Abstract
The phenomenon of repeated liquefaction of sand has been widely observed in field and laboratory testing, and the liquefaction responses of sand during repeated liquefaction events and the shear behavior of sand after initial liquefaction have been excessively tested. However, the dynamic and static properties of sand, which play a vital role in the assessment of the long-term safety and stability of geotechnical systems, under repeated liquefaction have not been studied systematically. As such, a series of undrained triaxial dynamic and compression tests are conducted in this study to investigate the liquefaction properties, dynamic modulus, undrained shear behavior, and seismic wave characteristics of sand during repeated liquefaction events. The test results indicate that the undrained shear strength increases with the number of liquefaction events, whereas the liquefaction resistance, static stability, dynamic modulus, and shear wave velocity decrease after initial liquefaction and then increase after subsequent liquefaction events. Further, a novel index based on the shear wave velocity and primary wave velocity is formulated to assess the liquefaction history of sand: this index of post-liquefaction sand tends to be much lower than that of unliquefied sand.















Similar content being viewed by others
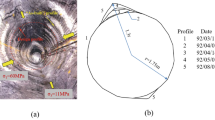
Data availability
All data supporting the results are included in this manuscript.
References
Andrade JE, Ramos AM, Lizcano A (2013) Criterion for flow liquefaction instability. Acta Geotech 8(5):525–535. https://doi.org/10.1007/s11440-013-0223-x
Asadi MS, Orense RP, Asadi MB, Pender MJ (2019) Post-liquefaction behavior of natural pumiceous sands. Soil Dyn Earthq Eng 118:65–74. https://doi.org/10.1016/j.soildyn.2018.12.009
Ashour M, Norris G, Nguyen T (2009) Assessment of the undrained response of sands under limited and complete liquefaction. J Geotech Geoenviron Eng 135(11):1772–1776. https://doi.org/10.1061/(ASCE)GT.1943-5606.0000114
ASTM Committee D-18 on Soil and Rock (2020) Standard test method for consolidated undrained triaxial compression test for cohesive soils (ASTM D4767-11). ASTM International, West Conshohocken
Casagrande A (1940) Characteristics of cohesionless soils affecting the stability of earth fills. Contributions to Soils Mechanics, 1925–1940. Boston Society of Civil Engineers, Boston, pp 257–276
Chaudhary SK, Kuwano J, Hashimoto S, Hayano Y, Nakamura Y (2002) Effects of initial fabric and shearing direction on cyclic deformation characteristics of sand. Soils Found 42(1):147–157. https://doi.org/10.3208/sandf.42.1147
Chen G, Li QY, Li DQ, Wu ZY, Liu Y (2019) Main frequency band of blast vibration signal based on wavelet packet transform. Appl Math Model 74:569–585. https://doi.org/10.1016/j.apm.2019.05.005
Chen G, Wang FT, Li DQ, Liu Y (2020) Dyadic wavelet analysis of bender element signals in determining shear wave velocity. Can Geotech J 57(12):2027–2030. https://doi.org/10.1139/cgj-2019-0167
Chen GX, Liang K, Zhao K, Yang J (2022) Shear modulus and damping ratio of saturated coral sand under generalized cyclic loadings. Géotechnique. https://doi.org/10.1680/jgeot.21.00181
Chen GX, Zhao DF, Chen WY, Juang CH (2019) Excess pore-water pressure generation in cyclic undrained testing. J Geotech Geoenviron Eng 145(7):04019022. https://doi.org/10.1061/(ASCE)GT.1943-5606.0002057
Chu J, Wanatowski D, Loke WL, Leong WK (2015) Pre-failure instability of sand under dilatancy rate controlled conditions. Soils Found 55(2):414–424. https://doi.org/10.1016/j.sandf.2015.02.015
Cubrinovski M, Henderson D, Bradley B (2012) Liquefaction impacts in residential areas in the 2010–2011 Christchurch earthquakes. In: Garevski M (ed) Earthquakes and health monitoring of civil structures. Springer, Dordrecht, pp 1–35
El-Sekelly W, Dobry R, Abdoun T, Steidl JH (2016) Centrifuge modeling of the effect of preshaking on the liquefaction resistance of silty sand deposits. J Geotech Geoenviron Eng 142(6):04016012. https://doi.org/10.1061/(ASCE)GT.1943-5606.0001430
Enomoto T (2019) Liquefaction and post-liquefaction properties of sand-silt mixtures and undisturbed silty sands. Soils Found 59(6):2311–2323. https://doi.org/10.1016/j.sandf.2019.09.005
Enomoto T (2020) Multiple-liquefaction behaviour of sands containing fines. Jpn Geotech J 15(2):281–293. https://doi.org/10.3208/jgs.15.281
Fardad Amini P, Huang D, Wang G, Jin F (2021) Effects of strain history and induced anisotropy on reliquefaction resistance of toyoura sand. J Geotech Geoenviron Eng. https://doi.org/10.1061/(ASCE)GT.1943-5606.0002588
Finn WL, Bransby PL, Pickering DJ (1970) Effect of strain history on liquefaction of sand. J Soil Mech Found Div 96(6):1917–1934. https://doi.org/10.1061/JSFEAQ.0001478
Gong WP, Tien YM, Juang CH, Martin JR, Zhang J (2016) Calibration of empirical models considering model fidelity and model robustness—Focusing on predictions of liquefaction-induced settlements. Eng Geol 203:168–177. https://doi.org/10.1016/j.enggeo.2015.11.003
Gu XQ, Zuo KL, Tessari A, Gao GY (2021) Effect of saturation on the characteristics of P-wave and S-wave propagation in nearly saturated soils using bender elements. Soil Dynam Earthq Eng 145:106742. https://doi.org/10.1016/j.soildyn.2021.106742
Hyde AF, Higuchi T, Yasuhara K (2007) Postcyclic recompression, stiffness, and consolidated cyclic strength of silt. J Geotech Geoenviron Eng 133(4):416–423. https://doi.org/10.1061/(ASCE)1090-0241(2007)133:4(416)
Ishibashi I, Sherif MA, Tsuchiya C (1977) Pore-pressure rise mechanism and soil liquefaction. Soils Found 17(2):17–27. https://doi.org/10.3208/sandf1972.17.2_17
Ishihara K, Okada S (1982) Effects of large preshearing on cyclic behavior of sand. Soils Found 22(3):109–125. https://doi.org/10.3208/sandf1972.22.3_109
Iwai H, Ni XQ, Ye B, Nishimura N, Zhang F (2020) A new evaluation index for reliquefaction resistance of Toyoura sand. Soil Dyn Earthq Eng 136:106206. https://doi.org/10.1016/j.soildyn.2020.106206
Juang CH, Chen CJ, Jiang T (2001) Probabilistic framework for liquefaction potential by shear wave velocity. J Geotech Geoenviron Eng 127(8):670–678. https://doi.org/10.1061/(ASCE)1090-0241(2001)127:8(670)
Kasama K, Zen K, Iwataki K (2006) Undrained shear strength of cement-treated soils. Soils Found 46(2):221–232. https://doi.org/10.3208/sandf.46.221
Khoshnevisan S, Juang CH, Zhou YG, Gong WP (2015) Probabilistic assessment of liquefaction-induced lateral spreads using CPT—Focusing on the 2010–2011 Canterbury earthquake sequence. Eng Geol 192:113–128. https://doi.org/10.1016/j.enggeo.2015.04.001
Kiyota T, Koseki J, Sato T, Kuwano R (2009) Aging effects on small strain shear moduli and liquefaction properties of in-situ frozen and reconstituted sandy soils. Soils Found 49(2):259–274. https://doi.org/10.3208/sandf.49.259
Kiyota T, Maekawa Y, Wu C (2019) Using in-situ and laboratory-measured shear wave velocities to evaluate the influence of soil fabric on in-situ liquefaction resistance. Soil Dyn Earthq Eng 117:164–173. https://doi.org/10.1016/j.soildyn.2018.11.016
Kumar J, Madhusudhan BN (2010) A note on the measurement of travel times using bender and extender elements. Soil Dyn Earthq Eng 30(7):630–634. https://doi.org/10.1016/j.soildyn.2010.02.003
Kuribayashi E, Tatsuoka F (1975) Brief review of liquefaction during earthquakes in Japan. Soils Found 15(4):81–92. https://doi.org/10.3208/sandf1972.15.4_81
Lade PV, Yamamuro JA (2011) Evaluation of static liquefaction potential of silty sand slopes. Can Geotech J 48(2):247–264. https://doi.org/10.1139/T10-063
Lombardi D, Bhattacharya S, Hyodo M, Kaneko T (2014) Undrained behaviour of two silica sands and practical implications for modelling SSI in liquefiable soils. Soil Dyn Earthq Eng 66:293–304. https://doi.org/10.1016/j.soildyn.2014.07.010
Matasović N, Vucetic M (1993) Cyclic characterization of liquefiable sands 119(11):1805–1822
Ministry of Water Resources of the People’s Republic of China (2019) Standard for geotechnical testing method (GB/T 50123-2019). China Planning Press, Beijing
Morimoto T, Aoyagi Y, Koseki J (2019) Effects of induced anisotropy on multiple liquefaction properties of sand with initial static shear. Soils Found 59(5):1148–1159. https://doi.org/10.1016/j.sandf.2019.03.015
Nakagawa K, Soga K, Mitchell JK (1997) Observation of Biot compressional wave of the second kind in granular soils. Géotechnique 47(1):133–147. https://doi.org/10.1680/geot.51.1.85.39355
Nelson F, Okamura M (2019) Influence of strain histories on liquefaction resistance of sand. Soils Found 59(5):1481–1495. https://doi.org/10.1016/j.sandf.2019.06.011
Ni XQ, Zhang Z, Ye B, Zhang S (2022) Unique relation between pore water pressure generated at the first loading cycle and liquefaction resistance. Eng Geol 296:106476. https://doi.org/10.1016/j.enggeo.2021.106476
Oda M, Kawamoto K, Suzuki K, Fujimori H, Sato M (2001) Microstructural interpretation on reliquefaction of saturated granular soils under cyclic loading. J Geotech Geoenviron Eng 127(5):416–423. https://doi.org/10.1061/(ASCE)1090-0241(2001)127:5(416)
Quigley MC, Bastin S, Bradley BA (2013) Recurrent liquefaction in Christchurch, New Zealand, during the Canterbury earthquake sequence. Geology 41(4):419–422. https://doi.org/10.1130/G33944.1
Rasouli H, Fatahi B, Nimbalkar S (2022) Re-liquefaction resistance of lightly cemented sands. Can Geotech J. Just-IN. https://doi.org/10.1139/cgj-2021-0343
Rees SD, Jones CR, Snelling K (2015) Adaptive control implementation for the dynamic cyclic testing of soil specimens. In: Winter MG, Smith DM, Eldred PJL, Toll DG (eds) Geotechnical engineering for infrastructure and development. ICE Publishing, London, pp 3317–3322
Rouholamin M, Bhattacharya S, Orense RP (2017) Effect of initial relative density on the post-liquefaction behaviour of sand. Soil Dyn Earthq Eng 97:25–36. https://doi.org/10.1016/j.soildyn.2017.02.007
Sawyer DE, DeVore JR (2015) Elevated shear strength of sediments on active margins: Evidence for seismic strengthening. Geophys Res Lett 42(23):10–216. https://doi.org/10.1002/2015GL066603
Seed HB (1979) Considerations in the earthquake-resistant design of earth and rockfill dams. Géotechnique 29(3):215–263. https://doi.org/10.1680/geot.1979.29.3.215
Seed HB (1987) Design problems in soil liquefaction. J Geotech Eng 113(8):827–845. https://doi.org/10.1061/(ASCE)0733-9410(1987)113:8(827)
Seed HB, Lee KL (1966) Liquefaction of saturated sands during cyclic loading. J Soil Mech Found Div 92(6):105–134. https://doi.org/10.1061/JSFEAQ.0000913
Shen MF, Chen QS, Zhang J, Gong WP, Juang CH (2016) Predicting liquefaction probability based on shear wave velocity: an update. Bull Eng Geol Env 75(3):1199–1214. https://doi.org/10.1007/s10064-016-0880-8
Skempton AW (1954) The pore-pressure coefficients A and B. Géotechnique 4(4):143–147. https://doi.org/10.1680/geot.1954.4.4.143
Song BH, Sun YF, Song YP, Dong LF, Du X, Zhou QK, Zhao XL (2020) Post-liquefaction re-compaction effect on the cyclic behavior of natural marine silty soil in the Yellow River delta. Ocean Eng 195:106753. https://doi.org/10.1016/j.oceaneng.2019.106753
Terzaghi K, Peck RB, Mesri G (1996) Soil mechanics in engineering practice. Wiley, New York
Thomann TG, Hryciw RD (1990) Laboratory measurement of small strain shear modulus under K0 conditions. Geotech Test J 13(2):97–105
Toyota H, Takada S (2017) Variation of liquefaction strength induced by monotonic and cyclic loading histories. J Geotech Geoenviron Eng 143(4):04016120. https://doi.org/10.1061/(ASCE)GT.1943-5606.0001634
Toyota H, Takada S (2022) Mechanical properties of cementitious sand and sand with small cyclic shear strain to assess aging effects on liquefaction. Acta Geotech 17:2825–2840. https://doi.org/10.1007/s11440-021-01368-6
Verdugo R (2016) Experimental and conceptual evidence about the limitations of shear wave velocity to predict liquefaction. Soil Dyn Earthq Eng 91:160–174. https://doi.org/10.1016/j.soildyn.2016.09.046
Wahyudi S, Koseki J, Sato T, Chiaro G (2016) Multiple-liquefaction behavior of sand in cyclic simple stacked-ring shear tests. Int J Geomech 16(5):C4015001. https://doi.org/10.1061/(ASCE)GM.1943-5622.0000596
Wakamatsu K (2012) Recurrence of liquefaction at the same site induced by the 2011 Great East Japan earthquake compared with previous earthquakes. In: Proceeding of the 15th World Conference on Earthquake Engineering. https://doi.org/10.5610/jaee.12.5_69
Wang G, Wei JT (2016) Microstructure evolution of granular soils in cyclic mobility and post-liquefaction process. Granular Matter 18(3):51. https://doi.org/10.1007/s10035-016-0621-5
Wang SY, Luna R, Onyejekwe S (2015) Postliquefaction behavior of low-plasticity silt at various degrees of reconsolidation. Soil Dyn Earthq Eng 75:259–264. https://doi.org/10.1016/j.soildyn.2015.04.014
Wu C, Kiyota T (2019) Effects of specimen density and initial cyclic loading history on correlation between shear wave velocity and liquefaction resistance of Toyoura sand. Soils Found 59(6):2324–2330. https://doi.org/10.1016/j.sandf.2019.03.018
Wu TW, Suzuki Y, Carlton B, Harbitz C, Kopf A (2021) Effect of prior small to moderate seismic events on monotonic undrained shear strength of sand. Soil Dyn Earthq Eng 141:106465. https://doi.org/10.1016/j.soildyn.2020.106465
Xie XL, Ye B, Zhao T, Feng XQ, Zhang F (2021) Changes in sand mesostructure under repeated seismic liquefaction events during centrifuge tests. Soil Dyn Earthq Eng 150:106940. https://doi.org/10.1016/j.soildyn.2021.106940
Yamada S, Takamori T, Sato K (2010) Effects on reliquefaction resistance produced by changes in anisotropy during liquefaction. Soils Found 50(1):9–25. https://doi.org/10.3208/sandf.50.9
Yang J, Liang LB, Chen Y (2022) Instability and liquefaction flow slide of granular soils: the role of initial shear stress. Acta Geotech 17:65–79. https://doi.org/10.1007/s11440-021-01200-1
Yasuda S, Harada K, Ishikawa K, Kanemaru Y (2012) Characteristics of liquefaction in Tokyo Bay area by the 2011 Great East Japan earthquake. Soils Found 52(5):793–810. https://doi.org/10.1016/j.sandf.2012.11.004
Yasuda S, Tohno I (1988) Sites of reliquefaction caused by the 1983 Nihonkai-Chubu earthquake. Soils Found 28(2):61–72. https://doi.org/10.3208/sandf1972.28.2_61
Ye B, Hu HL, Bao XH, Lu P (2018) Reliquefaction behavior of sand and its mesoscopic mechanism. Soil Dyn Earthq Eng 114:12–21. https://doi.org/10.1016/j.soildyn.2018.06.024
Zhu ZH, Zhang F, Peng QY, Chabot B, Dupla JC, Canou J, Cumunel G, Foerster E (2021) Development of an auto compensation system in cyclic triaxial apparatus for liquefaction analysis. Soil Dyn Earthq Eng 144:106707. https://doi.org/10.1016/j.soildyn.2021.106707
Acknowledgements
The financial support provided by the National Natural Science Foundation of China (Grant No. 41977242), the Major Program of the National Natural Science Foundation of China (Grant No. 42090055), and the Outstanding Youth Foundation of Hubei Province, China (Grant No. 2022CFA102) is acknowledged.
Author information
Authors and Affiliations
Corresponding author
Ethics declarations
Competing interest
The authors declare no competing interests.
Additional information
Publisher's Note
Springer Nature remains neutral with regard to jurisdictional claims in published maps and institutional affiliations.
Rights and permissions
Springer Nature or its licensor (e.g. a society or other partner) holds exclusive rights to this article under a publishing agreement with the author(s) or other rightsholder(s); author self-archiving of the accepted manuscript version of this article is solely governed by the terms of such publishing agreement and applicable law.
About this article
Cite this article
Zhang, S., Gong, W., Wu, Q. et al. Experimental studies on dynamic and static properties of sand during repeated liquefaction events. Acta Geotech. 18, 5231–5249 (2023). https://doi.org/10.1007/s11440-023-01819-2
Received:
Accepted:
Published:
Issue Date:
DOI: https://doi.org/10.1007/s11440-023-01819-2