. Introduction
Grazing is one of the most significant human disturbances affecting grassland ecosystems. It directly or indirectly affects grassland vegetation and soil through livestock activities such as trampling, ingestion, and excretion (Lemaire et al., 2000). Research has shown that long-term overgrazing is the primary cause of grassland degradation. Overgrazing not only reduces vegetation coverage and primary productivity, but also reduces biodiversity (Schönbach et al., 2011; White et al., 2000). Grazing has a positive effect (when moderately grazing) or a general effect on species richness (Fensham et al., 2014; Kimuyu et al., 2014; Socher et al., 2013). Some scholars have suggested that intermittent grazing or grazing prohibition can mitigate the adverse effects of grazing on biodiversity and ecosystem functions (Lunt et al., 2007; Williams et al., 2006; Williamson et al., 2014). Global sustainability and human prosperity depend on biodiversity and ecosystem products and services (e.g., primary productivity, nutrient cycling, and soil formation) (J. Wu, 2013). Therefore, it is crucial to discuss the influence of grazing activity on ecosystem functions. While the relationships between biodiversity and ecosystem functions have been repeatedly discussed in many studies (Lefcheck et al., 2015), there are many debates about the interaction mechanisms involved.
Plant functional traits are thought to reflect the different functions of plants in different environments. In the combined analysis of environmental driving factors and plant responses to change in ecosystem structure and function, plant functional characteristics are widely used (Díaz et al., 2007; Zheng et al., 2011). Several studies have explored the mechanisms underlying grassland vegetation responses to grazing by analyzing changes in functional traits (Louault et al., 2005). Based on plant functional traits, the influence of grazing on grassland vegetation can be explained, and the influence of grazing on grassland ecosystems can be explained by the individual action mechanisms of species. Plant functional traits can be useful predictors of plant responses to grazing. Thus, studying the relationship between plant functional traits and grazing is very important for the effective prediction of ecosystem responses to grazing and grassland management. The morphological and physiological traits of plants are usually related to grazing, such as the regeneration of tillers and carbohydrate redistribution. Long-term grazing can lead to changes in plant morphological and functional traits (Doescher et al., 1997; Zhao et al., 2009). For example, Louault et al. (2005) found that multiple traits of plants were significantly affected by grazing and that the sensitivity of different traits to grazing was significantly different between plants in nongrazed areas and plants in plots with 12 years of continuous grazing. Some researchers have found a significant negative correlation between stocking rate and plant height, plant weight, leaf area, and leaf dry biomass of three dominant species [i.e., Elymus nutans Griseb., Kobresia humilis (C. A. Mey ex Trauvt.) Sergievskay, and Stipa purpurea Griseb.] in the grassland region of Qinghai, China (J. Wang et al., 2016). Steppe vegetation affected by overgrazing tends to be short and small, which is a key factor contributing to decline in plant community productivity (X.-L. Li et al., 2014). Therefore, explaining and predicting the response of species and communities to grazing using plant functional traits is important for understanding changes in ecosystem function caused by changes in individual plant traits.
Stipa breviflora Griseb. is widely distributed in the steppe region of Central Asia, namely the Loess Plateau, Qinghai Tibet Plateau, and Xinjiang in China, as well as in Japan, Mongolian desert steppe regions, and elsewhere in the Eurasian steppe (Coupland, 1993). Therefore, we studied the effects of long-term grazing on S. breviflora individuals in a desert steppe. To account for differences between individual S. breviflora plants, tillering plants were selected for analysis of their individual functional traits. We investigated changes in species richness and the proportion of S. breviflora biomass under long-term grazing pressure. In addition, we analyzed the effects of long-term grazing pressure on aboveground and belowground prototypical traits of tillering individuals. This enabled the identification of the primary plant functional traits that determine the proportion of S. breviflora biomass. The results are of theoretical and practical significance for the restoration of degraded grasslands and biodiversity conservation. Based on the conclusions of previous studies indicating that grazing has a significant negative effect on plant functional traits, we hypothesized that long-term grazing (i) will cause stuntedness of the aboveground and belowground parts of tillering S. breviflora and (ii) force them to allocate more biomass to belowground.
. Material and Methods
Study Site
The study area was a desert steppe located in Siziwangqi, Wulanchabu, Inner Mongolia (41°47′17″ N and 111°53′46″ E, 1,450 m above sea level). This area belongs to a typical temperate continental arid region and is characterized by significant inter- and intra-annual variability in hydrothermal conditions. Topography is primarily low rolling hills, and the primary soil type is classified as calcic kastanozems, with an organic C content of 24.32 g kg−1, an available N content of 63.72 mg kg−1, and an available P content of 3.96 mg kg−1. Mean annual precipitation is 221.7 mm, with rainfall mainly occurring from June to September. The mean annual temperature is 3.7 °C, with the coldest month being January and the hottest being July. The meteorological data were automatically recorded by a CR1000 Meteorological Observation System (Campbell Scientific; Logan, USA). The area is a desert steppe, and the vegetation is dominated by S. breviflora, Artemisia frigida Wild., and Cleistogenes songorica (Roshev.) Ohwicz (Z. Wu, 2010).
Experimental Design
A long-term grazing study site was established in 2004. Grazing was conducted from June 1 to November 31 for 14 years between 2004 and 2017. Twelve paddocks each of 4.4 hm2 in area were constructed using a completely randomized block design (Figure 1), with four stocking rate treatments: control (CK; nongrazed), lightly grazed (LG; 0.91 sheep unit hm−2 half year−1), moderately grazed (MG; 1.82 sheep unit hm−2 half year−1), and heavily grazed (HG; 2.71 sheep unit hm−2 half year−1), with three replicates of each treatment. Stocking rates in this study were calculated using the methods described by M. Wang and Ma (1994) and Z. Wei (2000).
Data Collection and Analysis
From 2004 to 2017, 10 randomly selected 1.5 m × 1.5 m plots were set in each paddock before the onset of greenness, and each plot was fenced by a cage to protect the grass from sheep. In each plot, the aboveground biomass, plant height, projection cover, and abundance of each species were surveyed. Aboveground plant matter was clipped by species, and its fresh weight was measured; after returning to the laboratory, it was dried at 65 °C until a constant dry weight was obtained.
In August 2018, after measuring the S. breviflora plant height inside each cage, we dug a pit (1 m × 1 m × 1 m) near the outside edge of the cage, and flushed the soil profile toward the inside of the cage using a controllable water cannon (pressure less than 0.7 MPa) until the individual S. breviflora plants were completely exposed. All individual plants were placed in water, and the tillering plant samples (at least seven–eight clusters of sample for each plot) were separated. The samples were then transferred to the laboratory as soon as possible.
Three functional traits were assessed for each tillering plant sample: biomass traits, aboveground traits, and belowground traits. The aboveground traits included the leaf traits of plants, while the belowground traits included the root traits of plants (see details in Table 1).
Leaf length was measured as the average value of four sample plants with a DL91150 electronic Vernier caliper (Deli Corporation; Hangzhou, China). The total leaf area was measured using a digital scanner and ImageJ image-processing software. The roots were then separated from the tillering plant sample to estimate the total root length (RL), root average diameter (RAD), root tip number (RTN), root furcation number (RFN), and root crossing number (RCN) using a WinRHIZO root scanning analysis system (Regent Instruments; Quebec, Canada). The roots and leaves were packed in different envelope bags and dried for 48 hr to a constant weight at 65 °C and weighed with an electronic scale (precision 1/1,000) to calculate aboveground plant dry weight (leaf and stem), belowground dry weight (root), and root–shoot ratio. Specific leaf area (SLA) was calculated as the ratio of leaf area to leaf dry weight, and the specific root length (SRL) was calculated as the ratio of root length to root dry weight.
Table 1
Indicators of functional traits of tillering Stipa breviflora individuals.
After testing the normality of data, the difference in aboveground net primary productivity and the difference between S. breviflora aboveground traits and belowground traits between the four stocking rates were analyzed by repeated measures analysis of variance (ANOVA) and one-way ANOVA, and a post hoc analysis was conducted using the least significant difference (LSD) method with a significance testing level of α = 0.05.
Leaf and root biomass and its related phenotypic traits were analyzed using Pearson correlation analysis and partial least squares regression (PLSR). To establish the regression equation among biomass and phenotypic traits, the variable importance projection (VIP) of the regression equation was calculated to determine the various influencing factors.
where Rd(y, th) = R2(y, th), reflecting the accuracy of using the principal component th to explain variable y, which represents the explanatory ability of principal component th to variable y. Thus, ∑h Rd (y, th) represents the cumulative explanatory ability of principal component t1, t2, …, th to y, and R(y, th) is the correlation coefficient between y and the principal component th. whj is the jth component of axis wh. ωXj is the weight of the factor Xj. When VIPj is greater than 1, the independent variable Xj (j = 1, 2, …, k) can explain y. Repeated measures ANOVA and one-way ANOVA were conducted in SAS 9.4 (SAS Institute; Cary, USA) to detect the differences in measured indicators under different treatment (stocking rate), and PLSR analysis was conducted in the SIMCA-P (Umetrics; Umeå, Sweden) to find the primary factors influencing the net primary productivity.
. Results
Species Richness and Contribution of Stipa breviflora Under Different Long-Term Grazing Intensities
Figure 2 shows the changes in species richness under the four stocking rates from 2004 to 2017. The principal species composition of the experimental area included the dominant species, namely S. breviflora, A. frigida, C. songorica, Convolvulus ammannii Desr., and Kochia prostrate (L.) Schrad.; common species, namely Heteropappus altaicus (Willd.) Novopokr., Potentilla bifurca L., Ceratoides latens (J. F. Gmel.) Reveal et Holmgren, Allium tenuissimum L., Caragana microphylla Lam, and Salsola collina Pall; and the less common species, namely Chenopodium aristatum L., Cymbaria dahurica L., and Lappula myosotis V. Wolf. Although there was no obvious trend in species richness with respect to years of grazing, the species richness showed remarkable differences among the four stocking rates. The higher the stocking rate, the lower was the species richness and differences in species richness with grazing years.
Figure 2
Species richness under four stocking rates from 2004 to 2017. The F value and p values of the effects of time, stocking rate, and their interactions obtained by repeated measures ANOVA were adjusted according to Greenhouse–Geisser’s method as the data failed to pass the Mauchly’s test of sphericity. Different letters in the same column indicate significant differences among treatments, and no letter notes were added when there were no significant differences among treatments. CK – nongrazed; LG – lightly grazed (0.91 sheep unit hm−2 half year−1); MG – moderately grazed (1.82 sheep unit hm−2 half year−1); HG – heavily grazed (2.71 sheep unit hm−2 half year−1).
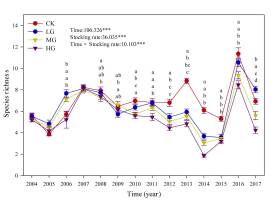
The proportion of S. breviflora biomass in the community from 2004 to 2008 indicated a lower contribution of S. breviflora to the community biomass in the short-term (i.e., within 4–5 years) grazing (see Figure 3). However, as the duration of grazing treatments increased, a strong increase in the percentage of S. breviflora in community biomass was observed after 2009, with it reaching 93% in 2017 under HG treatment.
Differences in Traits of Tillering Stipa breviflora Individuals
We found no obvious trend for species richness, but a clear evidence of an increasing contribution of S. breviflora to community aboveground net primary productivity with duration of grazing. To explain this phenomenon, we further examined the responses of traits of tillering S. breviflora individuals to long-term grazing and their effects on S. breviflora tillering net primary productivity. The differences in leaf and root traits of tillering S. breviflora plants under the four stocking rates are shown in Table 2. Although there was no clear evidence of differences between aboveground and belowground productivity in the four stocking rates, the root–shoot ratio under HG was significantly greater than that under CK and MG (p < 0.05).
Figure 4 reflects the differences in aboveground prototypical traits of tillering individual plants under the four stocking rates. The ungrazed (CK) and lightly grazing (LG) paddocks possessed larger LWE, LA, LL, and H than other grazing areas (p > 0.05), while the lightly grazing paddock showed the lowest SLA among the four stocking rates.
Six root traits were significantly different among the four stocking rates (p > 0.05, see the belowground root traits in Figure 5). The RAD attained higher values under the ungrazed (CK) treatment (p > 0.05), while the other five belowground traits (RL, SRL, RTN, RFN, and RCN) achieved greater values in grazed (LG, MG, or HG) treatments (p < 0.05).
Table 2
The aboveground and belowground biomass of tillering Stipa breviflora individuals under four stocking rates.
Figure 4
Aboveground traits of tillering Stipa breviflora individuals under four stocking rates. LWE – individual leaf mass; LL – average leaf length; H – average plant height; LA – leaf area; SLA – specific leaf area; LN – leaf number; CK – nongrazed; LG – lightly grazed (0.91 sheep unit hm−2 half year−1); MG – moderately grazed (1.82 sheep unit hm−2 half year−1); HG – heavily grazed (2.71 sheep unit hm−2 half year−1). Different letters represent significant differences between treatments (p < 0.05).
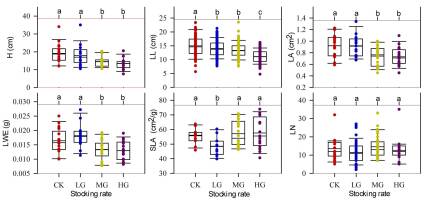
Figure 5
Root traits of tillering Stipa breviflora individuals under four stocking rates. RAD – root average diameter; SRL – specific root length; RL – total root length; RFN – root furcation number; RTN – root tip number; RCN – root crossing number; CK – nongrazed; LG – lightly grazed (0.91 sheep unit hm−2 half year−1); MG – moderately grazed (1.82 sheep unit hm−2 half year−1); HG – heavily grazed (2.71 sheep unit hm−2 half year−1).
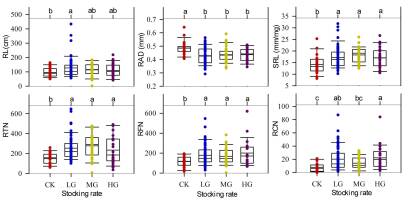
Leaf and Root Biomass, and Related Individual Phenotypic Traits of the Tillering Individuals
We found diverse patterns for the effects of tillering traits on aboveground/belowground net primary productivity of S. breviflora individuals (Figure 6). The significant positive correlation (p < 0.01) between LN and leaf biomass showed that LN is the only crucial tillering trait that determines the magnitude of aboveground net primary productivity of tillering S. breviflora (VIP > 1, contribution = 73%). However, the significant positive correlation (p < 0.05) of five root traits (RL, RCN, RFN, and SRL; VIP > 1, cumulative contribution reached 77%) and the negative correlation (p < 0.01) with tillering S. breviflora root biomass reflected the diverse mediation approaches of S. breviflora to increase belowground net primary productivity.
Figure 6
The variable importance projection (VIP) of tillering Stipa breviflora leaf biomass and aboveground functional traits (left), and the variable importance projection (VIP) of tillering S. breviflora root biomass and belowground functional traits (right). “+” indicates that the trait is positively correlated with the biomass (p < 0.01); “−” indicates that the trait is negatively correlated with the corresponding biomass (p < 0.01). LN – leaf number; SLA – specific leaf area; LL – average leaf length; LWE – individual leaf mass; LA – leaf area; H – average plant height; RL – total root length; RCN – root crossing number; RFN – root furcation number; SRL – specific root length; RTN – root tip number; RAD – root average diameter.
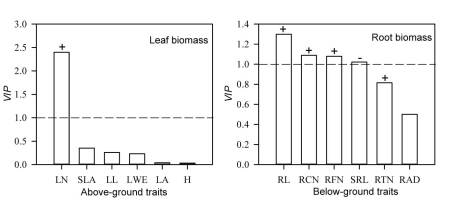
. Discussion
Change in Species Richness and the Proportion of Stipa breviflora in Community Biomass
Long-term monitoring of grassland species richness shows that the effect of grazing on species richness is accumulating in a long-term process, while the effects of short-term grazing are not obvious. Long-term grazing caused a greater difference in the percentage of S. breviflora biomass at different stocking rates. Because of the different characteristics of each species (e.g., grazing resistance and growth rates) and different effects of livestock activities on plants (e.g., grazing and trampling) (Herrera et al., 2012; Rotundo & Aguiar, 2008; Wan et al., 2011), different species have different response modes to grazing and fencing (G. L. Wu et al., 2009). Herbivores shape plant communities through selective foraging. For example, sheep select large, late-flowering herbs with a low leaf C/N ratio. Species that increased in abundance in response to enhanced grazing pressure were generally small in length and had high root–shoot ratios, exhibiting traits that reflect both resistance (through avoidance) and tolerance (through regrowth capacity) strategies (Evju et al., 2009). Different species thus have their own adaptation strategies, and species are variably sensitive to different stocking rates (Zheng et al., 2015). Leymus chinensis in the meadow steppe adapts to grazing disturbance through trade-offs between plant size and nutrient use strategies (Daorina et al., 2016; Lü et al., 2015). When environmental interference does not exceed the growth tolerance of a species, the species can grow normally in its community. The cumulative effect of long-term grazing exceeds the tolerance limit of some species, hindering growth. Furthermore, the greater the stocking rate, the more significant the cumulative effect. Hence, the number of species decreased, and the productivity of grassland declined under long-term high stocking rates. Compared with other species, S. breviflora has stronger grazing resistance (X. Wang et al., 2018); therefore, long-term grazing at a high stocking rate caused the percentage of S. breviflora in community biomass to increase. Additionally, changes in species richness became more volatile as time progressed. This may be related to differences in annual hydrothermal factors; for example, precipitation and temperature differences cause changes in grassland community composition and productivity (Pierre et al., 2016). Long-term grazing resulted in insignificant differences in species richness among different stocking rates, which is directly related to changes in species composition and productivity. In addition, because different herbage traits have different responses to grazing, grazing resulted in the richness of different herbage life forms as the community changed (Zheng et al., 2015), which in turn affected aboveground net primary productivity and species richness of grassland.
Long-term grazing did not change the dominant position of S. breviflora, but in the process of community change, the percentage of S. breviflora biomass increased; in the HG treatment, the dominant position of S. breviflora became more pronounced. In 2017, the percentage of S. breviflora biomass exceeded 90% of the total biomass of the community. This may be because the species is resistant to drought and also exhibits high yield and strong resistance to the direct effects of animal grazing, while long-term grazing leads to decreases in forbs (Chen et al., 2006). As species richness decreased, the competitive advantage of S. breviflora became more apparent, especially under HG conditions. It has been noted that the variation in individual plant functional traits and the response of traits to grazing were positively correlated. With increasing stocking rate, the variation and plasticity of leaf traits affecting the biomass of S. breviflora increased, which indicates an ecological response mechanism of the species (J. Li et al., 2017).
Difference in Functional Traits of Tillering Stipa breviflora Individuals
Some scholars have noted that plant phenotypic changes in functional traits represent the overall performance of plants as they adapt to changes in the external environment, so the change in phenotypic functional traits is a reflection of the adaptability of individual plants to their environment (Louault et al., 2005; Mooney et al., 2010). In line with the first point of our hypothesis, we found that grazing resulted in declines in S. breviflora LA, LL, and H. Furthermore, LWE, LA, LL, and H were significantly lower under HG, whereas the SLA was the smallest with light stocking rate. As a result, the leaf biomass of S. breviflora was the largest, but the light effect was weaker under light grazing. Grazing has a significant effect on plant size (individual biomass) and traits (e.g., leaf N content and leaf density) that affect the growth and development of most species (Zheng et al., 2015). For example, the H, plant weight, LA, and leaf dry biomass of three dominant species (Elymus nutans, Kobresia humilis, and Stipa purpurea) in an alpine meadow were found to have significant negative relationships with the stocking rate (J. Wang et al., 2016). This might be because plants commonly generate one or more avoidance strategies, such as tiller regeneration, leaf size reduction, SLA dynamic changes, anatomical changes of plant leaves, and changes in light capture capabilities (J. Wang et al., 2016). The leaf thickness of the three species in that study usually showed a unimodal response to grazing, while SLA generally showed a quadratic relationship with grazing intensity. This is similar to the response of S. breviflora to stocking rate, with a greater influence on S. breviflora leaf traits. Grazing effects on the S. breviflora root traits (e.g., RTN, RFN, and RCN) increased gradually with increasing stocking rate. This may be a manifestation of plant avoidance mechanisms, as the plant root tip plays a principal role in absorbing moisture, and an increase in the number of vegetation root tips can improve water use efficiency and survival rate (X. T. Wei et al., 2019). Although grazing results in structural damage of the aboveground parts of plants, plant water utilization directly determines the level of individual plant growth and development.
Stipa breviflora Distribution and Correlation of Biomass and Functional Traits
Individual data on S. breviflora plants can be used to analyze the distribution of aboveground and belowground biomass. In line with the second point of our hypothesis, the root–shoot ratio of S. breviflora tiller under HG treatment was significantly greater than that in the CK and MG treatments. The aboveground biomass of S. breviflora is predominantly composed of stems and leaves, whereas belowground biomass is mainly root biomass. However, the biomass of leaves of tillering S. breviflora individuals decreased with an increase in the stocking rate, although the change was not significant. There were no significant differences in the root biomass under different stocking rates. The root–shoot ratio of S. breviflora was significantly increased under HG because the biomass of the stems and leaves decreased. Due to the structural features of S. breviflora, one part of the stem is exposed aboveground, while the other part is belowground. As for the change in stem quality in the belowground part, further discussion and research is needed.
Moreover, grazing leads to changes in plant leaves, roots, and biomass allocation (Archer & Tieszen, 1983). Grazing repeatedly disrupts the functional balance between the root and the leaf crown. Therefore, heavy grazing can lead to an increase in plant root biomass and belowground net primary productivity (Gao et al., 2008). For moderately grazed grassland, the growth rate of the aboveground part was higher than that of the root parts; therefore, individual plant biomass shifted to aboveground biomass, and the root cap ratio decreased (Jatimliansky et al., 1997). Others have noted that belowground biomass and belowground net primary production decrease with increasing stocking rate and increase with increasing precipitation (Chen et al., 2006). However, the premise of this conclusion must be based on the actual local stocking rate, and the impact of precipitation requires long-term data for verification. Long-term continuous grazing, especially at high stocking rates, prevents the growth of individual plant parts. However, long-term grazing exclusion slows the circulation of materials and community structure changes such that the living space for the aboveground parts of individual plants becomes smaller. This eventually leads to belowground transfer of individual plant biomass. Despite these propositions, our study found that heavy grazing resulted in an insignificant reduction in the aboveground biomass of S. breviflora. The primary reason for this result may be that the number of tillers per bunch of grasses varied between different stocking rates, despite the lack of significant differences in tillering biomass among different stocking rates.
When establishing the correlation between biomass and phenotypic functional traits of S. breviflora, we found that aboveground biomass of tillering S. breviflora individuals was influenced by leaf number index, while an increase in RL and a decrease in SRL were associated with positive accumulation of root biomass. Therefore, root volume and total root surface area are the principal traits that determine root biomass changes. However, there were no significant differences in the leaf number, root volume, and total root surface area of S. breviflora tillers under different stocking rates. Therefore, there were no significant differences in leaf and root biomass under different stocking rates. Plant leaf photosynthesis and respiration, water use, and other ecological functions play important roles in ecosystem functioning (Nicotra et al., 2011). For S. breviflora, the aboveground part is mainly leaves, and the leaf area directly affects the strength of photosynthesis, so leaves play a decisive role in the accumulation of aboveground biomass. However, in our study, the aboveground biomass of S. breviflora tillers was not significantly different under different stocking rates, which is related to the leaf number of tillering S. breviflora individuals. Although grazing led to a reduction in leaf area, it may also lead to an increase in the number of leaves of individual tillers. The results indicated that there was no significant difference in the aboveground biomass of tillering S. breviflora individuals at different stocking rates. In summary, heavy grazing results in an increase in the number of leaves, but the effect of this increase was not significant. The root serves as an organ for storing organic matter and absorbing water and inorganic nutrients, and plays a key role in grass growth and regeneration (Shi et al., 2007; Veselova et al., 2005). In plants that are disturbed by drought or other stresses, root activity decreases along with a decrease in root biomass (R. Li et al., 2010). The length of the root determines the root biomass, and the specific root length refers to the root length per unit weight, analogous to the relationship between income and cost. When the benefits outweigh the costs, conditions are conducive to the accumulation of root biomass (i.e., the decrease in specific root length is beneficial to the accumulation of biomass). Grazing resulted in significant increase in total root length and specific root length of S. breviflora individual tiller than that in the control. However, it is the increase in total root length and the decrease in specific root length that promotes an increase in root biomass and root vigor (Ren et al., 2021). Thus, grazing did not cause changes in the root biomass of S. breviflora individuals at the four stocking rates. However, it did not cause changes in the root biomass of S. breviflora individuals at the four stocking rates.
In natural grasslands with long-term grazing disturbance, grassland ecosystem function changes due to changes in grassland vegetation traits, species composition, and productivity. Grassland communities can change in response to grazing because of the effects of grazing on the individual characteristics of plants. In the case of the S. breviflora desert steppe, the grazing caused changes in the species composition of the grassland communities, preserving the dominant species, such as S. breviflora, with a high tolerance to long-term grazing. However, some species disappeared with grazing disturbance, leading to a new grassland community structure and composition.
. Conclusions
Long-term grazing resulted in greater differences between different stocking rate conditions with respect to species richness and the percentage of S. breviflora biomass in the total community biomass. As the stocking rate increased, species richness declined, and S. breviflora biomass accounted for a greater proportion of the total community biomass. Long-term heavy grazing resulted in significant reductions in LWE, LL, H, and LA of S. breviflora tillers aboveground. The RAD in the CK treatment was the highest among all four stocking rates. The RTN, SRL, RFN, and RCN in the CK treatment were significantly lower than that in the HG, and the RL in the CK treatment was significantly lower than in the LG. The individual S. breviflora root–shoot ratio in the HG treatment was significantly greater than that in the CK and MG treatments, showing that grazing leads to changes in the allocation of aboveground and belowground biomass of tillering S. breviflora individuals. The main trait influencing leaf biomass change in tillering S. breviflora individuals was LN, and the main root traits that affected the change in root biomass were RL and SRL. Furthermore, there was no significant difference in LN among the four stocking rate treatments, which was the main reason for the lack of a significant difference in the biomass of leaves and roots among the four stocking rate treatments. These results obtained on analyzing plant functional traits might help us predict the responses of species and communities to grazing.