This article discusses the autonomic nervous system, which is responsible for involuntary reactions such as heart rate, blood pressure and respiration. This is a Self-assessment article and comes with a self-assessment test.
NT Self-assessment articles offer bitesize CPD and are accompanied by multiple-choice assessments with feedback. Test yourself on this article or click here to choose other subjects from the Self-assessment archive.
Abstract
This article – the sixth in a series about the nervous system – discusses the function of the autonomic nervous system, which is a component of the peripheral nervous system. It regulates involuntary processes including heart rate, respiration, blood pressure, body temperature, digestive processes and urinary functions. The autonomic nervous system is divided into the sympathetic nervous system and the parasympathetic nervous system.
Citation: Bayram-Weston Z et al (2022) Nervous system 6: the autonomic nervous system – anatomy and function. Nursing Times [online]; 118: 8.
Authors: Zubeyde Bayram-Weston is senior lecturer in biomedical science; Maria Andrade-Sienz is honorary associate professor in biomedical science; John Knight is associate professor in biomedical science; all at the College of Human and Health Sciences, Swansea University.
- This article has been double-blind peer reviewed
- Scroll down to read the article or download a print-friendly PDF here (if the PDF fails to fully download please try again using a different browser)
- Assess your knowledge and gain CPD evidence by taking the Nursing Times Self-assessment test
- Click here to see other articles in this series
Introduction
The first article in this series introduced the nervous system, which comprises the central nervous system (CNS) and the peripheral nervous system (PNS). The next two articles focused on the CNS, and the following two on the PNS. This sixth and final article of the series continues to discuss the PNS, focusing on the structure and function of the autonomic nervous system (ANS).
As its name suggests, the ANS is primarily the self-regulating division of the nervous system. It consists of motor neurons that convey information from the spinal cord and brain stem to the cardiac tissues and multiple regions of smooth muscle and glandular epithelial tissue. In this way, the ANS regulates involuntary functions including:
- Heart rate;
- The vasodilation and vasoconstriction of blood vessels that allow vascular tone to be maintained;
- The movement of food through the digestive system via peristalsis;
- The release of secretions by a variety of endocrine and exocrine glands.
Although the ANS is regarded as part of the PNS, it has active components that reside in both the CNS and the PNS.
The dendrites and neuronal cell bodies of some autonomic neurons are in the grey matter of the spinal cord or brain stem. Their axons extend from these structures and terminate in a ganglion, which is a collection of cell bodies outside the CNS. The peripheral autonomic nerves mainly carry efferent fibres. As shown in Fig 1, the motor component of the ANS consists of two neurons:
- A preganglionic neuron, which is myelinated and conveys action potentials from the spinal cord to the ganglion;
- A postganglionic neuron, which is unmyelinated and relays action potentials to the effector tissues, such as smooth muscle or glands (Huether and McCance, 2017).
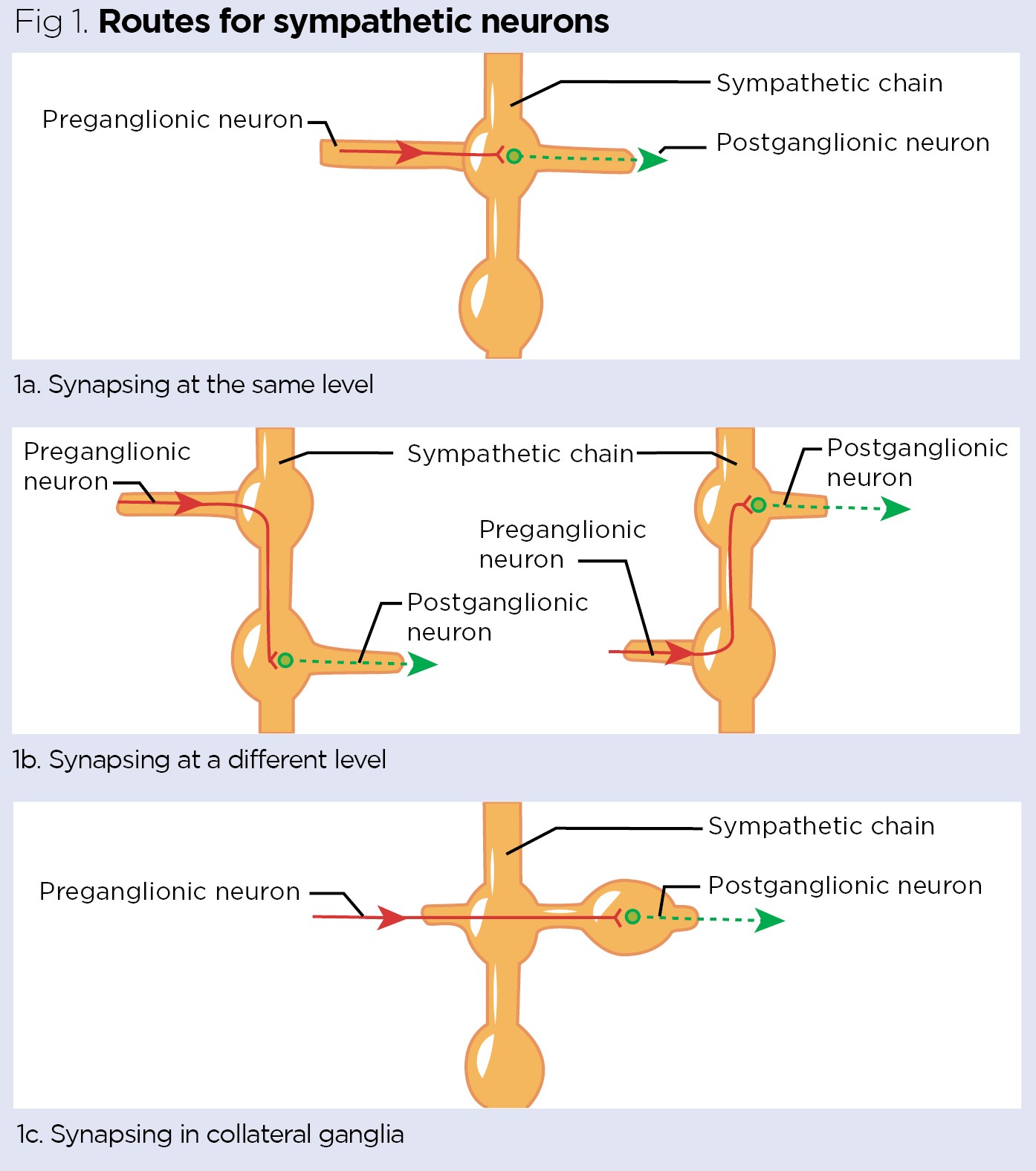
This organisation differs significantly from the somatic nervous system, where only a single motor neuron travels from the CNS to an innervated structure, such as a skeletal muscle, for example allowing one to wiggle a toe.
The two divisions of the ANS
The ANS is separated both structurally and functionally into two divisions: the sympathetic nervous system (SNS) and the parasympathetic nervous system (PSNS). When in balance, these two systems work together well in the body. However, they cannot both work at the same time: one must be ‘switched off’ for the other to work (Kiernan and Rajakumar, 2014).
As discussed in the previous article in this series, the spinal cord consists of a roughly H-shaped area of grey matter surrounded by white matter; the autonomic areas are located in the lateral horn of the grey matter. The cardiovascular and respiratory centres are in the brain stem (in the reticular formation). Both the SNS and the PSNS are ultimately controlled and regulated by the hypothalamus, and the paraventricular nucleus is the key hypothalamic site for this control. Complex neural pathways within the CNS interconnect and relay information between the hypothalamus and sympathetic and parasympathetic divisions.
The sympathetic nervous system
The SNS is so called because it acts in sympathy with the emotions. In association with rage or fear, the SNS triggers the stress response (‘fight or flight’ response), preparing the body for movement by causing increased heart and breathing rates, dilated pupils, slowed digestion, sweaty skin and increased blood flow to muscles. The SNS is therefore an energy-demanding system (VanPutte et al, 2017).
The sympathetic division’s cell bodies are located in the spinal cord, between the first thoracic region (T1) and the second lumbar region (L2); it is therefore also called the thoracolumbar division. The preganglionic axons of the SNS make synapses shortly after leaving the spinal cord in the sympathetic (paravertebral or sympathetic chain) ganglia. These preganglionic axons travel in a variety of ways:
- By directly synapsing with postganglionic neurons in the sympathetic chain ganglion at the same level of the ganglion (Fig 1a);
- By directly synapsing with postganglionic neurons in the sympathetic chain ganglion at the level of their nearest ganglion, which is either up or down in the sympathetic chain (Fig 1b);
- By passing through the sympathetic chain ganglion and synapsing in collateral ganglia (Fig 1c) (Mtui et al, 2016).
If the preganglionic axons pass through the sympathetic chain ganglion and synapse in collateral ganglia (Fig 1c), these fibres are called splanchnic nerves. The splanchnic nerves contribute to the innervation of the internal organs and are named according to the region they are innervating. For example, those innervating the thorax and abdomen are named thoracic, lumbar or sacral splanchnic nerves. Those innervating the aorta are named after the branches the ganglions are closest to and named celiac, superior mesenteric or inferior mesenteric nerves.
Preganglionic sympathetic neurons that supply the adrenal medulla also travel in the splanchnic nerves and do not synapse before reaching the gland. Therefore, the secretory cells in the adrenal medulla are regarded as modified postganglionic neurons. Because preganglionic neurons’ axons are all myelinated, transmission of action potentials to the adrenal medulla is extremely quick and initiates the rapid release of epinephrine and norepinephrine, which are mediators of the stress response. This explains why being suddenly frightened triggers the sympathetic stress response almost instantly.
The parasympathetic nervous system
The PSNS counterbalances the sympathetic system. It initiates the ‘rest and digest’ responses and causes the opposite effects to those of the SNS, including reduced heart and breathing rates, constricted pupils, and secretion by the salivary glands and many other organs of the digestive tract. While the SNS is energy-demanding, the PSNS conserves energy by promoting physiological effects associated with the resting state.
The neuron cell bodies of the PSNS are located in the cranial nerve nuclei and in the sacral region of the spinal cord (Fig 2); this division is therefore also known as the craniosacral division. Unlike in the SNS, in the PSNS the preganglionic fibres travel close to the organ they innervate before making synapses with relatively short postganglionic neurons. The dendrites and cell bodies of parasympathetic postganglionic neurons are in the parasympathetic ganglia (terminal ganglia). These are near the effector they innervate, and their short axons spread out into the walls of the organs. As a result, each parasympathetic postganglionic neuron synapses to a single effector, for example innervation of the lacrimal glands or salivary glands (Patton and Thibodeau, 2016).
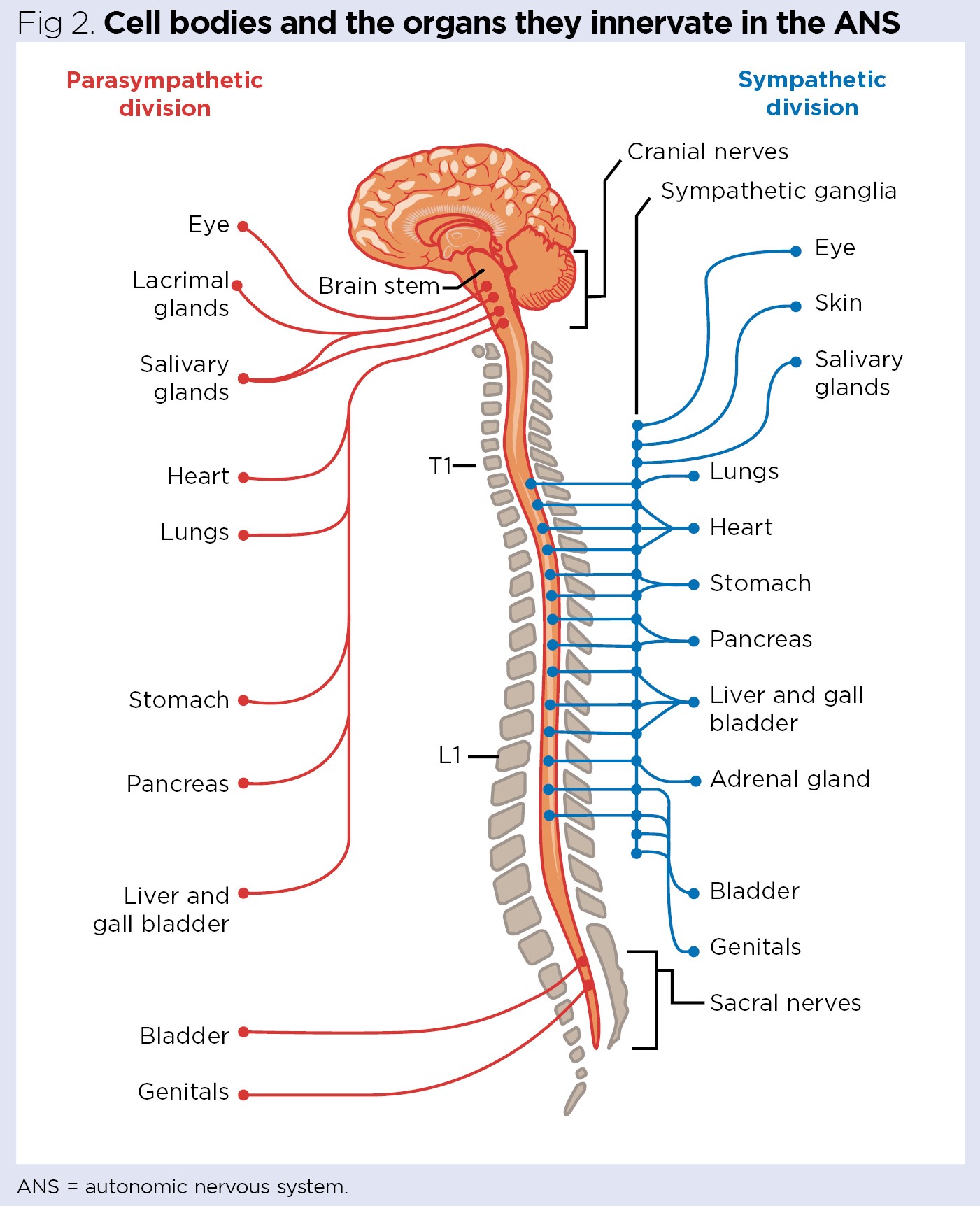
Parasympathetic fibres arising from the brain stem travel to viscera of the head, thorax and abdomen, whereas parasympathetic fibres arising from the sacral region (S2, S3 and S4) run either separately or together with spinal nerves. The preganglionic fibres unite to form the pelvic nerve, which innervates the viscera of the pelvic cavity, such as the bladder, pelvic genital organs and part of the urethra.
Preganglionic parasympathetic fibres emerge in four cranial nerves:
- The oculomotor nerve (CN III);
- The facial nerve (CN VII);
- The glossopharyngeal nerve (CN IX);
- The vagus nerve (CN X).
Parasympathetic stimulation though the oculomotor nerve synapses in the ciliary ganglion (Fig 2). Postganglionic fibres innervate the smooth muscle sphincter of the pupil and the ciliary muscle to produce the accommodation reflex.
The facial nerve synapses in the:
- Pterygoplatine ganglion, which innervates the lacrimal and nasal glands;
- Submandibular ganglion, which innervates the submandibular and sublingual salivary glands (Fig 2).
The glossopharyngeal nerve synapses in the otic ganglion, which innervates the parotid salivary gland (Fig 2).
Strong parasympathetic stimulation though the vagus nerve reduces heart rate and cardiac output, lowering blood pressure and increasing secretion of digestive juices and insulin (Fig 2) (Thibodeau, 2018).
Neurotransmitters and receptors
The physiological effects of autonomic stimulation depend on the nature of the receptors on the target cells. In addition, released neurotransmitters influence the action of the next cell. Drugs are available that either induce or suppress sympathetic or parasympathetic activity. This can be achieved using:
- Agonists, which bind to sympathetic or parasympathetic receptors to upregulate activity;
- Antagonists, which block sympathetic or parasympathetic receptors, thereby preventing the binding of the natural neurotransmitters (McCorry, 2007).
Sympathetic preganglionic fibres and parasympathetic preganglionic and postganglionic fibres release acetylcholine (Fig 3); this is the same neurotransmitter released by somatic efferent neurons. These fibres are characterised as cholinergic, because they release acetylcholine. Most sympathetic postganglionic fibres release norepinephrine (noradrenaline) and therefore are referred to as adrenergic (Fig 3). Only a few sympathetic postganglionic fibres release acetylcholine, such as those that innervate the sweat glands (Marieb and Hoehn, 2018).
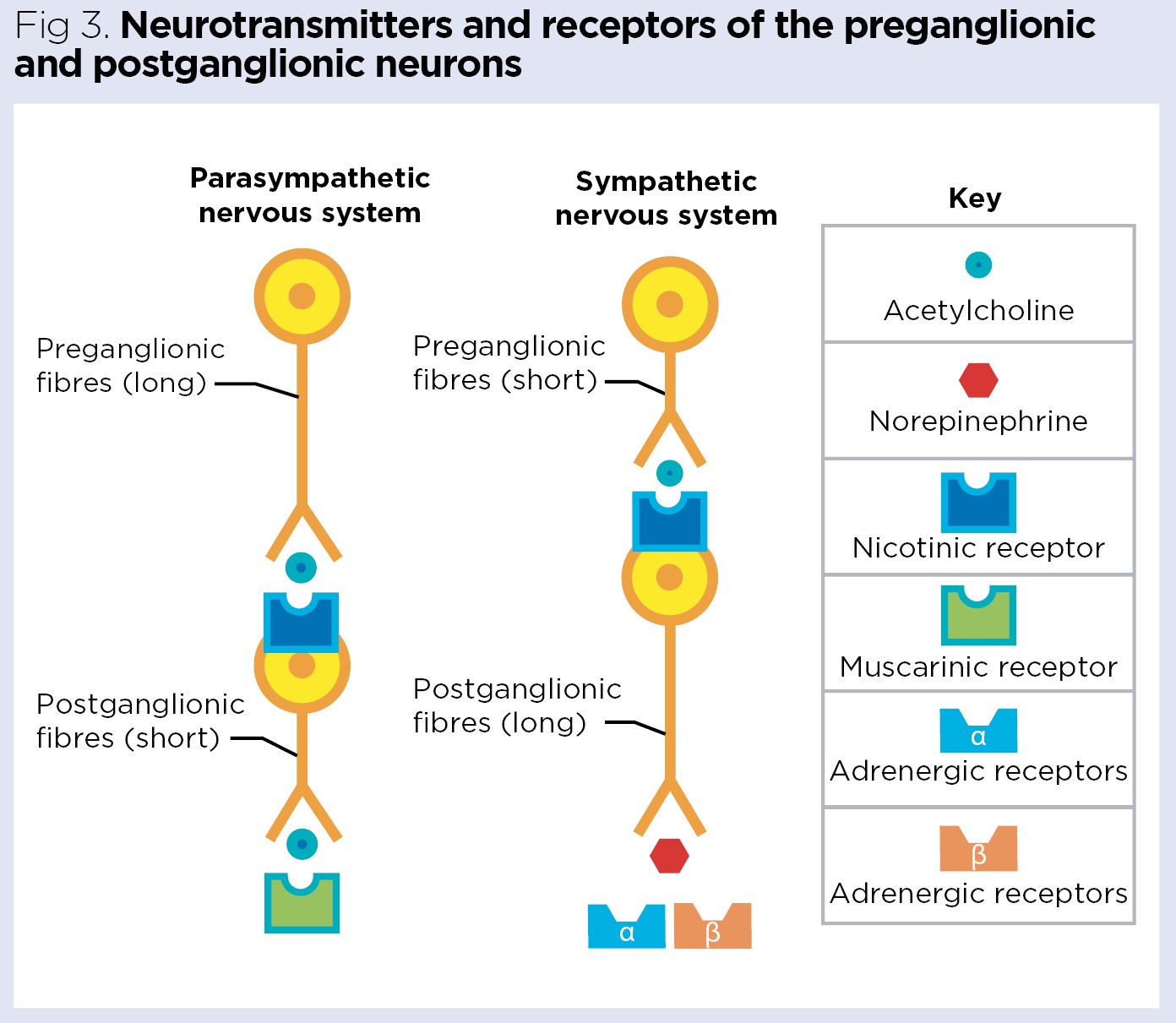
Broadly, two major types of adrenergic receptor are recognised: the α and β receptors (Fig 3). Cells of the effector organs may have only one or both types. The α adrenergic receptors are subdivided according to the action produced – α1 adrenergic activity is associated with excitation or stimulation, whereas α2 adrenergic activity is associated with inhibition or relaxation. Most α adrenergic receptors on effector organs belong to the α1 class. The β receptors are also subdivided – β1 receptors increase heart rate and contractility and cause the release of renin from the kidney, while β2 receptors assist all remaining effects of the β receptors.
Norepinephrine stimulates all α1 and β1 receptors and only certain β2 receptors. The main response to norepinephrine is stimulation of α1 adrenergic receptors that cause vasoconstriction (blood vessel narrowing). In response to vasoconstriction in the extremities, norepinephrine sends blood to essential organs such as the brain and heart. It also creates greater resistance for the heart to beat against, thereby increasing blood pressure. If blood pressure drops dangerously low, norepinephrine can be used to return it to normal.
Epinephrine (adrenaline) is produced in the adrenal glands and stimulates all four types of receptor; it induces general vasodilation due to most of the β receptors in the muscle vasculatures. In effect, epinephrine directly activates and upregulates the SNS (McCorry, 2007).
Nicotinic and muscarinic receptors are the two main types of cholinergic receptors, and both are activated by acetylcholine. However, nicotinic receptors are present on the plasma membrane of chromaffin cells of the adrenal gland and the motor endplate (neuromuscular junctions), whereas muscarinic receptors present on the plasma membrane of all effectors, including cardiac muscle, smooth muscle and glands. Although the same neurotransmitter binds to both types of receptor, the mechanism of action is different in each (McCorry, 2007).
Function of the ANS
Many organs are innervated by both the SNS and the PSNS (Fig 2), but the two divisions generally cause opposite responses. For example, in the small intestine the SNS reduces peristalsis and the PSNS increases peristalsis. However, there are exceptions. For example, peripheral vascular resistance is increased dramatically by sympathetic action but not altered by parasympathetic action.
The SNS favours body function that can support energetic physical activity, whereas the PSNS regulates body function that can conserve and restore energy (Table 1). The effects of sympathetic stimulation are longer-lasting and more widespread than those of parasympathetic stimulation.
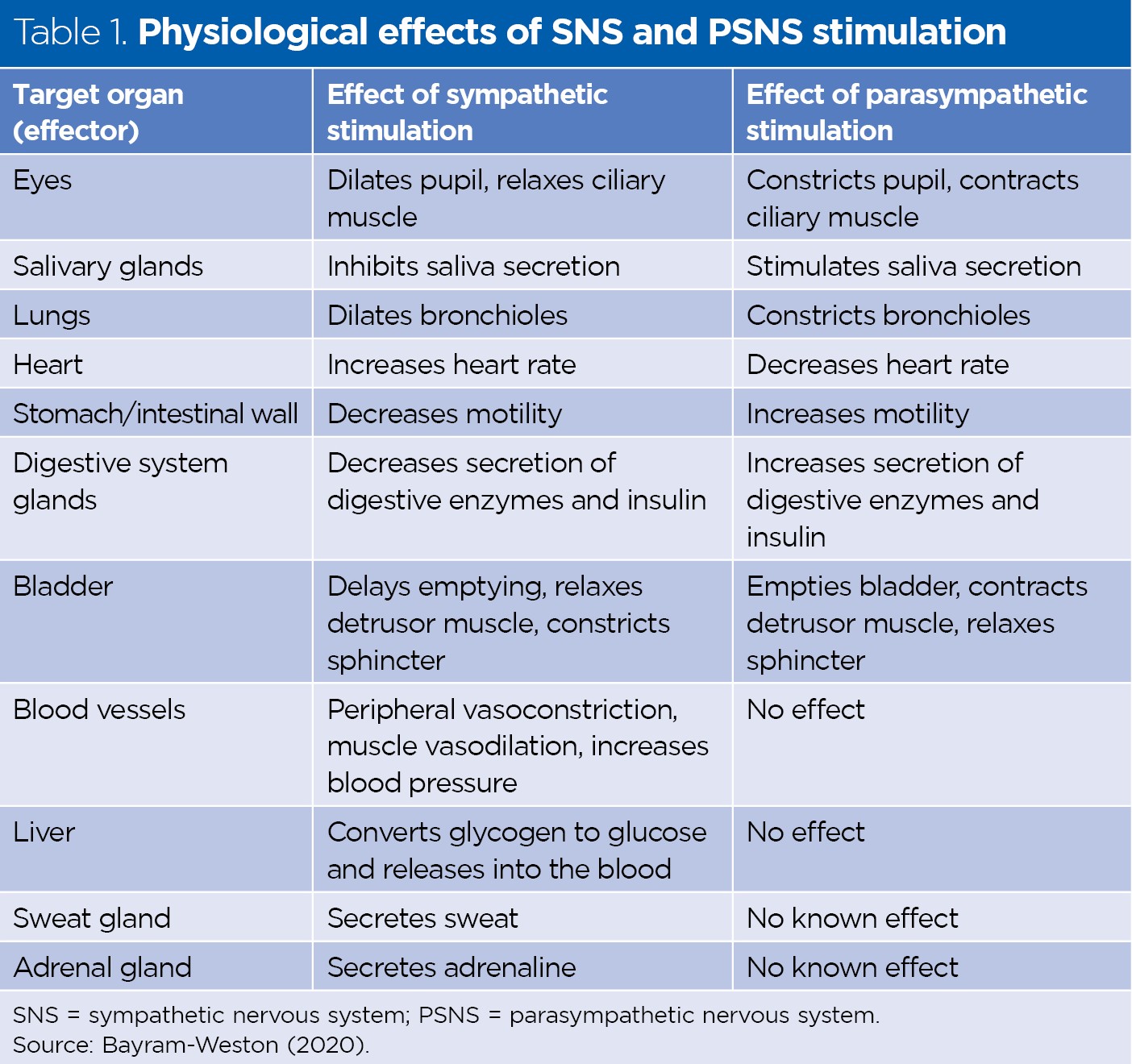
Generally, sympathetic stimulation promotes responses that protect the body, for example increased blood glucose levels, temperature and blood pressure. In emergency situations, a general and widespread response of the sympathetic system occurs. Regulation of vasomotor tone is considered the single most important function of the sympathetic nervous system (Thibodeau, 2018).
Increased parasympathetic activity promotes rest and digestion. It is characterised by reduced heart rate and enhanced organ function, especially in the digestive system. Stimulation of the vagus nerve in the gastrointestinal tract increases peristalsis and secretion and relaxes the sphincters. Activation of the PSNS in the head – provided by the oculomotor, facial and glossopharyngeal nerves – causes pupil constriction, tear secretion and increased salivary gland secretion. Stimulation of the sacral division of the PSNS contracts the urinary bladder and assists the process of genital erection (Fig 2) (Tortora and Derrickson, 2014).
Most blood vessels involved in the control of blood pressure are innervated by sympathetic fibres, so to decrease blood pressure, it is more important to block or paralyse the continuous discharge of the SNS than to promote the activity of the PSNS.
Damage to the ANS
The variety and number of effectors innervated by the ANS means that autonomic disorders have diverse and widespread consequences. Autonomic dysfunction develops when the nerves of the ANS are damaged; this is known as autonomic neuropathy or dysautonomia. It can affect part of or the entire ANS and can range from mild to life-threatening.
People can experience different symptoms depending on the cause, and the effects may be mild to severe. Symptoms of an autonomic nerve disorder include:
- Dizziness and syncope (fainting), especially when standing, caused by a sudden drop in blood pressure;
- Inability to alter heart rate with exercise (exercise intolerance);
- Sweating abnormalities, which could alternate between sweating too much and too little and affect body-temperature regulation;
- Abnormal gastrointestinal motility or digestive difficulties, such as appetite loss, diarrhoea, constipation, nausea, vomiting or difficulty swallowing;
- Urinary problems or bladder dysfunction, such as difficulty starting urination, incontinence, inability to sense a full bladder, or inability to completely empty the bladder, which can lead to urinary tract infections;
- Sexual problems, such as ejaculation difficulties, difficulty maintaining an erection, vaginal dryness or low libido;
- Vision problems, such as blurred vision or inability of the pupils to react quickly to light;
- Tremor or muscle weakness (Mtui et al, 2016; Siegel and Sapru, 2010).
Some patients with poorly controlled diabetes mellitus develop a condition called gastroparesis, in which damage to autonomic nerves leads to poor emptying of the stomach; food remains for long periods and begins to ferment.
Orthostatic (postural) hypotension is also often related to improper regulation of the ANS. This is a condition in which the body is affected by changes in position. After standing up suddenly from a sitting position, the blood pressure in the upper body (including the brain) is temporally reduced due to a shift of blood to the lower part of the body. This can cause dizziness, light-headedness, nausea, sweating and fainting. Lying down improves symptoms. People with diabetes or syphilis often experience orthostatic hypotension, because these conditions cause damage to the sympathetic nerves (Siegel and Sapru, 2010). Orthostatic hypotension also becomes more common in old age, as the ANS’s efficiency and ability to respond quickly decreases. It is therefore often recommended that older patients rise from chairs, beds and the toilet slowly to allow the age-compromised ANS time to respond.
Spinal shock
Spinal shock can occur in response to physical damage to the spinal cord. Because the sympathetic nerves leave the spinal cord between T1 and L2, spinal shock causes a temporary suppression of all reflex activity below the level of injury. However, because the vagus nerve is a cranial nerve and not part of the spinal column, it is not affected. Spinal shock can last from a few hours to a few weeks (Atkinson and Atkinson, 1996).
Spinal shock may lead to a condition called neurogenic shock (vasogenic shock). This is a sudden loss of the sympathetic nerves’ system signals, and the critical features are:
- Sudden loss of sympathetic output that maintains vascular tone, causing hypotension (decrease in blood pressure), peripheral vasodilation and venous pooling;
- Bradycardia (slowing of the heart rate), causing low cardiac output due to unopposed parasympathetic stimulation;
- Poikithermia (poor regulation of body temperature) caused by the SNS diverting blood from the periphery to the internal organs during the severe stress.
Collectively, the drop in blood pressure and slowed heart rate quickly reduce blood flow to the brain, causing fainting (Fig 4).
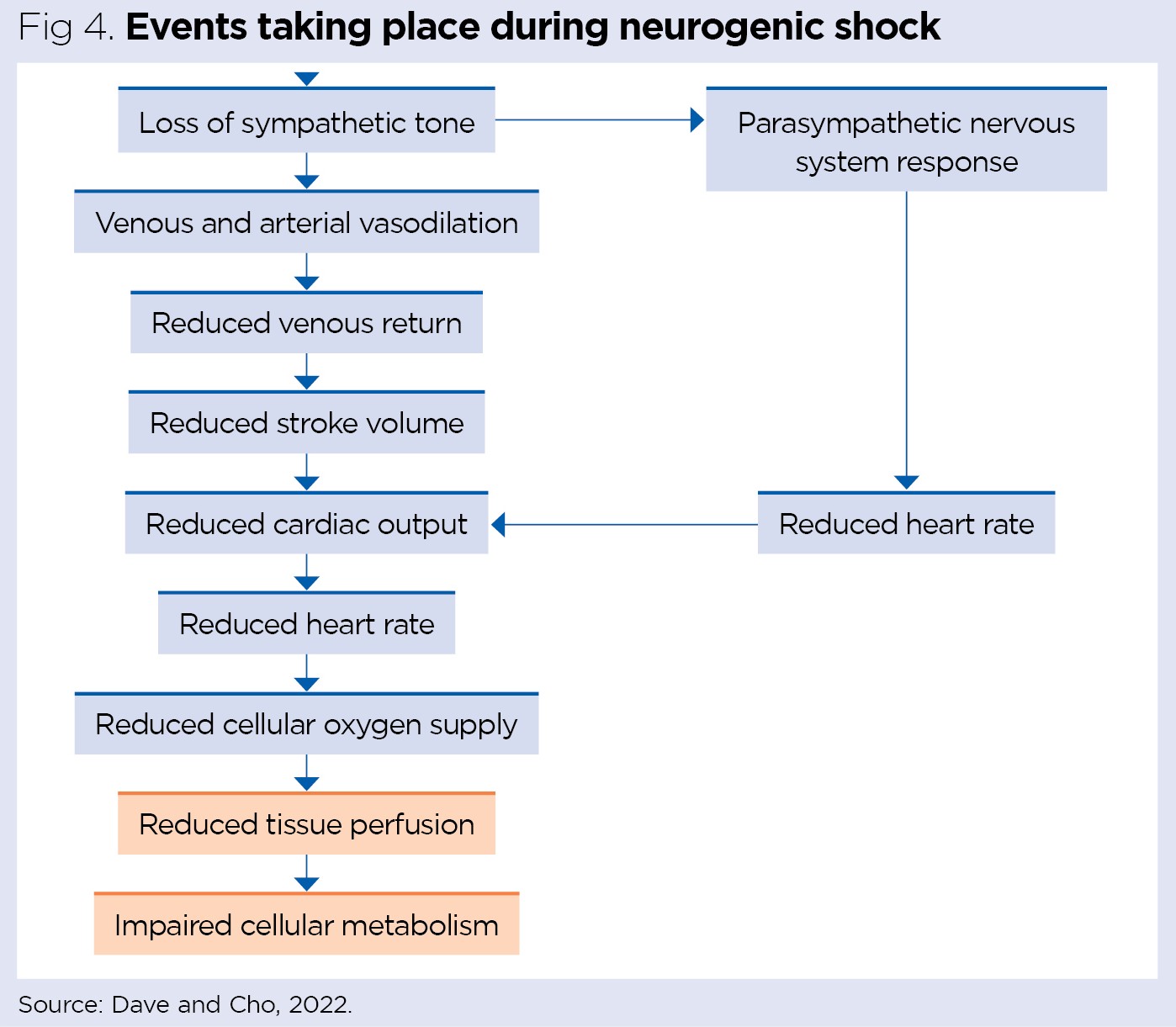
Conclusion
This final article in the series about the CNS has explained that almost every organ system depends on ANS control through its two divisions. The SNS is referred to as the ‘fight or flight’ division because it works under conditions of increased physical activity or stress. The PSNS is known as the ‘rest and digest’ division, because it has more effect under conditions of rest.
Key points
- Complex neural pathways interconnect and relay information between the hypothalamus and the autonomic nervous system
- The autonomic nervous system has two divisions: the sympathetic nervous system and the parasympathetic nervous system
- The sympathetic division acts under physical activity or stress, while the parasympathetic division acts under conditions of rest
- The physiological effects of autonomic stimulation depend on the neurotransmitters released and the nature of the target cells’ receptors
- Autonomic dysfunction develops when the system’s nerves are damaged and has diverse and widespread consequences
Also in this series
- Nervous system 1: introduction to the nervous system
- Nervous system 2: the central and peripheral nervous system I
- Nervous system 3: the central and peripheral nervous system II
- Nervous system 4: the peripheral nervous system – spinal nerves
- Nervous system 5: the peripheral nervous system – cranial nerves
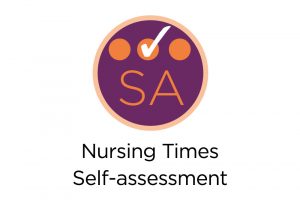
- Test your knowledge with Nursing Times Self-assessment after reading this article. If you score 80% or more, you will receive a personalised certificate that you can download and store in your NT Portfolio as CPD or revalidation evidence.
- Take the Nursing Times Self-assessment for this article
References
Atkinson PP, Atkinson JL (1996) Spinal shock. Mayo Clinic Proceedings; 71: 4, 384-389.
Bayram-Weston Z (2020) The nervous system. In: Knight J et al (eds) Understanding Anatomy and Physiology in Nursing. Sage.
Dave S, Cho JJ (2022) Neurogenic Shock. StatPearls Publishing.
Huether SE, McCance KL (2017) Understanding Pathophysiology. Elsevier.
Kiernan JA, Rajakumar N (2014) Barr’s the Human Nervous System: An Anatomical Viewpoint. Wolters Kluwer.
Marieb E, Hoehn K (2018) Human Anatomy and Physiology, Global Edition. Pearson.
McCorry LK (2007) Physiology of the autonomic nervous system. American Journal of Pharmaceutical Education; 71: 4, 78.
Mtui E et al (2016) Fitzgerald’s Clinical Neuroanatomy and Neuroscience. Elsevier.
Patton K, Thibodeau G (2016) The Human Body in Health and Disease. Elsevier.
Siegel A, Sapru HN (2010) Essential Neuroscience. Wolters Kluwer.
Thibodeau P (2018) Anthony’s Textbook of Anatomy and Physiology. Elsevier.
Tortora GJ, Derrickson B (2014) Principles of Anatomy and Physiology. Wiley.
VanPutte CL et al (2017) Seeley’s Anatomy and Physiology. McGraw-Hill.
Don't miss more great clinical content from Nursing Times
NT Bitesize learning videos – helping you to organise learning to fit in with your schedule
Clinical zones – keep up to date with articles in your clinical subject or nursing role/setting
CPD zone – user-friendly online learning units on fundamental aspects of nursing
Journal Club – clinical articles with discussion handouts for participatory CPD
Practical Procedures – 'how to' guides and teaching materials for clinical procedures
Self-assessment – clinical articles with linked online assessments for bitesize CPD
Systems of Life – applied anatomy and physiology to support your practice
Have your say
or a new account to join the discussion.