han, S.-J., Zhang, R.-J., Yu, J.-B., Li, Y.-B., Yu, X.-P., et al. (2022). Inter-alpha-trypsin inhibitor heavy chain 4 plays an important role in the development and reproduction of nilaparvata lugens. Insects 13 (3), 303. doi: 10.3390/insects13030303
Jing, Y.-P., An, H., Zhang, S., Wang, N., Zhou, S. (2018). Protein kinase C mediates juvenile hormone–dependent phosphorylation of Na+/K+-ATPase to induce ovarian follicular patency for yolk protein uptake. J. Biol. Chem. 293 (52), 20112–20122. doi: 10.1074/jbc.RA118.005692
Joga, M. R., Mogilicherla, K., Smagghe, G., Roy, A. (2021). RNA interference-based forest protection products (FPPs) against wood-boring coleopterans: Hope or hype? Front. Plant Sci. 1943. doi: 10.3389/fpls.2021.733608
Johnson, M. T. (2011). Evolutionary ecology of plant defences against herbivores. Funct. Ecol. 25, 305–311.
Kamita, S. G., Hinton, A. C., Wheelock, C. E., Wogulis, M. D., Wilson, D. K., Wolf, N. M., et al. (2003). Juvenile hormone (JH) esterase: why are you so JH specific? Insect Biochem. Mol. Biol. 33 (12), 1261–1273. doi: 10.1016/j.ibmb.2003.08.004
Kandasamy, D., Zaman, R., Nakamura, Y., Zhao, T., Hartmann, H., Andersson, M. N., et al. (2023). Conifer-killing bark beetles locate fungal symbionts by detecting volatile fungal metabolites of host tree resin monoterpenes. PloS Biol. 21 (2), e3001887. doi: 10.1371/journal.pbio.3001887
Kang, Z. W., Liu, F. H., Xu, Y. Y., Cheng, J. H., Lin, X. L., Jing, X. F., et al. (2021). Identification of candidate odorant-degrading enzyme genes in the antennal transcriptome of Aphidius gifuensis. Entomological Res. 51 (1), 36–54. doi: 10.1111/1748-5967.12489
Kanost, M. R., Jiang, H., Yu, X. Q. (2004). Innate immune responses of a lepidopteran insect, Manduca sexta. Immunol. Rev. 198 (1), 97–105. doi: 10.1111/j.0105-2896.2004.0121.x
Keeling, C. I., Li, M., Sandhu, H. K., Henderson, H., Saint Yuen, M. M., Bohlmann, J. (2016). Quantitative metabolome, proteome and transcriptome analysis of midgut and fat body tissues in the mountain pine beetle, Dendroctonus ponderosae Hopkins, and insights into pheromone biosynthesis. Insect Biochem. Mol. Biol. 70, 170–183. doi: 10.1016/j.ibmb.2016.01.002
Kelsey, R. G., Gallego, D., Sánchez-García, F., Pajares, J. (2014). Ethanol accumulation during severe drought may signal tree vulnerability to detection and attack by bark beetles. Can. J. For. Res. 44 (6), 554–561. doi: 10.1139/cjfr-2013-0428
Krokene, P. (2015). “Conifer defense and resistance to bark beetles,” in Bark beetles (Elsevier), 177–207.
Kuroda, M. I., Kernan, M. J., Kreber, R., Ganetzky, B., Baker, B. S. (1991). The maleless protein associates with the X chromosome to regulate dosage compensation in Drosophila. Cell 66 (5), 935–947. doi: 10.1016/0092-8674(91)90439-6
Lasko, P. F., Ashburner, M. (1988). The product of the Drosophila gene vasa is very similar to eukaryotic initiation factor-4A. Nature 335 (6191), 611–617. doi: 10.1038/335611a0
Lehenberger, M., Benkert, M., Biedermann, P. H. (2021). Ethanol-enriched substrate facilitates ambrosia beetle fungi, but inhibits their pathogens and fungal symbionts of bark beetles. Front. Microbiol. 11, 3487. doi: 10.3389/fmicb.2020.590111
Lehninger, A. (1950). Role of metal ions in enzyme systems. Physiol. Rev. 30 (3), 393–429. doi: 10.1152/physrev.1950.30.3.393
Li, G., Xu, L., Zhang, H., Liu, J., Yan, J., Yan, Y. (2020). A de novo designed esterase with p-nitrophenyl acetate hydrolysis activity. Molecules 25 (20), 4658. doi: 10.3390/molecules25204658
Liu, Y., Moural, T., Koirala BK, S., Hernandez, J., Shen, Z., Alyokhin, A., et al. (2021). Structural and functional characterization of one unclassified glutathione S-transferase in xenobiotic adaptation of Leptinotarsa decemlineata. Int. J. Mol. Sci. 22 (21), 11921. doi: 10.3390/ijms222111921
Liu, Y., Sui, Y. P., Wang, J. X., Zhao, X. F. (2009). Characterization of the trypsin-like protease (Ha-TLP2) constitutively expressed in the integument of the cotton bollworm, Helicoverpa armigera. Arch. Insect Biochem. Physiology: Published Collaboration Entomological Soc. America 72 (2), 74–87. doi: 10.1002/arch.20324
Livak, K. J., Schmittgen, T. D. (2001). Analysis of relative gene expression data using real-time quantitative PCR and the 2– ΔΔCT method. methods 25 (4), 402–408. doi: 10.1006/meth.2001.1262
Lü, F.-G., Fu, K.-Y., Li, Q., Guo, W.-C., Ahmat, T., Li, G.-Q. (2015). Identification of carboxylesterase genes and their expression profiles in the Colorado potato beetle Leptinotarsa decemlineata treated with fipronil and cyhalothrin. Pesticide Biochem. Physiol. 122, 86–95. doi: 10.1016/j.pestbp.2014.12.015
Margoliash, E., Frohwirt, N. (1959). Spectrum of horse-heart cytochrome c. Biochem. J. 71 (3), 570. doi: 10.1042/bj0710570
Mogilicherla, K., Roy, A. (2023). RNAi-chitosan biopesticides for managing forest insect pests: an outlook. Front. Forests Global Change 6, 1219685. doi: 10.3389/ffgc.2023.1219685
Montagné-Huck, C., Brunette, M. (2018). Economic analysis of natural forest disturbances: A century of research. J. For. Economics 32, 42–71. doi: 10.1016/j.jfe.2018.03.002
Morris, J. L., Cottrell, S., Fettig, C. J., Hansen, W. D., Sherriff, R. L., Carter, V. A., et al. (2017). Managing bark beetle impacts on ecosystems and society: priority questions to motivate future research. J. Appl. Ecol. 54 (3), 750–760. doi: 10.1111/1365-2664.12782
Mouches, C., Pauplin, Y., Agarwal, M., Lemieux, L., Herzog, M., Abadon, M., et al. (1990). Characterization of amplification core and esterase B1 gene responsible for insecticide resistance in Culex. Proc. Natl. Acad. Sci. 87 (7), 2574–2578. doi: 10.1073/pnas.87.7.2574
Napoleão, T. H., Albuquerque, L. P., Santos, N. D., Nova, I. C., Lima, T. A., Paiva, P. M., et al. (2019). Insect midgut structures and molecules as targets of plant-derived protease inhibitors and lectins. Pest Manage. Sci. 75 (5), 1212–1222. doi: 10.1002/ps.5233
Naseer, A., Mogilicherla, K., Sellamuthu, G., Roy, A. (2023). Age matters: Life-stage, tissue, and sex-specific gene expression dynamics in Ips typographus (Coleoptera: Curculionidae: Scolytinae). Front. Forests Global Change 6. doi: 10.3389/ffgc.2023.1124754
Ortego, F., López-Olguı́n, J., Ruız, M., Castañera, P. (1999). Effects of toxic and deterrent terpenoids on digestive protease and detoxication enzyme activities of Colorado potato beetle larvae. Pesticide Biochem. Physiol. 63 (2), 76–84. doi: 10.1006/pest.1998.2386
Parde, V. D., Sharma, H. C., Kachole, M. S. (2012). Inhibition of Helicoverpa armigera gut pro-proteinase activation in response to synthetic protease inhibitors. Entomologia experimentalis applicata 142 (2), 104–113. doi: 10.1111/j.1570-7458.2011.01209.x
Peng, J., Chen, S., Büsser, S., Liu, H., Honegger, T., Kubli, E. (2005). Gradual release of sperm bound sex-peptide controls female postmating behavior in Drosophila. Curr. Biol. 15 (3), 207–213. doi: 10.1016/j.cub.2005.01.034
Peral-Aranega, E., Saati-Santamaría, Z., Ayuso-Calles, M., Kostovčík, M., Veselská, T., Švec, K., et al. (2023). New insight into the bark beetle ips typographus bacteriome reveals unexplored diversity potentially beneficial to the host. Environ. microbiome 18 (1), 53. doi: 10.1186/s40793-023-00510-z
Pitt, C., Robert, J. A., Bonnett, T. R., Keeling, C. I., Bohlmann, J., Huber, D. P. (2014). Proteomics indicators of the rapidly shifting physiology from whole mountain pine beetle, Dendroctonus ponderosae (Coleoptera: Curculionidae), adults during early host colonization. PloS One 9 (10), e110673. doi: 10.1371/journal.pone.0110673
Powell, D., Groβe-Wilde, E., Krokene, P., Roy, A., Chakraborty, A., Löfstedt, C., et al. (2021). A highly-contiguous genome assembly of the Eurasian spruce bark beetle, Ips typographus, provides insight into a major forest pest. Commun. Biol. 4 (1), 1–9. doi: 10.1038/s42003-021-02602-3
Robert, J. A., Pitt, C., Bonnett, T. R., Yuen, M. M., Keeling, C. I., Bohlmann, J., et al. (2013). Disentangling detoxification: gene expression analysis of feeding mountain pine beetle illuminates molecular-level host chemical defense detoxification mechanisms. PloS One 8 (11), e77777. doi: 10.1371/journal.pone.0077777
Roy, A., Das, S. (2015). Molecular mechanism underlying the entomotoxic effect of Colocasia esculenta tuber agglutinin against Dysdercus cingulatus. Insects 6 (4), 827–846. doi: 10.3390/insects6040827
Roy, A., Gupta, S., Hess, D., Das, K. P., Das, S. (2014). Binding of insecticidal lectin C olocasia esculenta tuber agglutinin (CEA) to midgut receptors of B emisia tabaci and L ipaphis erysimi provides clues to its insecticidal potential. Proteomics 14 (13-14), 1646–1659. doi: 10.1002/pmic.201300408
Roy, A., Walker, W., III, Vogel, H., Chattington, S., Larsson, M., Anderson, P., et al. (2016). Diet dependent metabolic responses in three generalist insect herbivores Spodoptera spp. Insect Biochem. Mol. Biol. 71, 91–105. doi: 10.1016/j.ibmb.2016.02.006
Royer, V., Fraichard, S., Bouhin, H. (2002). A novel putative insect chitinase with multiple catalytic domains: hormonal regulation during metamorphosis. Biochem. J. 366 (3), 921–928. doi: 10.1042/bj20011764
Sandal, S., Singh, S., Bansal, G., Kaur, R., Mogilicherla, K., Pandher, S., et al. (2023). Nanoparticle-Shielded dsRNA Delivery for Enhancing RNAi Efficiency in Cotton Spotted Bollworm Earias vittella (Lepidoptera: Nolidae). Int. J. Mol. Sci. 24 (11), 9161. doi: 10.3390/ijms24119161
Saxena, H., Negi, H., Keshan, R., Chitkara, P., Kumar, S., Chakraborty, A., et al. (2023). A comprehensive investigation of lipid-transfer proteins from Cicer arietinum disentangles their role in plant defense against Helicoverpa armigera-infestation. Front. Genet. 14. doi: 10.3389/fgene.2023.1195554
Schlyter, F., Cederholm, I. (1981). Separation of the sexes of living spruce bark beetles, Ips typographus (L.),(Coleoptera: Scolytidae) 1. Z. für angewandte Entomologie 92 (1-5), 42–47. doi: 10.1111/j.1439-0418.1981.tb01650.x
Schuldt, B., Buras, A., Arend, M., Vitasse, Y., Beierkuhnlein, C., Damm, A., et al. (2020). A first assessment of the impact of the extreme 2018 summer drought on Central European forests. Basic Appl. Ecol. 45, 86–103. doi: 10.1016/j.baae.2020.04.003
Schweizer, F., Heidel-Fischer, H., Vogel, H., Reymond, P. (2017). Arabidopsis glucosinolates trigger a contrasting transcriptomic response in a generalist and a specialist herbivore. Insect Biochem. Mol. Biol. 85, 21–31. doi: 10.1016/j.ibmb.2017.04.004
Seidl, R., Schelhaas, M.-J., Rammer, W., Verkerk, P. J. (2014). Increasing forest disturbances in Europe and their impact on carbon storage. Nat. Climate Change 4 (9), 806–810. doi: 10.1038/nclimate2318
Sellamuthu, G., Bílý, J., Joga, M. R., Synek, J., Roy, A. (2022). Identifying optimal reference genes for gene expression studies in Eurasian spruce bark beetle, Ips typographus (Coleoptera: Curculionidae: Scolytinae). Sci. Rep. 12 (1), 1–17. doi: 10.1038/s41598-022-08434-3
Senf, C., Buras, A., Zang, C. S., Rammig, A., Seidl, R. (2020). Excess forest mortality is consistently linked to drought across Europe. Nat. Commun. 11 (1), 1–8. doi: 10.1038/s41467-020-19924-1
Senf, C., Seidl, R. (2021). Persistent impacts of the 2018 drought on forest disturbance regimes in Europe. Biogeosciences 18 (18), 5223–5230. doi: 10.5194/bg-18-5223-2021
Shevchenko, A., Tomas, H., Havli, J., Olsen, J. V., Mann, M. (2006). In-gel digestion for mass spectrometric characterization of proteins and proteomes. Nat. Protoc. 1 (6), 2856–2860. doi: 10.1038/nprot.2006.468
Silva, W., Cardoso, C., Ribeiro, A. F., Terra, W. R., Ferreira, C. (2013). Midgut proteins released by microapocrine secretion in Spodoptera frugiperda. J. Insect Physiol. 59 (1), 70–80. doi: 10.1016/j.jinsphys.2012.10.015
Smagghe, G., Tirry, L. (2001). “Insect midgut as a site for insecticide detoxification and resistance,” in Biochemical sites of insecticide action and resistance (Berlin, Heidelberg: Springer), 293–321. doi: 10.1007/978-3-642-59549-3_14
Smith, H. W. (1965). The development of the flora of the alimentary tract in young animals. J. Pathol. Bacteriology 90, 495–513. doi: 10.1002/path.1700900218
Sommerfeld, A., Rammer, W., Heurich, M., Hilmers, T., Müller, J., Seidl, R. (2021). Do bark beetle outbreaks amplify or dampen future bark beetle disturbances in Central Europe? J. Ecol. 109 (2), 737–749. doi: 10.1111/1365-2745.13502
Sood, S., Sharma, A., Sharma, N., Kanwar, S. (2016). Carboxylesterases: sources, characterization and broader applications. Insight Enzym Res. 1 (1), 1–11. doi: 10.21767/2573-4466.100002
Stermitz, F. R., Kamm, C. D., Tawara, J. N. (2000). Piperidine alkaloids of spruce (Picea) and fir (Abies) species. Biochem. Systematics Ecol. 28 (2), 177–181. doi: 10.1016/S0305-1978(99)00054-X
Sutherland, T. D., Peng, Y. Y., Trueman, H. E., Weisman, S., Okada, S., Walker, A. A., et al. (2013). A new class of animal collagen masquerading as an insect silk. Sci. Rep. 3 (1), 1–6. doi: 10.1038/srep02864
Tawara, J. N., Blokhin, A., Foderaro, T. A., Stermitz, F. R., Hope, H. (1993). Toxic piperidine alkaloids from pine (Pinus) and spruce (Picea) trees. New structures and a biosynthetic hypothesis. J. Organic Chem. 58 (18), 4813–4818. doi: 10.1021/jo00070a014
Tian, C. B., Li, Y. Y., Huang, J., Chu, W. Q., Wang, Z. Y., Liu, H. (2020). Comparative transcriptome and proteome analysis of heat acclimation in predatory mite Neoseiulus barkeri. Front. Physiol. 11, 426. doi: 10.3389/fphys.2020.00426
Torres-Banda, V., Obregón-Molina, G., Soto-Robles, L. V., Albores-Medina, A., López, M. F., Zúñiga, G. (2022). Gut transcriptome of two bark beetle species stimulated with the same kairomones reveals molecular differences in detoxification pathways. Comput. Struct. Biotechnol. J. 20, 3080–3095. doi: 10.1016/j.csbj.2022.06.029
Toth, D., Maitah, M., Maitah, K., Jarolínová, V. (2020). The impacts of calamity logging on the development of spruce wood prices in Czech forestry. Forests 11 (3), 283. doi: 10.3390/f11030283
Vindstad, O. P. L., Birkemoe, T., Ims, R. A., Sverdrup-Thygeson, A. (2020). Environmental conditions alter successional trajectories on an ephemeral resource: a field experiment with beetles in dead wood. Oecologia 194 (1), 205–219. doi: 10.1007/s00442-020-04750-5
Vindstad, O. P. L., Jepsen, J. U., Ek, M., Pepi, A., Ims, R. A. (2019). Can novel pest outbreaks drive ecosystem transitions in northern-boreal birch forest? J. Ecol. 107 (3), 1141–1153. doi: 10.1111/1365-2745.13093
Wagner, W., Möhrlen, F., Schnetter, W. (2002). Characterization of the proteolytic enzymes in the midgut of the European Cockchafer, Melolontha melolontha (Coleoptera: Scarabaeidae). Insect Biochem. Mol. Biol. 32 (7), 803–814. doi: 10.1016/S0965-1748(01)00167-9
Wang, H.-L., Rao, Q., Chen, Z.-Z. (2023). Identifying potential insecticide resistance markers through genomic‐level comparison of Bemisia tabaci (Gennadius) lines. Arch. Insect Biochem. Physiol. 114 (1), e22034. doi: 10.1002/arch.22034
Wei, Z., Yin, Y., Zhang, B., Wang, Z., Peng, G., Cao, Y., et al. (2007). Cloning of a novel protease required for the molting of Locusta migratoria manilensis. Development Growth Differentiation 49 (7), 611–621. doi: 10.1111/j.1440-169X.2007.00957.x
Willforss, J., Chawade, A., Levander, F. (2018). NormalyzerDE: online tool for improved normalization of omics expression data and high-sensitivity differential expression analysis. J. Proteome Res. 18 (2), 732–740. doi: 10.1021/acs.jproteome.8b00523
Yadav, M., Panwar, R., Rustagi, A., Chakraborty, A., Roy, A., Singh, I. K., et al. (2023). Comprehensive and evolutionary analysis of Spodoptera litura-inducible Cytochrome P450 monooxygenase gene family in Glycine max elucidate their role in defense. Front. Plant Sci. 14, 1221526. doi: 10.3389/fpls.2023.1221526
Yamamoto, K., Shigeoka, Y., Aso, Y., Banno, Y., Kimura, M., Nakashima, T. (2009). Molecular and biochemical characterization of a Zeta-class glutathione S-transferase of the silkmoth. Pesticide Biochem. Physiol. 94 (1), 30–35. doi: 10.1016/j.pestbp.2009.02.008
Yan, J., Cheng, Q., Li, C. B., Aksoy, S. (2002). Molecular characterization of three gut genes from Glossina morsitans morsitans: cathepsin B, zinc-metalloprotease and zinc-carboxypeptidase. Insect Mol. Biol. 11 (1), 57–65. doi: 10.1046/j.0962-1075.2001.00308.x
Yuan, L.-y., Hao, Y.-h., Chen, Q.-k., Pang, R., Zhang, W.-q. (2020). Pancreatic triglyceride lipase is involved in the virulence of the brown planthopper to rice plants. J. Integr. Agric. 19 (11), 2758–2766. doi: 10.1016/S2095-3119(20)63188-4
Keywords: conifer pests, Scolytinae, Ips typographus (L.), comparative proteomics, DAPs, digestion, detoxification, enzyme assay
Citation: Ashraf MZ, Mogilicherla K, Sellamuthu G, Siino V, Levander F and Roy A (2023) Comparative gut proteomics study revealing adaptive physiology of Eurasian spruce bark beetle, Ips typographus (Coleoptera: Scolytinae). Front. Plant Sci. 14:1157455. doi: 10.3389/fpls.2023.1157455
Received: 02 February 2023; Accepted: 01 November 2023;
Published: 21 November 2023.
Edited by:
Manuel Martinez, Polytechnic University of Madrid, SpainReviewed by:
Shiv Bharadwaj, Institute of Biotechnology of the Czech Academy of Sciences, CzechiaJonathan Gershenzon, Max Planck Institute for Chemical Ecology, Germany
Copyright © 2023 Ashraf, Mogilicherla, Sellamuthu, Siino, Levander and Roy. This is an open-access article distributed under the terms of the Creative Commons Attribution License (CC BY). The use, distribution or reproduction in other forums is permitted, provided the original author(s) and the copyright owner(s) are credited and that the original publication in this journal is cited, in accordance with accepted academic practice. No use, distribution or reproduction is permitted which does not comply with these terms.
*Correspondence: Amit Roy, roy@fld.czu.cz
†These authors have contributed equally to this work