Growth form classification for sessile suspension feeders and their distribution in Antarctic fjord, King George Island
Daniela C.S. THORNE1* , Bernabé MORENO1,2 and Aldo G. INDACOCHEA11 Carrera de Biología Marina, Universidad Científica del Sur, Lima 15067, Perú
2 Marine Ecology Department, Institute of Oceanology Polish Academy of Sciences, 81-712 Sopot, Poland
* corresponding author <thornedaniela@gmail.com>
Abstract: Sessile suspension feeders depend primarily on availability of a space to settle and access to the water column. Their sessile nature incapacitates displacement during disturbances thus they rely on their morphology to overcome selective processes. We classified the assemblage of SSF from Mackellar Inlet (King George Island, Antarctica) according to their growth forms (GF) and epibiotic association type, the latter based on direct observation of the epibiotic behaviour of every individual. Organisms that did not comply with any previously established GF were grouped into ‘other GF’. Sampling stations were distributed across the fjord following a gradient based primarily on the distance to Domeyko Glacier (inner, middle, outer sections). Seven GF were recognised in the glaciomarine fjord: tree, bush, stalk, mound, flat, runner, and sheet. Four types of epibiotic associations were identified: basibiont, both facultative epibiont and basibiont, facultative epibiont (non-basibiont), and epibiont. Our results showed that the tree GF were found in the inner and middle sections, mound in middle and outer, and flat across all fjord sections. These GF enhanced GF-diversity since they constituted additional substrate for most of the ‘other GF’ which had primarily an epibiotic strategy. Contrastingly, bush, runner and stalk GF were only found in the outer section of the fjord, thus the most distanced from periglacial disturbances. The GF distribution was consistent with distance to glacier, both in number and strategies. These results highlight the potentialities of the morpho-functional classification applied to Antarctic sessile suspension feeders to help understand their distribution based on adaptive capabilities.
Keywords: Antarctica, macrozoobenthos, functional morphology, life strategies, soft bottom.
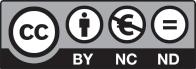
Introduction
Antarctic sessile suspension feeders (SSF) are characterised by high species richness, diversity, and biomass (Brey and Gerdes 1997; Gutt 2007; Clarke 2008; Pabis et al. 2011). Bryozoans, cnidarians, ascidians, and sponges contribute considerably to the benthic structure in the Southern Ocean, and are key components of energy transfer i.e., pelagic-benthic coupling (Brey and Gerdes 1997; Gutt and Starmans 1998; Gili et al. 2001; Tatián et al. 2008a; Alurralde et al. 2019). The functioning of taxa feeding actively or passively on organic particles and small living organisms in the water column transported by sea currents depends on the influx of particulate material (organic and inorganic) and physical disturbances (Gutt 2007; Pabis et al. 2011). The increase of ocean temperature in recent decades caused by climate change enhances sedimentation and ice scouring, have a direct impact on SSF assemblages (Meredith and King 2005; Barnes and Souster 2011; Rückamp et al. 2011; Barnes 2017).
Understanding the mechanisms that regulate species adaptive capacities is essential for predicting which populations are likely to be affected, benefited, or remain neutral to changing environmental conditions (Morley et al. 2019). For SSF, the morphology is a valuable functional trait through which they cope with environmental pressures (Momo et al. 2008; Tatián et al. 2008b; Torre et al. 2014). Basic patterns are repeated between phylogenetically distant taxa that may represent convergent adaptations to cope with similar environmental conditions (Jackson 1979; Kott 1989). Jackson (1979) proposed a classification of growth forms (GF) that divides colonial organisms into six groups based on space occupancy: tree, plate, mound, vine, runner, and sheet. This model was based on geometric parameters (size and shape) that enabled the interpretation of their adaptive significance and specific potential for survival. Some growth forms had increased commitment with survival (trees ≥ plates > mounds > sheets) while the other GF, such as runners and vines, were considered as ‘fugitives’ by exhibiting a refuge-seeking strategy (Jackson 1979).
In an environment with high sediment discharge, such as inner parts of fjords, diversity of functional traits is lower and increases with distance from disturbance, as reflected in peak biological parameter values (Pęcherzewski 1980; Włodarska-Kowalczuk et al. 2005). The GF approach has been used before in polar latitudes for the assessment of distributions and adaptive strategies of benthos in disturbed environments (Teixidó et al. 2004; Momo et al. 2008; Pabis et al. 2014; Torre et al. 2014; Krzemińska and Kukliński 2018). Teixidó et al. (2004) examined macrobenthic recovery patterns after ice disturbance in the Weddell Sea and concluded that GF cover patterns changed along successional stages.
GF classification has the potential to summarize and characterize local-toregional distributions along environmental gradients (Stach 1936; Schopf 1969; Ryland and Warner 1986; Nelson et al. 1988) as well as to increase the
268 Daniela C.S. Thorne, Bernabé Moreno, Aldo G. Indacocheaunderstanding of the environmental pressures and opportunities faced by organisms (Chapin et al. 2000; de Bello et al. 2010; Díaz et al. 2013; PérezHarguindeguy et al. 2013). This knowledge is also essential for understanding the changes that can occur in an ecosystem caused by changing environmental conditions (Smith 1995; Amini et al. 2004). In this study, we aimed to classify the SSF assemblage of Mackellar Inlet into a GF classification and evaluate their spatial distribution. We hypothesize that the distance to the glacier influences the distribution of GF in Mackellar Inlet.
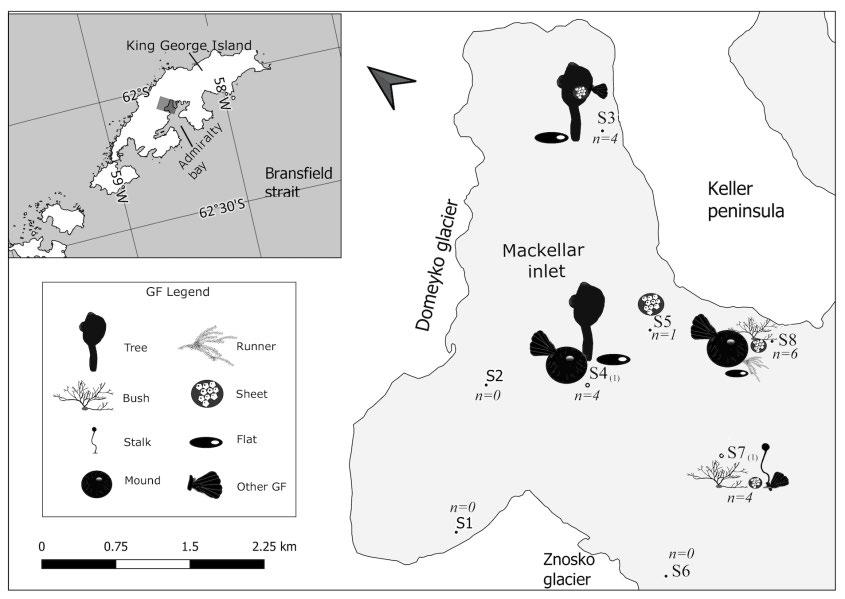
Study area
Mackellar Inlet is a glaciomarine fjord of approximately 16 km2 surface area found within Admiralty Bay, the largest bay of King George Island (KGI) (Fig 1). KGI is situated on the border of Antarctic and Subantarctic climatic zones. Westerly winds predominate in Admiralty Bay, with west-southwest reaching high velocities and generating strong downfall winds (Kowalski 1985; Zwolska and Janecki 1999). These winds induce an outflow of surface waters into the Bransfield Strait and inflow of deep waters that prevents the formation of any distinct parameters (Pruszak 1980; Lipski 1987; Lipski and Rakusa-Suszczewski
1990; Robakiewicz and Rakusa-Suszczewski 1999). Currents in the center of the bay are more intense than within inlets which are low-energy due to tides (Campos et al. 2012).
Subglacial streams bring melted waters into the fjord that contain large amounts of suspended mineral matter (Pęcherzewski 1980). The total amount of inorganic suspended matter in Admiralty Bay over a year varies from 32 264 to 171 000 tons (Rakusa-Suszczewski 1995), and due to climate-induced phenomena a tendency to of increased sedimentation has been reported (Gilbert et al. 2002; Sanders et al. 2010; Cook et al. 2016). The belt of hard-bottom is narrow in Mackellar Inlet, and it quickly changes to soft-bottom dominated by silt and mud (Zielinski 1990). Calving of glaciers also delivers dropstones to nearby areas, enhancing heterogeneity and influencing on benthic biodiversity (Gutt 2001; Ziegler et al. 2016). Some oceanographic phenomena typical for coastal waters are recognized, such as the circulation generated by tides and the nearshore upwelling (Pruszak 1980; Lipski 1987).
Material and methods
Sampling design. — The fjord was intentionally divided into three sections in a distance gradient to Domeyko Glacier, and eight sampling stations across sections were surveyed (Table 1, Fig. 1). In each station four replicates of softbottom macrozoobenthos were collected from an inflatable boat by manually deploying a 0.05 m² Van Veen grab sampler, except in stations S4 and S7 where
Table 1
Sampling stations from Mackellar Inlet, King George Island (WGS84 geographic system) and environmental data measurements. Subscripts in S4 and S7 indicate single samples, no replicates.
only one sample in each was collected (S4(1) and S7(1)). Sessile suspension feeding organisms were retrieved and fixed with 4% formalin, and few grab samples were preserved in 70% ethanol for genetic research.
Three parameters relevant to the distribution of the GF are presented in Table 1. Approximate linear distances of each station to the front of the Domeyko Glacier (Znosko Glacier end for S6) were measured in ArcMap 10.7 using a WorldView-2 satellite image from March 2012 (see acknowledgments). Depth corresponds to the first grab deployed at each station and samples for grain-size analysis were collected by the INGEMMET during the ANTAR XXV Expedition in 2018. Folk (1954) grain-size classification and nomenclature were used (Table 1). For other single measurements of environmental parameters taken at the moment or same week of sampling (Appendix 1).
Growth form classification. — Specimens classified as SSF following classification by Barnes and Sands (2017) were taxonomically identified following Monniot and Monniot (1983), Primo and Vázquez (2007) and Monniot et al. (2011) and for ascidians (Appendix 2) and Hayward (1995) and the Atlas of Antarctic Bryozoa (http://www.iopan.gda.pl/ekologia/Antarctica/ index.php?go=Taxa) for bryozoans (Appendix 3). Additionally, some identities were confirmed by specialists (see acknowledgments). Samples are stored in the scientific collection of the Universidad Científica del Sur (Peru) (Appendix 4).
We adapted the GF classification by Jackson (1979) distinguishing tree, plate, vine, mound, runner and sheet forms to suit better the shallow Antarctic assemblages with morpho-functional descriptions of other authors (i.e, bush, stalk and flat) (Connell and Keough 1985; Hageman et al. 1998; Torre et al. 2014) (Table 2). Eleven taxa have not been previously described morphofunctionally, therefore they were classified as ‘other GF’. The attributes of each GF are focused on the disposition of the colony or body in relation to the water column and substrate, thus in how they occupy space (Connell and Keough 1985). Additionally, we report the type of epibiotic association (i.e., organismsubstrate relationship) found for each taxa, which included: 1) basibiont [b]; 2) both facultative epibiont and basibiont [fe–b]; 3) facultative epibiont (nonbasibiont) [fe]; and 4) epibiont [e] (strict epibiont on this study) (Wahl and Mark 1999).
Data analysis. — Each station was described by GF number (n), GF relative abundance, and number of taxa (S’) per GF. The proportion of GF (%) was calculated for each station. GF number and number of taxa per GF in each station were discussed in relation to distance to the glacier as glacier disturbance is one the most important structural forces for zoobenthic assemblages in Admiralty Bay (Siciński et al. 2011). For distribution analysis, GF abundance data was transformed into presence/absence to produce a two-way cluster analysis (Qmode for stations, and R-mode for GF) using Sørensen (dis)similarity matrix. Routines were performed in PRIMER 6 (Clarke and Gorley 2006).
Descriptions and ecological implications of the growth forms found in a shallow softbottom Antarctic fjord. Classification based on Jackson (1979) but adapted to suit the Antarctic assembly: tree, plate, vine, mound, runner, and sheet proposed by Jackson (1979), bush by Connell and Keough (1985), stalk by Hageman et al. (1998) and flat Momo et al. (2008).
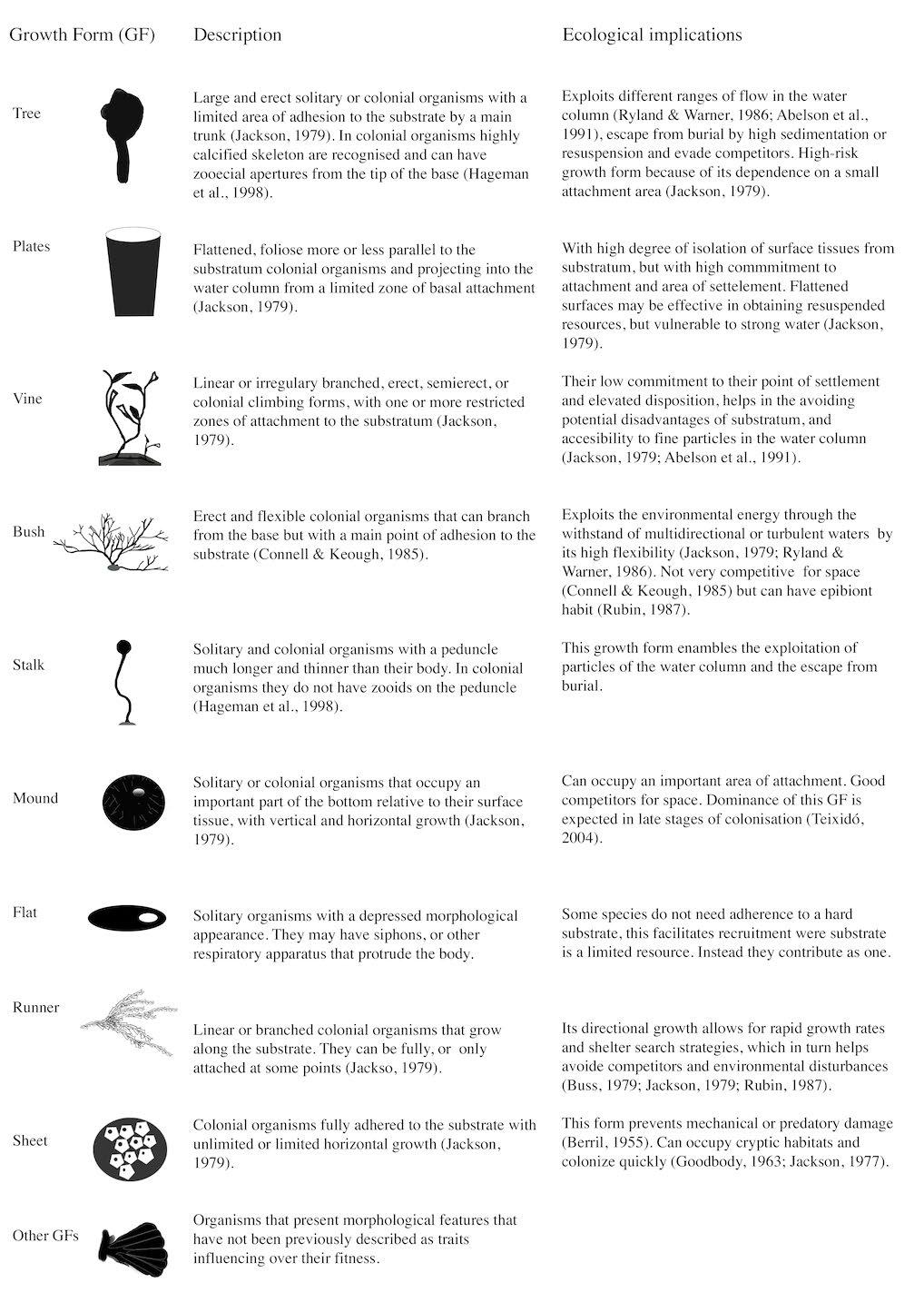
Results
Of the total number of individuals and colonies collected (390), 87% were ascidians and 13% bryozoans within 18 and 13 taxa, respectively. Seven GF were identified for Mackellar Inlet: tree, bush, stalked, mound, flat, runner and sheet; and an additional group was considered (‘other GF’) (Table 3). The latter was the most speciose with eleven taxa, represented mostly by epibiotic colonial ascidians with small rounded or elongated forms, and only one bryozoan taxon was placed in this group as Tubuliporidae (Johnston, 1837). Seven taxa had sheet GF, three taxa had mound and bush GF, and two had tree and flat GF. Each stalk and runner GF were represented by one taxon (Table 3). With the exception of ‘other GF’, all growth forms were composed either by ascidians or bryozoans.
All taxa from the same GF shared an epibiotic association type, except for mound which had both solitary Pyura setosa (Sluiter, 1905) and colonial Aplidium spp. representatives, classified as basibiont and without epibiotic association type (non-epibiont non-basibiont), respectively. Additionally, some GF shared their epibiotic association type: tree, flat and the solitary mound were basibionts; bush and runner were facultative epibionts and basibiont; stalk and sheet were facultative epibionts and the majority of ‘other GF’ were epibionts (Table 3).
No sessile suspension feeders were found in stations S1, S2, and S6. Station S8 had the highest GF number followed by S7, both in the outer section of the fjord. Only one GF was found in S5, located in the middle section of the fjord. Sheet GF had the widest distribution, found at five stations (absent only in S4(1)) (Figs. 1 and 2). Sheet and flat were found in all three sections of the fjord, while bush (S7(1) and S8), runner (S8) and stalk (S7(1)) were only found in the outer stations of the fjord.
The most abundant station was S4(1) with 307 organisms of which > 97% were epibiotic living over an aggregation of basibionts (Fig. 2). In S3 the abundance was low (11), but tree and flat GF allowed for the presence of some epibiotic forms. In S5 sheet was the only GF, with 15 colonies. Stations S7(1) and S8 had 19 and 13 taxa, respectively. Of the total abundance across stations (390 individuals and colonies) 84% were classified as ‘other GF’ (329), and the majority of which (234) were Tylobranchion sp.1 zooids were found individually embedded in their own tunic and were thus counted as such.
The number of GF and taxa changed in relation to the distance from the glacier (Fig. 3). GF number was the highest in station S8, the most distant to Domeyko Glacier, while the inner S1, S2 did not present any SSF. No GF were found in S6 located in the outer section of the fjord but the closest to Znosko Glacier. On the other hand, S3, S4(1) and S7(1) stations from the inner, middle, and outer sections presented the same number of GF (Fig. 3). The number of taxa constantly increased with the distance from the glacier, except for S5 (see Appendix 5).
Table 3
Classification of ascidians and bryozoans found in Mackellar Inlet (King George Island) during the austral summer 2017 (ANTAR XXIV) based on growth forms; and their corresponding epibiotic association type: basibiont (b), facultative epibiont and basibiont (fe–b), facultative epibiont only (fe) (non-basibiont), epibiont (e). A – Ascidiacea, B – Bryozoa.
Growth Forms (GF) taxa taxonomic group epibiotic association type A B b fe-b fe e
Tree Molgula pedunculata (Herdman, 1881) x
Cnemidocarpa verrucosa (Lesson, 1830) x
Nematoflustra flagellata (Waters, 1904) x
Bush
Himantozoum sp. 1 (Harmer, 1923) x
Camptoplites sp. (Harmer, 1923) x
Stalk Sycozoa gaimardi (Herdman, 1886) x
Pyura setosa (Sluiter, 1905) x
Mound
Aplidium sp. 2 (Savigny, 1816)
Aplidium sp. 3 (Savigny, 1816)
Flat Ascidia challengeri (Herdman, 1882) x
Corella eumyota (Traustedt, 1882) x
Runner Himantozoum sp. 2 (Harmer, 1923) x
Fenestrulina sp. 1 (Jullien, 1888) x
Fenestrulina sp. 2 (Jullien, 1888) x
Micropora sp. (Gray, 1848) x
Inversiula nutrix (Jullien, 1888) x
Sheet
Antarctothoa sp. (Moyano, 1987) x
Patinella sp. (Dall, 1871) x
Bryozoa sp. 1 x
Bryozoa sp. 2 x
Tylobranchion speciosum (Herdman, 1886) x
Tylobranchion sp. (Herdman, 1886) x
Aplousobranchia sp. 1 x
Aplousobranchia sp. 2 x
Aplousobranchia sp. 3 x
Other
Cnemidocarpa sp. (Huntsman, 1913) x
Styelidae (Herdman, 1881) x
Polyclinidae sp. 1 (Milne Edwards, 1841) x
Aplidium sp. 1 (Savigny, 1816) x
Molgula enodis (Sluiter, 1912) x
Tubuliporidae (Johnston, 1837) x
The Q-mode (stations) cluster analysis performed on Sørensen dissimilarity matrix shows that S3 and S4(1) were the most similar stations (75% similarity), followed by S7(1) and S8 (60%). These subgroups shared 49% of similarity, and 27% with the outgroup S5 (Fig. 4A). On the other hand, the R-mode (GF) cluster analysis showed two groups: tree, mound, flat and ‘other GF’ at 65% similarity,
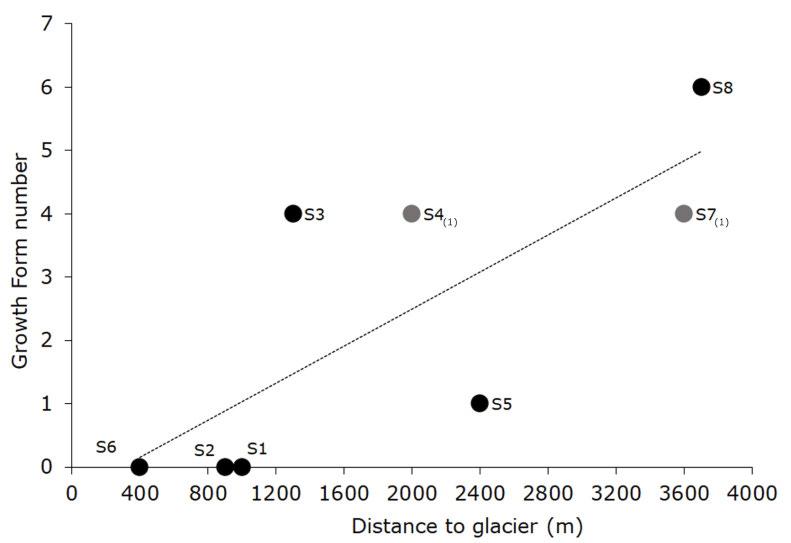
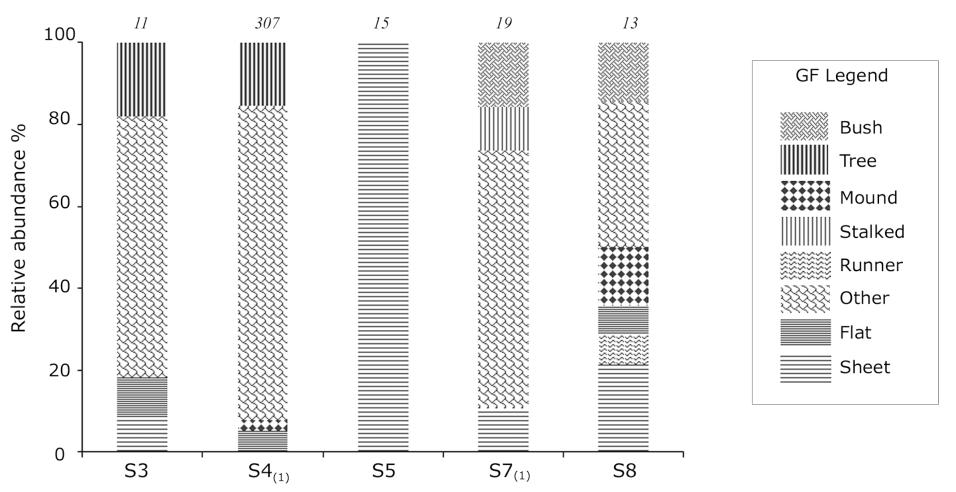
4. Two-way cluster analysis using Sorensen similarity and group-average linking for presence/ absence data. A. Grouping based on sampling stations (Q-mode) categorized by fjord section. Subscript in S4 and S7 indicates only one sample collected on those stations. B. Grouping based on growth forms (R-mode).
and, runner, bush, and sheet at 53% similarity (Fig. 4B). Stalk GF was an outgroup of the previous sharing 20% similarity. The first group is composed by GF found along the fjord while the second group is composed by GF found in the outer section plus the sheet GF with a more opportunistic strategy.
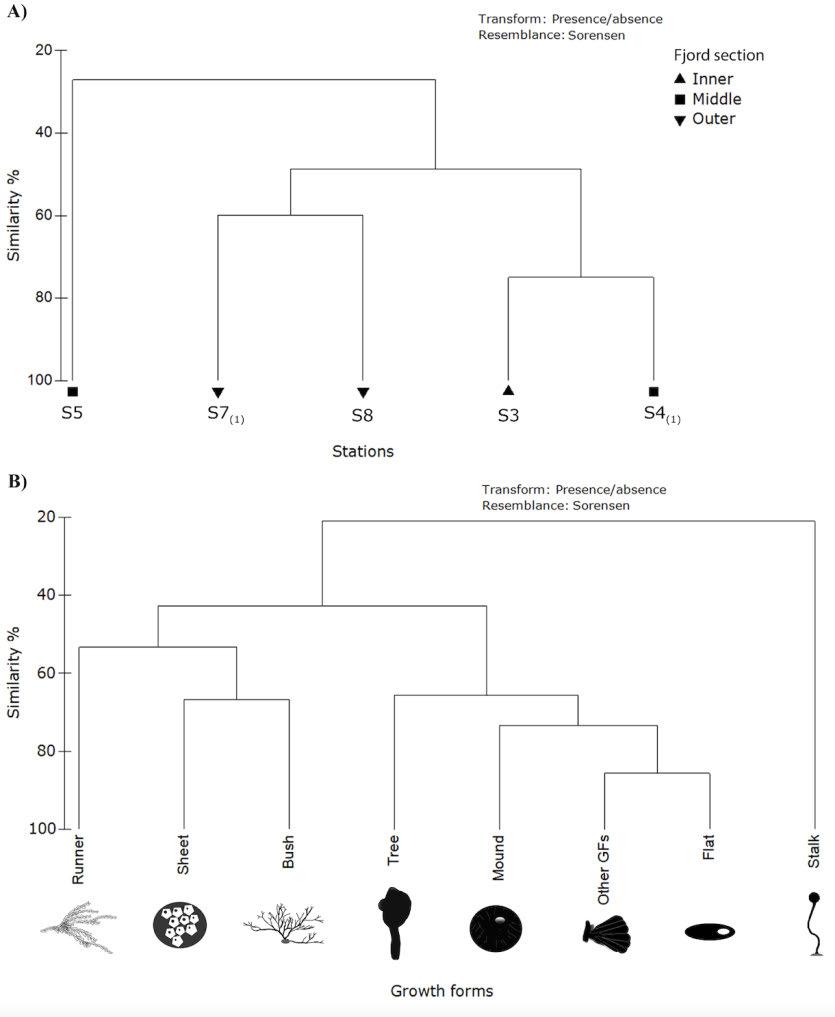
Discussion
Glacier disturbance in the Southern Ocean has been reported as one of the most important factors structuring macro and megazoobenthic assemblages in shallow areas (Echeverría et al. 2005; Echeverría and Paiva 2006; Smale et al. 2008; Siciński et al. 2011; Pabis et al. 2014). Glacier disturbance in fjords can reduce biomass and the local diversity of benthic communities by means of mechanical impact (ice scour), and by the lithogenic input of large amounts of inorganic particles (Pęcherzewski 1980; Barnes and Souster 2011). Encrusting species were reported to dominate inner and shallow sections of fjords, while branched and tuft-like were better represented in deeper and less disturbed areas (Barnes 1995; Pabis et al. 2014). Therefore, spatial distribution of GF could serve as an indicator of the level of disturbance. Glacier disturbances in Mackellar Inlet also seemed to have an influence for diversity and distribution of GF confirmed by positive relationship between the number of GF, and the distance to the glaciers. This result is consistent with other studies that report higher, taxonomic and functional diversity that increase with depth and distancing from source of disturbance (Barnes 1995; Gutt and Starmans 1998; Gutt 2001; Włodarska-Kowalczuk et al. 2005; Krzemińska et al. 2018).
In the present study, the tree-like Molgula pedunculata (Herdman, 1881) and flat Ascidia challengeri (Herdman, 1881) were found in the inner section of the fjord. Tree GF allow their siphons to escape from sediment resuspension, heavy sedimentation and other deleterious processes as these structures usually hover between 10–30 cm above the bottom (Jackson 1979; Tatián et al. 2008b; Torre et al. 2012; Torre et al. 2014), while flat GF were found to cope with high concentration of inorganic particles (200 mgL-1) (Tatián et al. 2008b; Torre et al. 2014). Although through different mechanisms both GF seem to resist high sedimentations, but within some limits. A population decrease of Cnemidocarpa verrucosa (Lesson, 1830) and M. pedunculata and the flat ascidians Corella eumyota (Traustedt, 1882) and A. challengeri were reported from 1994–2010 in Potter Cove as a result of increased sedimentation (Sahade et al. 2015).
A more abundant assemblage was found in the middle section of the fjord in station S4 (1) with various tree individuals ( C. verrucosa ), two flats (A. challengeri) and a solitary mound (P. setosa). Mound GF were found to be dominant in undisturbed areas, while tree GF were usually observed in areas with varying disturbance intensity (Teixidó et al. 2004) Dominance of a GF in a determined environment undoubtedly reflects its success but parameters which control presence/absence also have ecological significance (Hageman et al. 1997). Conditions in this station allowed for the presence of three types of habitats forming GF: tree, flat and mound. These diversified the inner and middle section of the fjord by acting as basibionts for other organisms, especially for the ‘other GF’. Most of these organisms were ascidians with diverse morphologies, from small globulars, as Molgula enodis, to irregular forms, as
Tylobrachion speciosum or Aplidium spp. Previous studies have proved that growth morphologies can influence community development (Nelson 2009). It is assumed that elevated positions are advantageous to feeding on drifting particles due to current increase and in an environment with substratum scarcity epibiosis is essential (Gutt and Schickan 1998).
In the eastern part of the middle section (S5), the low number of GF may be reflecting some degree of physical disturbance. Only small bryozoans with sheet GF were found colonising small rocks (primary substratum) where the cheilostome Inversiula nutrix Jullien, 1888 was dominant with ten colonies. Due to its low two-dimensional profile this species was recorded successfully inhabiting impacted sites (Clark et al. 2017; Krzemińska et al. 2018). Sheet GF are dominant during the first stages of colonisation and are favoured by availability of free surface (Boyer et al. 1990) and diverse substrata (Barnes et al. 1995; Amini et al. 2004; Pabis et al. 2014). In addition, sheet GF was found in all stations except for S4(1), the most abundant one due to a high aggregation of Tylobranchion sp., indicating a low competitive capacity for space, as suggested by Teixidó et al. (2004). Competition for space have been reported to be less relevant for structuring communities in shallow subtidal (0–15 m) and intermediate circalittoral zones (15–30 m) because of the predominance of much more recurrent glacier-related disturbance factors (Dayton et al. 1974), although competition do occur and might have more significant role in these assemblages.
Some GF, such as bush of Nematoflustra flagellata (Waters, 1904), Himantozoum sp. 1 and Camptoplites sp., stalk of Sycozoa gaimardi (Herdman, 1886), and runner GF as Himantozoum sp 2, were found only in the outer section of the fjord and were considered as less competitive (Connell and Keough 1985; Jackson 1979; Teixido et al. 2004). The outer part of the fjord located further from glacier disturbances is exposed to faster bottom currents of central basin having has potential greater larval flux and higher concentrations of chlorophyll-a essential for sessile suspension feeders (Siciński et al. 2011; Campos et al. 2012; Jansen et al. 2018; Krzemińska and Kukliński 2018; Baylón et al. 2019). The morphological flexibility of bush, stalk and runner GF makes them capable for surviving in moderate to high energy waters (Wildish and Kristmanson 1997; Kuklinski 2009). In addition, the presence of gravel in S8 may reduce of impacts by granting protected areas (Krzemińska et al. 2018). Contrastingly, the absence of GF in the western outer section of the fjord (S6) could be explained by vicinity of Znosko Glacier (400 m) as important factor providing high amounts of lithogenic material, hindering settlement.
Jackson (1979) interpreted two main strategies in GF, e.g., committed with survival and the fugitives. GF with strong attachment resources and higher tolerance to disturbances present the first strategy (tree>plate>mound>sheet), while GF with a refuge-seeking behaviour present the latter (runner and vines). In Mackellar Inlet, two groups of GF were formed based on distribution similarities.
The first corresponded to those GF that tolerate the harsh processes of the inner and middle section of the fjord: tree, flat, and mound (all defined as basibionts, except for the colonial mounds) and those that benefit from the additional substrata, or epibionts. Antarctic species are known to form as biogenic substrata (basibionts) structuring benthic communities in disturbed areas (Dayton et al. 1994). Furthermore, colonial invertebrates are much less fouled and appear to be more affected by high sedimentation than solitary organisms (Jackson 1977). This implies fundamental differences that must be considered for future research for assessing distribution of growth forms. The second group corresponds to GF previously found behaving as facultative epibionts which can also be considered a fugitive strategy: bush, runner, and sheet. The first two were only found in the outer section of the fjord while sheet was found across the fjord and has been defined as having an opportunistic behaviour (Pabis et al. 2014).
Conclusions
The composition of GF was different along the fjord: bush, runner and stalk were only found in the outer sections, while tree, flat, sheet and the ‘other GF’ were found in the inner section. Mound GF was found in the middle and outer sections. Sheet GF was distributed along the fjord, attributable to its tolerance to physical processes, but showed reduced competitive capacities compared to the ‘other GF’ with epibiotic strategy. These findings support previous interpretations of GF attributes such as ‘resistant’ and fugitives. The structure of the benthic communities in shallow Antarctic fjords can be influenced by the epibiotic associations of the GF. The presented GF classification may provide relevant insights to deal with uncertainties when projecting responses of Antarctic sessile suspension feeders to future ecological changes and disturbances. However, future research should also be focused on larger spatiotemporal scales and higher resolution to achieve a better understanding of the biological response to environmental processes in Antarctic fjords.
Acknowledgments. — This study was part of the project Environmental factors governing the distribution of macrobenthos in Mackellar Inlet, King George Island, Antarctica financed by Dirección de Asuntos Antárticos’, Ministerio de Relaciones Exteriores (MRE, Peru) and executed by Universidad Científica del Sur (CIENTIFICA) during the Peruvian Antarctic Expedition ANTAR XXIV (austral summer of 2017). The latter provided financial support through Fondo de Tesis 2019. We thank T1 EP Albino Milla and T2 EP Joel Garcia, technical staff of the Compañía de Operaciones Antárticas (COA), for their support during fieldwork. We thank the Instituto Geológico, Minero y Metalúrgico (INGEMMET), specifically José Herrera and Luis Cerpa for providing the granulometric data; and MRE for providing the satellite image. We thank Marcos Tatián for his relevant advice and support during and after the course Taxonomía de Ascidias de
la costa Pacífica Sudamericana y de la Antártida, likewise, we thank Piotr Kukliński for his support in the identification of bryozoans. We extend our thanks to José Antonio Arenas, Báslavi Cóndor-Luján, Hector Aponte and the two anonymous reviewers for their critical review and valuable help in the improvement of the manuscript. We also acknowledge Jeffrey Mangel (ProDelphinus) and Donna Pringle (Dirección General de Investigación, Desarrollo e Innovación, DGIDI, CIENTIFICA) for proofreading the English version of the manuscript and for their useful observations.
Appendix Appendix 1.
Single measurements of four environmental parameters taken from the bottom of the water column of sampling stations the day or week of sampling in Mackellar Inlet, King George Island. Current speed and direction, salinity and temperature obtained with an Aanderaa SEAGUARD RCM CTD. Dissolved oxygen (mg. L-1) measured with a multiparameter HANNA HI 9828 in water samples collected with a 5L Niskin bottle approximately at the depth of grab samples.
Ascidians (Chordata: Ascidiacea) found during the austral summer 2017 in Mackellar Inlet, King George Island, indicating their growth form and their epibiotic association type. b: basibiont; fe–b: both facultative epibiont and basibiont; fe: facultative epibiont; and e: epibiont.
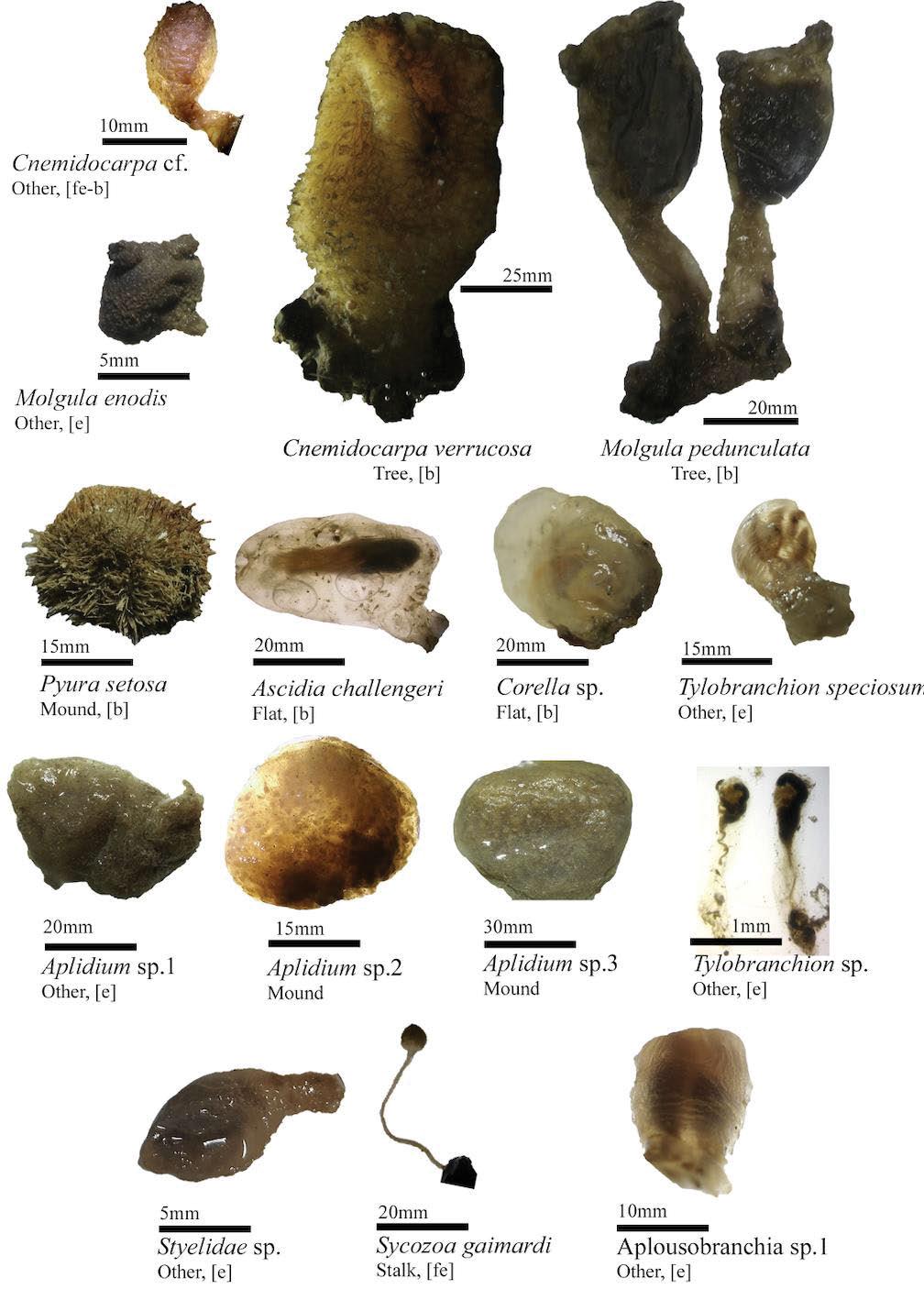
Bryozoans (Bryozoa) found during the austral summer 2017 in Mackellar Inlet, King George Island, indicating their growth form and their epibiotic association type. b: basibiont; fe–b: both facultative epibiont and basibiont; fe: facultative epibiont, and e: epibiont.
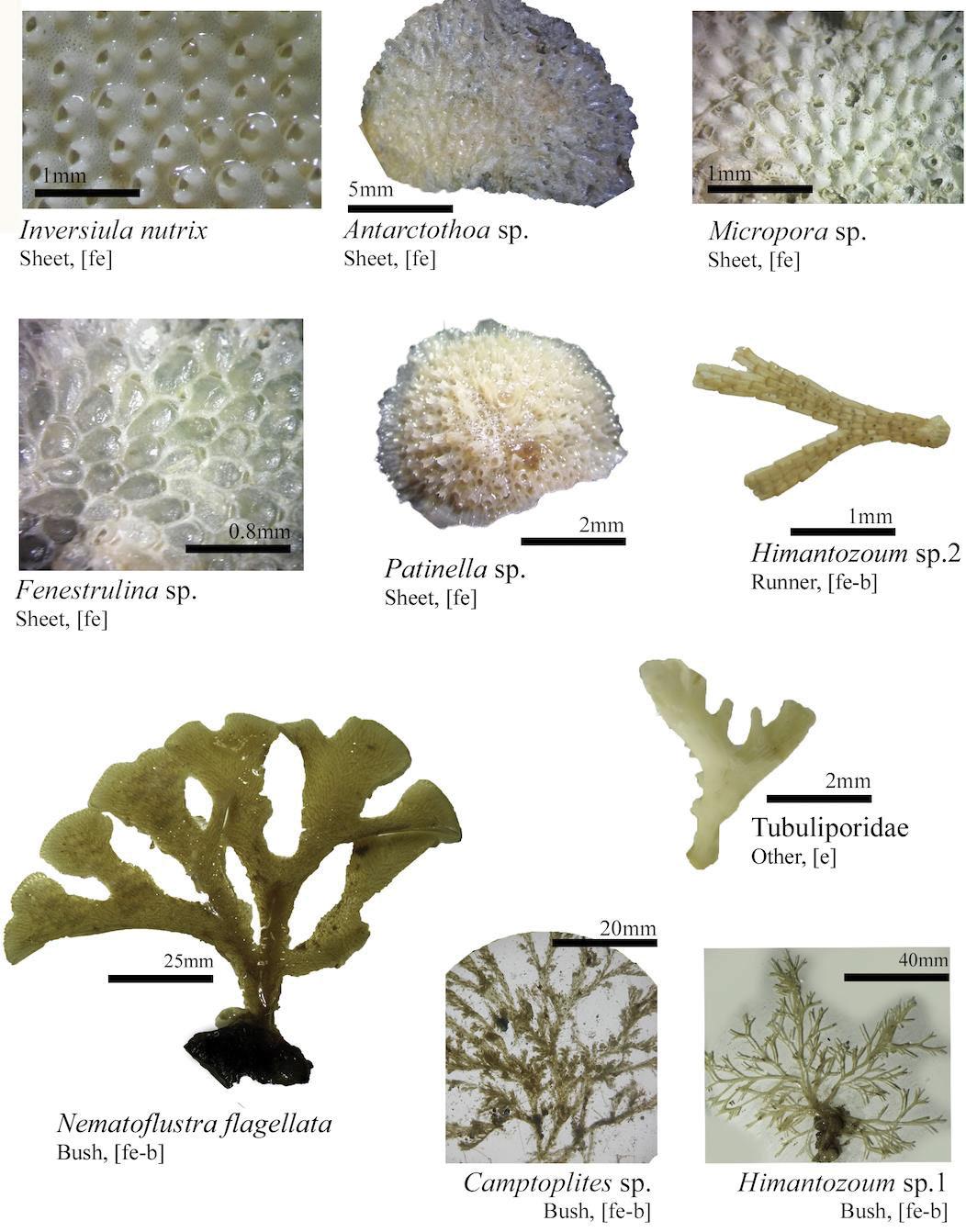
Appendix 4. Collection information of samples at the Universidad Cientifica del Sur.
References
Alurralde G., Fuentes V.L., MaggionI T., Movilla J., Olariaga A., Orejas C., Schloss R. and Tatián M. 2019. Role of suspension feeders in Antarctic pelagic-benthic coupling: Trophic ecology and potential carbon sinks under climate change. Marine Environmental Research 152: 104790. doi:10.1016/J.MARENVRES.2019.104790
Amini Z.Z., Adabi M.H., Burrett C.F. and Quilty P.G. 2004. Bryozoan distribution and growth form associations as a tool in environmental interpretation, Tasmania, Australia. Sedimentary Geology 167: 1–15. doi:10.1016/j.sedgeo.2004.01.010
Barnes D.K.A. 1995. Sublittoral epifaunal communities at Signy Island, Antarctica. II. Below the ice-foot zone. Marine Biology 121: 565–572. doi:10.1007/BF00349467
Barnes D.K.A. 2017. Polar zoobenthos blue carbon storage increases with sea ice losses, because across-shelf growth gains from longer algal blooms outweigh ice scour mortality in the shallows. Global Change Biology 23: 5083–5091. doi:10.1111/gcb.13772
Barnes D.K.A and Sands C.J. 2017. Functional group diversity is key to Southern Ocean benthic carbon pathways. PLoS ONE 12: e0179735. doi:10.1371/journal.pone.0179735
Barnes D.K.A and Souster T. 2011. Reduced survival of Antarctic benthos linked to climate induced iceberg scouring. Nature Climate Change 1: 365–368. doi:10.1038/nclimate1232
Baylón M., Hernández-Becerril D.U., Indacochea A. and Purca S. 2019. Variabilidad espaciotemporal del fitoplancton de la ensenada Mackellar, Bahía Almirantazgo, Isla Rey Jorge, Antártida, durante el verano austral 2012/2013. Revista de Biología Marina y Oceanografía 54: 151–165. doi: 10.22370/rbmo.2019.54.2.1809 https://doi.org/https://doi.org/10.22370/ rbmo.2019.54.2.1809
Boyer M., Matricardi G. and Pisano E. 1990. Zoarial forms in the development of a bryozoan community. Anales de Biología Murcia 16: 155–162.
Brey T. and Gerdes D. 1997. Is Antarctic benthic biomass really higher than elsewhere? Antarctic Science 9: 266–267. doi:10.1017/s0954102097000357
Campos L.S., Barboza C.A.M., Bassoi M., Bernardes M., Bromberg S., Corbisiert N., Fontes R.F. C., Gheller P.F., Hajdu E., Kawall H.G., Lange P.K., Lanna A.M., Lavrado H.P., Monteiro G. C.S., Montone R.C., Morales T., Moura R.B., Nakayama C.R., Oackes T., Paranhos R., Passos F.D., Petti M.A.V., Pellizariv H., Rezende C.E., Rodrigues M., Henrique Rosa L., Secchi E., Tenenbaum D.R. and Yoneshigue-Valentin Y. 2012. Environmental processes, biodiversity and changes in Admiralty Bay, King George Island, Antarctica. Adaptation and Evolution in Marine Environments 2: 127–156. doi:10.1007/978-3-642-27349-0_8
Chapin F.S., Zavaleta E.S., Eviner V.T., Naylor R.L., Vitousek P.M., Reynolds H.L., Hooper D.U., Lavorel S., Sala O.E., Hobbie S.E., Mack M.C. and Díaz S. 2000. Consequences of changing biodiversity. Nature 405: 234–242. doi:10.1038/35012241
Clarke A. 2008. Antarctic marine benthic diversity: patterns and processes. Journal of Experimental Marine Biology and Ecology 366: 48–55. doi:10.1016/j.jembe.2008.07.008
Clarke K.R. and Gorley R.N. 2006. PRIMER v6: User Manual/Tutorial. Plymouth, UK.
Clark G.F., Stark, J.S. and Johnston E.L. 2017. Tolerance rather than competition leads to spatial dominance of an Antarctic bryozoan. Journal of Experimental Marine Biology and Ecology 486: 222–229. doi:10.1016/j.jembe.2016.10.008
Connell J. and Keough M. 1985. Disturbance and patch dynamics of subtidal marine animals on hard substrata. In: Pickett S.T. and White P. (eds.) The ecology of natural disturbance and patch dynamics. Academic Press Inc.: 125–151.
Cook A.J., Holland P.R., Meredith M.P., Murray T., Luckman A. and Vaughan D.G. 2016. Ocean forcing of glacier retreat in the western Antarctic Peninsula. Science 353: 283–286. doi: 10.1126/science.aae00
De Bello F., Lavorel S., Díaz S., Harrington R., Cornelissen J.H.C., Bardgett R.D., Berg M.P., Cipriotti P., Feld C.K., Hering D., Martins Da Silva P., Potts S., Sandin L., Sousa J.P., Storkey J., Wardle D. and Harrison P.A. 2010. Towards an assessment of multiple ecosystem processes and services via functional traits. Biodiversity and Conservation 19: 2873–2893. doi:10.1007/ s10531-010-9850-9
Dayton P.K., Robilliard G.A., Paine R.T. and Dayton L.B. 1974. Biological accommodation in the benthic community at McMurdo Sound, Antarctica. Ecological Monographs 44: 105–128.
Dayton P K., Mordida B.J. and Bacon F. 1994. Polar marine communities. American Zoologist 34: 90–99.
Díaz S., Purvis A., Cornelissen J.H.C., Mace G.M., Donoghue M.J., Ewers R.M., Jordano P. and Pearse W.D. 2013. Functional traits, the phylogeny of function, and ecosystem service vulnerability. Ecology and Evolution 3: 2958–2975. doi:10.1002/ece3.601
Echeverría C.A. and Paiva P.C. 2006. Macrofaunal shallow benthic communities along a discontinuous annual cycle at Admiralty Bay, King George Island, Antarctica. Polar Biology 29: 263–269. doi:10.1007/s00300-005-0049-6
Echeverría C.A., Paiva P.C. and Alves V.C. 2005. Composition and biomass of shallow benthic megafauna during an annual cycle in Admiralty Bay, King George Island, Antarctica. Antarctic Science 17: 312–318. doi:10.1017/S0954102005002762
Folk R.L. 1954. The distinction between grain size and mineral composition in sedimentary-rock nomenclature. The Journal of Geology 62: 344–359.
Gilbert R., Nielsen N., Möller H., Desloges J.R. and Rasch M. 2002. Glacimarine sedimentation in Kangerdluk (Disko Fjord), West Greenland, in response to a surging glacier. Marine Geology 191: 1–18. doi:10.1016/S0025-3227(02)00543-1
Gili J.M., Coma R., Orejas C., López-González P.J. and Zabala M. 2001. Are Antarctic suspensionfeeding communities different from those elsewhere in the world? Polar Biology 24: 473–485. doi:10.1007/s003000100257
Gutt J .2001. On the direct impact of ice on marine benthic communities, a review. Ecological Studies in the Antarctic Sea Ice Zone: 157–168. doi:10.1007/s003000100262
Gutt J. 2007. Antarctic macro-zoobenthic communities: a review and an ecological classification. Antarctic Science 19: 165. doi:10.1017/S0954102007000247
Gutt J. and Schickan T. 1998. Epibiotic relationships in the Antarctic benthos. Antarctic Science 10: 398–405. doi:10.1017/S0954102098000480
Gutt J. and Starmans A. 1998. Structure and biodiversity of megabenthos in the Weddell and Lazarev Seas (Antarctica): Ecological role of physical parameters and biological interactions. Polar Biology 20: 229–247. doi:10.1007/s003000050300
Hageman S.J., Bone Y., Mcgowran B. and James N.P. 1997. Bryozoan colonial growth-forms as paleoenvironmental indicators: Evaluation of methodology. Palaios 12: 405. doi:10.2307/ 3515380
Hageman S.J., Bock P.E., Bone Y. and Mcgowran B. 1998. Analysis bryozoan growth habits: classification. SEPM Society for Sedimentary Geology Paleontological Society 72: 418–436. doi: 10.1017/S0022336000024161
Hayward P.J. 1995. Antarctic cheilostomatous bryozoa. Oxford University Press.
Jackson J.B.C. 1977. Competition on marine hard substrata: the adaptive significance of solitary and colonial strategies. The American Naturalist 111: 743–767
Jackson J.B.C. 1979. Morphological strategies of sessile animals. Biology and Systematic of Colonial Organisms: 499–555.
Jansen J., Hill N.A., Dunstan P.K., Mckinlay J., Sumner M.D., Post A.L., Eléaume M.P., Armand L. K., Warnock J.P., Galton-Fenzi B.K. and Johnson C.R. 2018. Abundance and richness of key Antarctic seafloor fauna correlates with modelled food availability. Nature Ecology & Evolution 2: 71–80. doi: 10.1038/s41559-017-0392-3
Kott P. 1989. Form and function in the Ascidiacea. Bulletin of Marine Science 45: 253–276.
286 Daniela C.S. Thorne, Bernabé Moreno, Aldo G. IndacocheaKukliński P. 2009. Ecology of stone-encrusting organisms in the Greenland Sea—a review. Polar Research 28: 222–237. doi:10.3402/polar.v28i2.6114
Kowalski D. 1985. Wind structure at the Arctowski Station Polish Polar Research 6: 391–403.
Krzemińska M. and Kukliński P. 2018. Biodiversity patterns of rock encrusting fauna from the shallow sublittoral of the Admiralty Bay. Marine Environmental Research 139: 169–181. doi:10.1016/j.marenvres.2018.03.016
Krzemińska M., Siciński J. and Kukliński P. 2018. Biodiversity and biogeographic affiliation of Bryozoa from King George Island (Antarctica). Systematics and Biodiversity 16: 576–586. doi:10.1080/14772000.2018.1457099
Lipski M. 1987. Variations of physical conditions, nutrients and chlorophyll a content in Admiralty Bay (King George Island, South Shetland islands, 1979). Polish Polar Research 8: 307–332.
Lipski I.M. and Rakusa-Suszczewski S. 1990. Early summer pattern of vertical distribution of chlorophyll a (Bransfield Strait Antarctica November 1986). Polskie Archiwum Hydrobiologii 37: 287–293.
Meredith M.P. and King J.C. 2005. Rapid climate change in the ocean west of the Antarctic Peninsula during the second half of the 20th century. Geophysical Research Letters 32: 1–5. doi:10.1029/2005GL024042
Momo F., Sahade R. and Tatián M. 2008. Benthic animal communities of Potter Cove (King George Island, Antarctica): Observed patterns and explanatory models. The Antarctic ecosystem of Potter Cove, King-George Island (Isla 25 Mayo). Reports on Polar and Marine Research: 1–411. doi:10.2312/BzPM_0571_2008
Monniot C. and Monniot F. 1983. Ascidies antarctiques et subantarctiques: morphologie et biogeographie. Muséum national d'Histoire Naturelle, Paris.
Monniot F., Dettai A., Eleaume M., Cruaud C. and Ameziane N. 2011. Antarctic Ascidians (Tunicata) of the French-Australian survey CEAMARC in Terre Adélie. Zootaxa 54: 1–54. doi:10.11646/zootaxa.2817.1.1
Morley S.A, Barnes D.K.A. and Dunn M.J. 2019. Predicting which species succeed in climateforced polar seas. Frontiers in Marine Science 5: 1–507. doi: 10.3389/fmars.2018.00507
Nelson C.S., Hyden F.M., Keane S.L., Leask S.L. and Gordon D.P. 1988. Application of bryozoan zoarial growth form studies in facies analysis of non-tropical carbonate deposits in New Zealand. Sedimentary Geology, 60: 301–322. doi: /10.1016/0037-0738(88)90126-1
Nelson M.L. 2009. Growth morphology and succession in a temperate marine fouling community. Master’s thesis, Humboldt State University.
Pabis K., Sicinski J. and Krymarys, M. 2011. Distribution patterns in the biomass of macrozoobenthic communities in Admiralty Bay (King George Island, South Shetlands, Antarctic). Polar Biology 34: 489–500. doi:10.1007/s00300-010-0903-z
Pabis K., Hara U., Presler P. and SicinskI J. 2014. Structure of bryozoan communities in an Antarctic glacial fjord (Admiralty Bay, South Shetlands). Polar Biology 37: 737–751. doi:10.1007/s00300-014-1474-1
Pęcherzewski K. 1980. Distribution and quantity of suspended matter in Admiralty Bay (King George Island, South Shetland Islands). Polish Polar Research 1: 75–82.
Pérez-Harguindeguy N., Díaz S., Garnier E., Lavorel S., Poorter H., Jaureguiberry P., Bret-Harte M. S., Cornwelf W.K., Craine J.M., Gurvich D.E., Urcelay E.J., Veneklaas E.J., Reich P.B., Poorter L., Wright I.J., Ray P., Enrico L., Pausas J.G., De Vos A.C., Buchmann N., Funes G., Quétier F., Hodgson J.G., Thompson K., Morgan H.D., Ter Steege H., Van Der Heijden A., Sack L., Blonder B., Poschlod P., Vaierentti M.V., Conti G., Staver A.C., Aquino S. and Cornelissen J.H.C. 2013. New handbook for standardised measurement of plant functional traits worldwide. Australian Journal of Botany 61: 167–234. doi:10.1071/BT12225
Primo C. and Vázquez E. 2007. Ascidians collected during the Spanish Antarctic expedition CIEMAR 99/00 in the Bransfield and Gerlache Straits. Journal of Natural History 41: 1775–1810. doi:10.1080/00222930701500126
Pruszak Z. 1980. Currents circulation in the waters of Admiralty Bay (region of Arctowski Station on King George Island). Polish Polar Research 1: 55–74.
Rakusa-Suszczewski S. 1995. Flow of matter in the Admiralty Bay area, King George Island, Maritime Antarctic. NIPR Symposium on Polar Biology, National Institute of Polar Research, Tokyo 8: 101–113.
Robakiewicz M. and Rakusa-Suszczweski S.1999. Application of 3D circulation model to Admiralty Bay, King George Island Antarctica. Polar Research 20: 43–58
Rückamp M., Braun M., Suckro S. and Blindow N. 2011. Observed glacial changes on the King George Island ice cap, Antarctica, in the last decade. Global and Planetary Change 79: 99–109. doi:10.1016/j.gloplacha.2011.06.009
Ryland J.S. and Warner G.F. 1986. Growth and form in modular animals: ideas on the size and arrangement of zooids. Philosophical Transactions Royal Society of London, Series B, 313: 53–76. doi:10.1098/rstb.1986.0025
Sahade R., Lagger C., Torre L., Momo F., Monien P., Schloss I., Barnes D.K.A., Servetto N., Tarantelli S., Tatián M., Zamboni N. and Abele, D. 2015. Climate change and glacier retreat drive shifts in an Antarctic benthic ecosystem. Science Advances 1: 1–8. doi:10.1126/ sciadv.1500050
Sanders C.J., Santos I.R., Patchineelam S.R., Schaefer C. and Silva-Filho E.V. 2010. Recent 137Cs deposition in sediments of Admiralty Bay, Antarctica. Journal of Environmental Radioactivity 101: 421–424. doi:10.1016/j.jenvrad.2010.02.006
Schopf T.J. 1969. Paleoecology of ectoprocts (Bryozoans). Journal of Paleontology 43: 234–244.
Siciński J., Jażdżewski K., De Broyer C., Presler P., Ligowski R., Nonato E.F., Corbisier T.N., Petti M.A.V., Brito T.A.S., Lavrado H.P., Błażewicz-Paszkowycz., Pabis K., Jażdżewska A. and Campos L.S. 2011. Admiralty Bay benthos diversity – A census of a complex polar ecosystem. Deep-Sea Research Part II: Topical Studies in Oceanography 58: 30–48. doi:10.1016/j.dsr2.2010.09.005
Smale D.A, Brown K.M., Barnes D.K.A., Fraser K.P.P. and Clarke A. 2008. Ice scour disturbance in Antarctic Waters. Science 321: 371. doi: 10.1126/science.1158
Smith A.M. 1995. Paleoenvironmental interpretation using bryozoans: a review. In: Bosence D. and Allison P. (eds.) Marine paleoenvironmental analysis from fossils. Geological Society, Special Publication, London 83: 231–243. doi:10.1144/GSL.SP.1995.083.01.11
Stach L.W. 1936. Correlation of zoarial form with habitat. The Journal of Geology 44: 60–65. doi:10.1086/624395
Tatián M., Mercuri G., Fuentes V.L., Antacli J.C., Stellfeldt A. and Sahade R. 2008a. Role of benthic filter feeders in pelagic-benthic coupling: assimilation, biodeposition and particle flux. The Antarctic ecosystem of Potter Cove, King-George Island (Isla 25 Mayo). Reports on Polar and Marine Research. Alfred-Wegener-Institut für Polar- und Meeresforschung, Bremerhaven: 118–127.
Tatián M., Milozzi A., Sahade R., Mercuri G. and Lagger C. 2008b. Contribution of different seston components to ascidian food in Potter Cove. The Antarctic ecosystem of Potter Cove, KingGeorge Island (Isla 25 Mayo). Reports on Polar and Marine Research. Alfred-WegenerInstitut für Polar- und Meeresforschung, Bremerhaven: 128–134.
Teixidó N., Garrabou J., Gutt J. and Arntz W.E. 2004. Recovery in Antarctic benthos after iceberg disturbance: Trends in benthic composition, abundance and growth forms. Marine Ecology Progress Series 278: 1–16. doi:10.3354/meps278001
Torre L., Servetto N., Eöry M.L., Momo F., Tatián M., Abele D. and Sahade R. 2012. Respiratory responses of three Antarctic ascidians and a sea pen to increased sediment concentrations. Polar Biology 35: 1743–1748. doi:10.1007/s00300-012-1208-1
Torre L., Abele D., Lagger C., Momo F. and Sahade R. 2014. When shape matters: Strategies of different Antarctic ascidians morphotypes to deal with sedimentation. Marine Environmental Research 99: 179–187. doi:10.1016/j.marenvres.2014.05.014
288 Daniela C.S. Thorne, Bernabé Moreno, Aldo G. IndacocheaWahl M. and Mark O. 1999. The predominantly facultative nature of epibiosis: experimental and observational evidence. Marine Ecology Progress Series 187: 59–66. doi:10.3354/ meps187059
Wildish D. and Kristmanson D. 1997. Benthic suspension feeders and flow. Cambridge University Press, Cambridge.
Włodarska-Kowalczuk M., Pearson T.H. and Kendall M.A. 2005. Benthic response to chronic natural physical disturbance by glacial sedimentation in an Arctic fjord. Marine Ecology Progress Series 303: 31–41. doi: 10.3354/meps303031
Ziegler A.F., Smith C.R., Edwards K.F. and Vernet M. 2017. Glacial dropstones: Islands enhancing seafloor species richness of benthic megafauna in West Antarctic Peninsula fjords. Marine Ecology Progress Series 583: 1–14. doi:10.3354/meps12363
Zielinski K. 1990. Bottom macroalgae of the Admiralty Bay (King George Island, South Shetlands, Antarctica). Polish Polar Research 11: 95–131.
Zwolska I. and Janecki T. 1999. Weather and hydrological conditions in the region of Admiralty Bay (King George Island, South Shetlands, Antarctica) in 1998. Polish Polar Studies. XXVI Polar Symposium, June 1999, Lublin: 329–338.
Received 24 December 2021
Accepted 27 May 2022