Synthesis and biological evaluation of novel benzothiazole derivatives as potential anticancer and antiinflammatory agents
- 1Department of Pharmacy, Wenzhou Hospital of Integrated Traditional Chinese and Western Medicine, Wenzhou, China
- 2School of Pharmaceutical Science, Wenzhou Medical University, Wenzhou, China
- 3Department of Pharmacy, The First People’s Hospital of Taizhou, Taizhou, China
- 4Scientific Research Center, Wenzhou Medical University, Wenzhou, China
Introduction: Cancer, a significant global health concern, necessitates innovative treatments. The pivotal role of chronic inflammation in cancer development underscores the urgency for novel therapeutic strategies. Benzothiazole derivatives exhibit promise due to their distinctive structures and broad spectrum of biological effects. This study aims to explore new anti-tumor small molecule drugs that simultaneously anti-inflammatory and anticancer based on the advantages of benzothiazole frameworks.
Methods: The compounds were characterized by nuclear magnetic resonance (NMR), liquid chromatograph-mass spectrometer (LC-MS) and high performance liquid chromatography (HPLC) for structure as well as purity and other related physicochemical properties. The effects of the compounds on the proliferation of human epidermoid carcinoma cell line (A431) and human non-small cell lung cancer cell lines (A549, H1299) were evaluated by MTT method. The effect of compounds on the expression levels of inflammatory factors IL-6 and TNF-α in mouse monocyte macrophages (RAW264.7) was assessed using enzyme-linked immunosorbent assay (ELISA). The effect of compounds on apoptosis and cell cycle of A431 and A549 cells was evaluated by flow cytometry. The effect of compounds on A431 and A549 cell migration was evaluated by scratch wound healing assay. The effect of compounds on protein expression levels in A431 and A549 cells was assessed by Western Blot assay. The physicochemical parameters, pharmacokinetic properties, toxicity and drug similarity of the active compound were predicted using Swiss ADME and admetSAR web servers.
Results: Twenty-five novel benzothiazole compounds were designed and synthesized, with their structures confirmed through spectrogram verification. The active compound 6-chloro-N-(4-nitrobenzyl) benzo[d] thiazol-2-amine (compound B7) was screened through a series of bioactivity assessments, which significantly inhibited the proliferation of A431, A549 and H1299 cancer cells, decreased the activity of IL-6 and TNF-α, and hindered cell migration. In addition, at concentrations of 1, 2, and 4 μM, B7 exhibited apoptosis-promoting and cell cycle-arresting effects similar to those of the lead compound 7-chloro-N-(2, 6-dichlorophenyl) benzo[d] thiazole-2-amine (compound 4i). Western blot analysis confirmed that B7 inhibited both AKT and ERK signaling pathways in A431 and A549 cells. The prediction results of ADMET indicated that B7 had good drug properties.
Discussion: This study has innovatively developed a series of benzothiazole derivatives, with a focus on compound B7 due to its notable dual anticancer and anti-inflammatory activities. B7 stands out for its ability to significantly reduce cancer cell proliferation in A431, A549, and H1299 cell lines and lower the levels of inflammatory cytokines IL-6 and TNF-α. These results position B7B7 as a promising candidate for dual-action cancer therapy. The study’s mechanistic exploration, highlighting B7’s simultaneous inhibition of the AKT and ERK pathways, offers a novel strategy for addressing both the survival mechanisms of tumor cells and the inflammatory milieu facilitating cancer progression.
1 Introduction
Cancer is a formidable disease that poses a significant threat to human health and has been a persistent challenge in the global public health domain (Ferlay et al., 2015; Fitzmaurice et al., 2015; Bray et al., 2021; Sung et al., 2021). Despite notable advances in the medical field, cancer treatment still confronts various limitations and challenges (Chang et al., 2021; Craig et al., 2021; Ghanbar and Suresh, 2024). Drug therapy stands out as a widely employed approach in cancer treatment (Wu et al., 2022), where the effectiveness and side effects of drugs are pivotal factors influencing treatment outcomes (Dinić et al., 2020; Steinbrueck et al., 2020; Li et al., 2024b). Consequently, the quest for novel anticancer drugs has emerged as a central focus of contemporary research.
Chronic inflammation is increasingly recognized as a critical factor in cancer development. This complex interplay facilitates tumor initiation and progression. IL-6 and TNF-α are key inflammatory cytokines known for their roles in immune regulation, inflammation, and cell proliferation. Their involvement in tumor development and progression is a subject of ongoing research (Hastir et al., 2020; Park et al., 2020; Cowan et al., 2022). These research underscores the multifaceted roles of IL-6 and TNF-α in tumor development. These cytokines not only contribute to the pro-inflammatory tumor microenvironment but also interact with various signaling pathways and immune cells, influencing both local tumor growth and systemic inflammatory responses. Their impact on tumor development and progression highlights their potential as targets for therapeutic intervention in cancer treatment (Meza et al., 2021).
Over the past two decades, benzothiazole compounds have attracted considerable research attention due to their distinctive structure and diverse biological activities, including anti-tumor (Kamal et al., 2010; Kamal et al., 2011; El-Helby et al., 2019; Makowska et al., 2019; Mokesch et al., 2020), anti-inflammatory (Lee et al., 2011; Kumar and Singh, 2021), neuroprotective (Choi et al., 2007), antibacterial (Al-Tel et al., 2011; Racané et al., 2020), and antiparasitic (Awadh, 2023) activities, etc. In recent years, extensive research has focused on modifying the benzothiazole nucleus to enhance its anti-tumor activities. Among the modified structures, benzothiazole derivatives exhibiting specificity towards various anti-tumor receptors have consistently emerged. This includes compounds interacting with receptor tyrosine kinases such as C-Met and EGFR (Noolvi et al., 2012), those influencing the PI3K/Akt/mTOR pathway (D'Angelo et al., 2011), and those exhibiting antimicrobial properties, as illustrated in Scheme 1. Additionally, two compounds have garnered special attention for their excellent anti-tumor effects: PMX610 [2-(3,4-dimethoxyphenyl)-5-fluorobenzothiazole] (Mortimer et al., 2006) and compound 4i (Noolvi et al., 2012) (Figure 1). PMX610 has been reported to possess potent and selective in vitro anti-tumor properties in human cancer cell lines, particularly against non-small cell lung, colon, and breast cancer lines from 60 human cancer cell line screen. Compound 4i demonstrated promising anticancer activity against the non-small cell lung cancer cell line HOP-92, with substitutions at the 2,6-positions displayed significant anticancer potential in initial cytotoxicity screening across three human cancer cell lines. These findings underscore the significance of benzothiazole as a core structure in drug synthesis, with modifications to the benzothiazole nucleus enhancing its anticancer activity. The importance of benzothiazole and its derivatives in anticancer research is progressively becoming apparent.
Based on the above analysis, this study is to explore new anti-tumor small molecule drugs that simultaneously anti-inflammatory and anticancer based on the advantages of benzothiazole frameworks. Based on compounds PMX610 and 4i as lead compounds, we attempted to design A, B, C, and D four series of compounds. Series A of benzothiazoles has been designed by incorporating diverse substituted phenyl groups at the 2-phenyl position of 2-phenyl benzothiazole, drawing inspiration from existing literature and undergoing further optimization (Kumbhare et al., 2012). Series B and C of benzothiazoles have been meticulously designed by introducing an additional carbon atom or carbonyl groups between two rings, compared to compound 4i. Series D of benzothiazoles has been designed as a further replacement of Series B (Figure 1). The compounds designed in this study exhibit a distinct departure in structure from classical small molecule anti-tumor compounds. This structural variation enables the exploration of the relationship between activity and structure, with the intention of leading to the derivation of more advantageous molecular architectures. We anticipate that these compounds will emerge as promising candidates for anticancer prospects, providing scientific evidence for future anticancer drug development and introducing new possibilities to clinical treatment.
2 Materials and methods
2.1 Chemical reagents and instruments
The reagents and alkoxyamine intermediates employed in chemical synthesis were procured directly from reputable suppliers such as Macklin, Aladdin, and Energy Chemical. All solvents utilized possessed high chemical purity and underwent no further treatment. These included petroleum ether (PE), ethyl acetate (EA), dichloromethane (DCM), dimethyl sulfoxide (DMSO), and 1,4-Dioxane. The progression of the reaction was tracked through analytical thin-layer chromatography (TLC) utilizing a silica gel GF254 plate (Qingdao Haiyang Chemical Plant, China), with spot observations made under UV light at 254 nm or 365 nm. Column chromatography was conducted using silica gel (90–150 μm; Qingdao Ocean Chemical Co., Ltd.). The melting points were determined using the XT-4 micromelting point apparatus without correction. 1H NMR and 13C NMR spectra were acquired using a Bruker 400/600 MHz Avance NMR spectrometer, employing CDCl3 or DMSO-d6 as solvents. Mass spectra were generated using an ACQUITY I-Class UPLC and a XEVO TQD triple quadrupole Mass Spectrometer (Waters, USA). HPLC (Agilent 1260, USA) assessed the purity of the compounds, all of which exhibited purity levels surpassing 96%. Elemental analysis for C, H, and N was conducted via an elemental analyzer (Flash EA1112, United States) and found to be within ± 0.3% of the theoretical values.
2.2 Synthesis method of 2-phenol-benzothiazole
A substantial quantity of 2-phenol-benzothiazole must be synthesized. Benzothiazole (1 mmol), 2-hydroxyiodobenzene (1 mmol), and K2CO3 (2 mmol) as a binding agent were combined in 2 mL of DMSO. The resulting mixture is magnetically stirred at 120°C for 6 h, with the reaction progress monitored via TLC. Upon completion, the reaction mixture was cooled to room temperature, and the solvent is evaporated under reduced pressure. Following this, water (8 mL) and an equivalent volume of ethyl acetate were introduced for extraction through multiple iterations of small-volume extractions (3 times). The aqueous layer was discarded, and the organic layer was desiccated with Na2SO4. Subsequent to drying, the organic layer underwent evaporation under reduced pressure, and the resulting residue was subjected to column chromatography. Intermediate 1 was extracted through column chromatography using a mobile phase of petroleum ether: ethyl acetate = 2:1. The yield is 50%, and the product was dried in an oven for subsequent use.
2.3 General synthesis method of A1-A8
To attain the desired A-type final product, 2-phenol-benzothiazole (1 mmol) was combined with various brominated compounds (1.2 mmol) and K2CO3 (2 mmol) as a binding agent, within of acetonitrile (6 mL). The resulting mixture underwent stirring at room temperature for a duration of 3 h. Throughout the reaction, the solution underwent a noticeable transition from a pale-yellow suspension to a white suspension, with progress monitored through TLC. Following the completion of the reaction, solvent evaporation was conducted under reduced pressure. Subsequently, water (6 mL) was introduced to dissolve K2CO3, followed by the addition of an equivalent volume of ethyl acetate for extraction. This extraction process was iteratively performed in three steps, employing small aliquots. The aqueous layer was discarded, and the organic layers were consolidated and desiccated using solid Na2SO4. Following desiccation, the organic layer underwent evaporation under reduced pressure to eliminate excess ethyl acetate. The resultant product was subjected to recrystallization using petroleum ether. Yield determination took place after the drying process, and subsequent characterization of the product was performed.
2.4 General synthesis method of B1-B8
In a 100 mL three-necked flask, either 2-amino-6-chlorobenzothiazole (3.4 mmol) or 2-amino-5-fluorobenzothiazole (3.4 mmol), along with 2 mmol of K2CO3 as a binding agent, were introduced. Subsequently, acetonitrile (6 mL) was added, and the mixture underwent sonication. Concurrently, benzyl bromide (1 mmol) was dissolved in acetonitrile (15 mL). The benzyl bromide solution was then meticulously introduced dropwise into the three-necked flask using a constant pressure dropping funnel, with a controlled rate of 1 drop every 5 s. The ensuing reaction mixture underwent reflux for a duration of 6–7 h, with the progression monitored via TLC. Upon completion, the reaction mixture was gradually cooled to room temperature, and the solvent was then evaporated under reduced pressure. Extraction ensued by introducing saturated NaCl (8 mL) and an equivalent volume of ethyl acetate, followed by multiple iterations for thorough extraction. The resultant organic layer was separated, and solid Na2SO4 was incorporated for desiccation. The desiccated organic layer underwent further evaporation under reduced pressure, and the resultant residue underwent purification through column chromatography. The elution solvent comprised a blend of petroleum ether and ethyl acetate in a 9:1 ratio. Fractions collected during chromatography were concentrated under reduced pressure, yielding the final solid product. The overall yield was determined post-drying.
2.5 General synthesis method of C1-C7
In the reaction involving 2-amino-6-chlorobenzothiazole (1 mmol) or 2-amino-5-fluorobenzothiazole (1 mmol), acyl chloride (4 mmol), and triethylamine (1 mL) as a binding agent, dioxane (10 mL) was employed as the solvent. The reaction mixture underwent reflux for 3–4 h, with TLC used to monitor the reaction progress. After completion, the reaction mixture was cooled to room temperature, and saturated Na2CO3, in a molar ratio equivalent to the acyl chloride, was added. The resulting mixture was stirred overnight. The precipitated product was filtered, followed by drying. The yield was determined post-drying.
2.6 General synthesis method of D1-D2
In a 100 mL three-necked flask, either B6 (3.4 mmol) or B8 (3.4 mmol), along with K2CO3 (2 mmol) as a binding agent, was introduced. Following this, acetonitrile (6 mL) was added, and the resulting mixture underwent sonication. Concurrently, benzyl bromide (1 mmol) was dissolved in acetonitrile (15 mL). The benzyl bromide solution was subsequently added dropwise to the three-necked flask using a constant-pressure dropping funnel at a rate of 1 drop every 5 s. The reaction mixture underwent reflux for 6–7 h, with TLC monitoring the reaction progress. Upon completion, the reaction mixture was cooled to room temperature, and the solvent was evaporated under reduced pressure. Extraction was carried out by adding saturated NaCl (8 mL) and an equal volume of ethyl acetate in multiple iterations. The organic layer was separated, and solid Na2SO4 was added for drying. The dried organic layer was evaporated under reduced pressure, and the resulting residue underwent purification via column chromatography. The elution solvent, a mixture of petroleum ether and ethyl acetate in a 15:1 ratio, was employed. The collected fractions were concentrated under reduced pressure to obtain the solid product, with yield determination following the drying process.
2.7 Structural characterization data of target compounds
Ethyl 2-(2-(benzo[d]thiazol-2-yl)phenoxy)acetate (A1) White solid; Yield/%: 78%; Mp/°C: 73.4∼75.4; ESI-MS [M + H]+: 314.6; 1H NMR (600 MHz, CDCl3) δ ppm: 8.556 (d, J = 7.8 Hz, 1H, Ph-H), 8.096 (d, J = 8.2 Hz, 1H, Ph-H), 7.935 (d, J = 8.0 Hz, 1H, Ph-H), 7.491 (t, J = 7.68 Hz, 1H, Ph-H), 7.439 (t, J = 7.86 Hz, 1H, Ph-H), 7.377 (t, J = 7.62 Hz, 1H, Ph-H), 7.199 (t, J = 7.56 Hz, 1H, Ph-H), 6.969 (d, J = 8.28 Hz, 1H, Ph-H), 4.866 (s, 2H, -OCH2), 4.299–4.335 (m, 2H, CH2), 1.316 (t, J = 7.14 Hz, 3H, CH3). 13C NMR (150 MHz, CDCl3) δ ppm: 168.2 (-C=O), 163.1 (thiazole-C), 155.6 (benzothiazole-C), 136.3 (benzothiazole-C), 131.9 (Ph-C), 130.2 (Ph-C), 126.1 (Ph-C), 124.9 (Ph-C), 122.9 (Ph-C), 122.4 (Ph-C), 121.4 (Ph-C), 112.6 (Ph-C), 66.1 (-OCH2COOCH2CH3), 61.7(-OCH2COOCH2CH3), 14.3(-OCH2COOCH2CH3). Anal. Calcd for C17H15NO3S: C, 65.16%; H, 4.82%; N, 4.47%; Found: C, 65.18%; H, 4.83%; N, 4.46%.
2-(2-((2-nitrobenzyl)oxy)phenyl)benzo[d]thiazole (A2) White solid; Yield/%: 81%; Mp/°C: 115.6∼117.6; ESI-MS [M + H]+: 363.2; 1H NMR (600 MHz, CDCl3) δ ppm: 8.502 (d, J = 7.8 Hz, 1H, Ph-H), 8.231 (d, J = 8.22 Hz, 1H, Ph-H), 8.115 (d, J = 8.16 Hz, 1H, Ph-H), 7.971 (d, J = 7.86 Hz, 1H, Ph-H), 7.905 (d, J = 7.92 Hz, 1H, Ph-H), 7.687 (t, J = 7.5 Hz, 1H, Ph-H), 7.496–7.547 (m, 2H, Ph-H), 7.373–7.440 (m, 2H, Ph-H), 7.182 (t, J = 7.5 Hz, 1H, Ph-H), 7.036 (d, J = 8.34 Hz, 1H, Ph-H), 5.792 (s, 2H, -OCH2). 13C NMR (150 MHz, CDCl3) δ ppm: 163.1 (thiazole-C), 155.8 (benzothiazole-C), 152.3 (Ph-C), 147.1 (Ph-C), 136.0 (Ph-C), 134.3 (Ph-C), 133.3 (benzothiazole-C), 132.0 (Ph-C), 130.4 (Ph-C), 129.1 (Ph-C), 128.8 (Ph-C), 126.2 (Ph-C), 125.3 (Ph-C), 125.0 (Ph-C), 123.0 (Ph-C), 122.2 (Ph-C), 121.4 (Ph-C), 113.4 (Ph-C), 68.2(-OCH2-). Anal. Calcd for C20H14N2O3S: C, 66.29%; H, 3.89%; N, 7.73%; Found: C, 66.31%; H, 3.88%; N, 7.74%.
2-(3-(2-(benzo[d]thiazol-2-yl)phenoxy)propyl)isoindoline-1,3-dione (A3) Pale yellow solid; Yield/%: 67%; Mp/°C: 141.6∼143.6; ESI-MS[M + H]+: 415.1; 1H NMR (600 MHz, CDCl3) δ ppm: 8.476 (dd, J = 7.8, 1.8 Hz, 1H, Ph-H), 8.033 (d, J = 8.4 Hz, 1H, Ph-H), 7.978 (d, J = 8.4 Hz, 1H, Ph-H), 7.721–7.735 (m, 2H, Ph-H), 7.585–7.599 (m, 2H Ph-H), 7.421–7.486 (m, 2H, Ph-H), 7.374 (t, J = 7.8 Hz, 1H, Ph-H), 7.115 (t, J = 7.68 Hz, 1H, Ph-H), 7.033 (d, J = 8.4 Hz, 1H, Ph-H), 4.319 (t, J = 5.88 Hz, 2H, -OCH2), 4.080 (t, J = 6.84 Hz, 2H, -CH2), 2.418–2.460 (m, 2H, -CH2). 13C NMR (150 MHz, CDCl3) δ ppm: 168.5 (-C=O), 163.3 (thiazole-C), 156.5 (benzothiazole-C), 135.9 (benzothiazole-C), 133.9 (Ph-C), 132.1 (Ph-C), 129.8 (Ph-C), 126.1 (Ph-C), 124.8 (Ph-C), 123.1 (Ph-C), 122.6 (Ph-C), 121.6 (Ph-C), 112.2 (Ph-C), 67.3 (-OCH2CH2CH2-), 36.1 (-OCH2CH2CH2-), 28.8 (-OCH2CH2CH2-). Anal. Calcd for C24H18N2O3S: C, 69.55%; H, 4.38%; N, 6.76%; Found: C,69.51%; H, 4.37%; N, 6.75%.
2-(2-((4-nitrobenzyl)oxy)phenyl)benzo[d]thiazole (A4) White solid; Yield/%: 70%; Mp/°C: 135.5∼137.5; ESI-MS [M + H]+: 363.2; 1H NMR (600 MHz, CDCl3) δ ppm: 8.535 (dd, J = 8.16, 2.4 Hz, 1H, Ph-H), 8.287 (d, J = 8.58 Hz, 2H, Ph-H), 8.101 (d, J = 8.16 Hz, 1H, Ph-H), 7.897 (d, J = 7.98 Hz, 1H, Ph-H), 7.733 (d, J = 8.58 Hz, 2H, Ph-H), 7.503 (t, J = 7.86 Hz, 1H, Ph-H), 7.370–7.454 (m, 2H, Ph-H), 7.184 (t, J = 7.62 Hz, 1H, Ph-H), 7.057 (d, J = 8.28 Hz, 1H, Ph-H), 5.426 (s, 2H,-OCH2). 13C NMR (150 MHz, CDCl3) δ ppm: 162.9 (thiazole-C), 155.8 (benzothiazole-C), 148.0 (Ph-C), 143.4 (Ph-C), 135.8 (Ph-C), 132.1 (benzothiazole-C), 130.4 (Ph-C), 128.3 (Ph-C), 126.3 (Ph-C), 125.1 (Ph-C), 124.1 (Ph-C), 122.9 (Ph-C), 122.2 (Ph-C), 121.4 (Ph-C), 113.0 (Ph-C), 69.9 (-OCH2-). Anal. Calcd for C20H14N2O3S: C, 66.29%; H, 3.89%; N, 7.73%; Found: C, 66.30%; H, 3.90%; N, 7.75%.
2-(2-((3-chlorobenzyl)oxy)phenyl)benzo[d]thiazol (A5) White solid; Yield/%: 65%; Mp/°C: 53.4∼55.4; ESI-MS [M + H]+: 352.1; 1H NMR (600 MHz, CDCl3) δ ppm: 8.546 (dd, J = 7.68, 1.38 Hz, 1H, Ph-H), 8.098 (d, J = 8.10 Hz, 1H, Ph-H), 7.899 (d, J = 7.92 Hz, 1H, Ph-H), 7.567 (s, 1H, Ph-H), 7.491 (t, J = 8.04 Hz, 1H, Ph-H), 7.347–7.429 (m, 5H, Ph-H), 7.158 (t, J = 7.74 Hz, 1H, Ph-H), 7.069 (d, J = 8.34 Hz, 1H, Ph-H), 5.311 (s, 2H, -OCH2). 13C NMR (150 MHz, CDCl3) δ ppm: 156.1 (thiazole-C), 138.3 (Ph-C) (benzothiazole-C), 136.1 (Ph-C), 134.7 (benzothiazole-C), 131.9 (Ph-C), 130.1 (Ph-C), 128.5 (Ph-C), 127.9 (Ph-C), 126.1 (Ph-C), 125.9 (Ph-C), 124.8 (Ph-C), 122.9 (Ph-C), 121.8 (Ph-C), 121.4 (Ph-C), 113.0 (Ph-C), 70.4 (-OCH2-). Anal. Calcd for C20H14ClNOS: C, 68.27%; H, 4.01%; N, 3.98%; Found: C, 68.28%; H, 4.02%; N, 3.99%.
2-(2-((4-methylbenzyl)oxy)phenyl)benzo[d]thiazole (A6) White solid; Yield/% 77%; Mp/°C: 57.3∼59.3; ESI-MS [M + H]+: 332.0; 1H NMR (600 MHz, CDCl3) δ ppm: 8.555 (dd, J = 7.98, 1.32 Hz, 1H, Ph-H), 8.082 (d, J = 8.16Hz, 1H, Ph-H), 7.883 (d, J = 7.92Hz, 1H, Ph-H), 7.402–7.489 (m, 4H, Ph-H), 7.351 (t, J = 7.38 Hz, 1H, Ph-H), 7.225 (d, J = 7.8 Hz, 2H, Ph-H), 7.124 (dd, J = 7.56, 5.58 Hz, 2H, Ph-H), 5.309 (s, 2H, -OCH2), 2.390 (s, 3H, -CH3). 13C NMR (150 MHz, CDCl3) δ ppm: 163.2 (thiazole-C), 156.3 (Ph-C), (benzothiazole-C), 138.0 (Ph-C), 136.1 (Ph-C), 133.0 (benzothiazole-C), 131.7 (Ph-C), 129.3 (Ph-C), 127.9 (Ph-C), 125.9 (Ph-C), 124.5 (Ph-C), 122.7 (Ph-C), 121.3 (Ph-C), 121.3 (Ph-C), 113.0 (Ph-C), 70.9 (-OCH2-), 21.2 (-CH3). Anal. Calcd for C21H17NOS: C, 76.10%; H, 5.17%; N, 4.23%; Found: C, 76.08%; H, 5.16%; N, 4.22%.
2-(2-(benzyloxy)phenyl)benzo[d]thiazole (A7) Gray solid; Yield/%: 70%; Mp/°C: 89.7∼91.7; ESI-MS [M + H]+: 318.4; 1H NMR (600 MHz, CDCl3) δ ppm: 8.557 (dd, J = 7.98, 1.50 Hz, 1H, Ph-H), 8.089 (d, J = 8.22 Hz, 1H, Ph-H), 7.879 (d, J = 7.98 Hz, 1H, Ph-H), 7.546 (d, J = 7.32 Hz, 2H, Ph-H), 7.481 (t, J = 8.04 Hz, 1H, Ph-H), 7.342–7.435 (m, 5H, Ph-H), 7.102–7.151 (m, 2H, Ph-H), 5.352(s, 2H, -OCH2). 13C NMR (150 MHz, CDCl3) δ ppm: 163.3 (thiazole-C), 156.3 (benzothiazole-C), 136.0 (Ph-C), 131.9 (Ph-C), (benzothiazole-C), 129.9 (Ph-C), 128.6 (Ph-C), 128.3 (Ph-C), 127.8 (Ph-C), 126.0 (Ph-C), 124.7 (Ph-C), 122.6 (Ph-C), 121.5 (Ph-C), 121.3 (Ph-C), 113.0 (Ph-C), 71.0 (-OCH2-). Anal. Calcd for C20H15NOS: C, 75.68%; H, 4.76%; N, 4.41%; Found: C, 75.70%; H, 4.77%; N, 4.42%.
4-((2-(benzo[d]thiazol-2-yl)phenoxy)methyl)benzonitrile (A8) Gray solid; Yield/%: 66%; Mp/°C: 131.8∼133.8; ESI-MS [M + H]+: 343.1; 1H NMR (600 MHz, CDCl3) δ ppm: 8.546 (d, J = 7.8 Hz, 1H, Ph-H), 8.098 (d, J = 8.16 Hz, 1H, Ph-H), 7.894 (d, J = 7.92 Hz, 1H, Ph-H), 7.717 (d, J = 8.1Hz, 2H, Ph-H), 7.660 (d, J = 8.04 Hz, 2H, Ph-H), 7.501 (t, J = 7.74 Hz, 1H, Ph-H), 7.431 (t, J = 8.1 Hz,1H, Ph-H), 7.381 (t, J = 7.68Hz, 1H, Ph-H), 7.175 (t, J = 7.56 Hz, 1H, Ph-H), 7.041 (d, J = 8.34 Hz, 1H, Ph-H), 5.380 (s, 2H, -OCH2). 13C NMR (150 MHz, CDCl3) δ ppm: 155.7 (thiazole-C), 141.4 (benzothiazole-C), 132.5 (benzothiazole-C), 131.9 (Ph-C), 130.2 (Ph-C), 128.1 (Ph-C), 126.2 (Ph-C), 124.9 (Ph-C), 122.8 (Ph-C), 122.0 (Ph-C), 121.3 (Ph-C), 118.5 (-CN), 112.8 (Ph-C), 112.2 (Ph-C), 70.1 (-OCH2-). Anal. Calcd for C21H14N2OS: C, 73.66%; H, 4.12%; N, 8.18%; Found: C, 73.64%; H, 4.11%; N, 8.17%.
6-fluoro-N-phenethylbenzo[d]thiazol-2-amine (B1) White solid; Yield/%: 86%; Mp/°C: 132.4∼134.6; ESI-MS [M + H]+: 273.1; 1H NMR (400 MHz, DMSO-d6) δ ppm: 12.85 (s, 1H, -NH), 8.07–8.04 (m, 1H, Ph-H), 7.85–7.79 (m, 3H, Ph-H), 7.60 (dd, J = 9.9, 2.5 Hz, 1H, Ph-H), 7.25–7.13 (m, 3H, Ph-H), 3.89 (s, 2H, -CH2), 3.87 (s, 2H, -CH2). 13C NMR (101 MHz, DMSO-d6) δ ppm: 165.6 (thiazole-C), 163.0 (Ph-C), 161.9 (Ph-C), 160.6 (Ph-C), 153.2 (Ph-C), 148.9 (benzothiazole-C), 123.4 (C, d, JC−C−C−F = 10.10 Hz), 122.9 (Ph-C), 112.2 (Ph-C), 111.9 (Ph-C), 111.6 (C, d, JC−C−C−C−F = 4.04 Hz), 106.8 (C, d, JC−C−F = 24.24 Hz), 56.2 (-CH2CH2-), 56.1 (-CH2CH2-). Anal. Calcd for C15H13FN2S: C, 66.15%; H, 4.81%; N, 10.29%; Found: C, 66.16%; H, 4.82%; N, 10.30%.
N-(2,6-dichlorobenzyl)-6-fluorobenzo[d]thiazol-2-amine (B2) Gray solid; Yield/%: 77%; Mp/°C: 156.8∼158.2; ESI-MS [M + H]+: 327.0; 1H NMR (400 MHz, DMSO-d6) δ ppm: 8.57 (d, J = 4.5 Hz, 1H, Ph-H), 7.70 (dd, J = 8.6, 5.6 Hz, 1H, Ph-H), 7.55 (d, J = 8.1 Hz, 2H, Ph-H), 7.43 (dd, J = 8.7, 7.5 Hz, 1H, Ph-H), 7.28 (dd, J = 10.4, 2.5 Hz, 1H, Ph-H), 6.99–6.85 (m, 1H, Ph-H), 4.83 (s, 2H, -CH2). 13C NMR (101 MHz, DMSO-d6) δ ppm: 168.1 (thiazole-C), 163.0 (Ph-C), 160.6 (benzothiazole-C), 136.2 (Ph-C), 132.9 (Ph-C), 131.2 (Ph-C), 129.1 (Ph-C), 126.0 (benzothiazole-C), 122.5 (C, d, JC−C−C−F = 10.1 Hz), 109.1(C, d, JC−C−F = 24.24 Hz), 105.1(C, d, JC−C−F = 24.25 Hz), 44.1 (-CH2-). Anal. Calcd for C14H9Cl2FN2S: C, 51.39%; H, 2.77%; N, 8.56%; Found: C, 51.37%; H, 2.76%; N, 8.55%.
N-(3,4-dimethoxybenzyl)-6-fluorobenzo[d]thiazol-2-amine (B3) Gray solid; Yield/%: 79%; Mp/°C: 135.5∼137.4; ESI-MS [M + H]+: 319.1; 1H NMR (400 MHz, DMSO-d6) δ(ppm): 8.56 (s, 1H, -NH), 7.43 (dd, J = 8.3, 5.5 Hz, 1H, Ph-H), 7.11 (s, 1H, Ph-H), 6.99–6.75 (m, 4H, Ph-H), 5.08 (s, 2H, -CH2). 3.73 (d, J = 5.1 Hz, 6H, -OCH3). 13C NMR (101 MHz, DMSO-d6) δ ppm: 163.0 (thiazole-C), 160.6 (Ph-C), 160.0 (Ph-C), 149.2 (benzothiazole-C), 148.5 (Ph-C), 141.9 (Ph-C), 141.8 (Ph-C), 129.2 (benzothiazole-C), 123.1 (C, d, JC−C−C−F = 9.09 Hz), 119.8 (Ph-C), 117.9 (Ph-C), 112.1 (C, d, JC−C−F = 18.18 Hz), 108.1 (C, d, JC−C−F = 23.23 Hz), 98.6 (Ph-C), 98.3 (Ph-C), 55.9 (-OCH3), 45.1 (-CH2-). Anal. Calcd for C16H15FN2O2S: C, 60.36%; H, 4.75%; N, 8.80%; Found: C, 60.38%; H, 4.76%; N, 8.81%.
6-fluoro-N-(4-nitrobenzyl)benzo[d]thiazol-2-amine (B4) White solid; Yield/%: 65%; Mp/°C: 142.5∼144.7; ESI-MS [M + H]+: 304.1; 1H NMR (400 MHz, DMSO-d6) δ ppm: 8.86 (t, J = 5.9 Hz, 1H, -NH), 8.27–8.21 (m, 2H, Ph-H), 7.70 (dd, J = 8.6, 5.6 Hz, 1H, Ph-H), 7.66–7.59 (m, 2H, Ph-H), 7.20 (dd, J = 10.5, 2.5 Hz, 1H, Ph-H), 6.91 (td, J = 9.3, 2.6 Hz, 1H, Ph-H), 4.76 (d, J = 5.9 Hz, 2H, -CH2). 13C NMR (101 MHz, DMSO-d6) δ ppm: 168.8 (thiazole-C), 153.9 (C, d, JC−C−C−F = 12.12 Hz), 147.2 (C, d, JC−C−F = 40.40 Hz), 128.6 (Ph-C), 127.5 (Ph-C), 126.4 (benzothiazole-C), 124.1 (Ph-C), 122.4 (C, d, JC−C−F = 10.10 Hz), 109.1 (Ph-C), 105.5 (Ph-C), 46.9 (-CH2-). Anal. Calcd for C14H10FN3O2S: C, 55.44%; H, 3.32%; N, 13.85%; Found: C, 55.47%; H, 3.33%; N, 13.86%.
6-chloro-N-(3-fluorobenzyl)benzo[d]thiazol-2-amine (B5) White solid; Yield/%: 45%; Mp/°C: 145.6∼147.6; ESI-MS [M + H]+: 293.5; 1H NMR (600 MHz, CDCl3) δ ppm:7.545 (d, J = 2.1 Hz, 1H, Ph-H),7.429 (d, J = 8.64 Hz, 1H, Ph-H), 7.103–7.246 (m, 5H, Ph-H), 5.149 (s, 1H, -NH), 4.646 (s, 2H, -OCH2). 13C NMR (150 MHz, CDCl3) δ ppm: 130.4 (benzothiazole-C), 130.4 (Ph-C), 127.0 (Ph-C), 126.5 (Ph-C), 123.1 (Ph-C), 123.1 (Ph-C), 120.5 (Ph-C), 119.8 (Ph-C), 114.9 (Ph-C), 114.8 (Ph-C), 114.6 (Ph-C), 114.4 (Ph-C), 48.6 (-CH2-). Anal. Calcd for C14H10ClFN2S: C, 57.44%; H, 3.44%; N, 9.57%; Found: C, 57.48%; H, 3.45%; N, 9.58%.
6-chloro-N-(3,5-dimethoxybenzyl)benzo[d]thiazol-2-amine (B6) Pale yellow solid; Yield/%: 58%; Mp/°C: 101.3∼103.3; ESI-MS [M + H]+: 335.1; 1H NMR (600 MHz, CDCl3) δ ppm: 7.539 (d, J = 1.98 Hz, 1H, Ph-H), 7.427 (d, J = 8.58 Hz, 1H, Ph-H), 7.236 (s, 1H, Ph-H), 6.342–6.528 (m, 3H, Ph-H), 5.083 (s, 1H, -NH), 4.557(s, 2H, -CH2), 3.746 (s, 6H, -OCH3). 13C NMR (150 MHz, CDCl3) δ ppm: 167.5 (thiazole-C), 161.3 (Ph-C),151.0 (benzothiazole-C), 139.6 (Ph-C), 131.8 (benzothiazole-C), 126.5 (Ph-C), 121.5 (Ph-C), 120.6 (Ph-C), 119.7 (Ph-C), 110.7 (Ph-C), 107.0 (Ph-C), 107.0 (Ph-C), 105.7 (Ph-C), 99.8 (Ph-C), 99.1 (Ph-C), 55.5 (-CH3), 49.5 (-CH2-). Anal. Calcd for C16H15ClN2O2S: C, 57.40%; H, 4.52%; N, 8.37%; Found: C, 57.36%; H, 4.51%; N, 8.36%.
6-chloro-N-(4-nitrobenzyl)benzo[d]thiazol-2-amine (B7) Pale yellow solid; Yield/%: 31%; Mp/°C: 148.4∼150.8; ESI-MS [M + H]+: 320.4; 1H NMR (600 MHz, CDCl3) δ ppm: 8.412 (d, J = 8.4 Hz, 1H, Ph-H), 8.216 (d, J = 8.4 Hz, 2H, Ph-H), 8.199 (s, 1H, Ph-H), 8.145 (d, J = 8.4 Hz, 1H, Ph-H), 7.451 (d, J = 8.4 Hz, 2H, Ph-H), 4.850 (s, 2H, -CH2), 3.077 (s, 1H, -NH). 13C NMR (150 MHz, CDCl3) δ ppm: 167.5 (thiazole-C), 158.7 (benzothiazole-C),151.1 (Ph-C), 129.6 (Ph-C), 129.5 (benzothiazole-C), 126.6 (Ph-C), 121.6 (Ph-C), 115.8 (Ph-C), 110.5 (Ph-C), 48.7 (-CH2-). Anal. Calcd for C14H10ClN3O2S: C, 52.59%; H, 3.15%; N, 13.14%; Found: C, 52.61%; H, 3.14%; N, 13.13%.
N-benzyl-6-chlorobenzo[d]thiazol-2-amine (B8) Pale yellow solid; Yield/%: 50%; Mp/°C: 123.5∼125.5; ESI-MS [M + H]+: 275.1; 1H NMR (600 MHz, CDCl3) δ ppm: 7.540 (d, J = 2.04 Hz, 1H, Ph-H), 7.357–7.394 (m, 3H, Ph-H), 7.305–7.332 (m, 3H, Ph-H), 7.243 (d, J = 2.04 Hz, 1H, Ph-H), 5.160 (s, 1H, -NH), 4.631(s, 2H, -CH2). 13C NMR (125 MHz, DMSO-d6) δ ppm: 166.9 (thiazole-C), 151.2 (benzothiazole-C), 138.5 (Ph-C), 131.9 (benzothiazole-C), 128.6 (Ph-C), 128.3 (Ph-C), 127.3 (Ph-C), 127.1 (Ph-C), 126.8 (Ph-C), 125.7 (Ph-C), 124.7 (Ph-C), 120.5 (Ph-C), 118.9 (Ph-C), 47.2 (-CH2-). Anal. Calcd for C14H11ClN2S: C, 61.20%; H, 4.04%; N, 10.20%; Found: C, 61.17%; H, 4.03%; N, 10.19%.
N-(6-fluorobenzo[d]thiazol-2-yl)benzamide (C1) Gray solid; Yield/%: 45%; Mp/°C: >300; ESI-MS [M + H]+: 273.1; 1H NMR (400 MHz, DMSO-d6) δ ppm: 12.99 (s, 1H, -CONH), 8.21–8.11 (m, 2H, Ph-H), 8.06 (dd, J = 8.7, 5.5 Hz, 1H, Ph-H), 7.68 (t, J = 7.4 Hz, 1H, Ph-H), 7.65–7.53 (m, 3H, Ph-H), 7.23 (td, J = 9.1, 2.4 Hz, 1H, Ph-H). 13C NMR (101 MHz, DMSO-d6) δ 166.4 (thiazole-C), 163.0 (Ph-C), 161.6 (Ph-C), 160.6 (Ph-C), 133.4 (Ph-C), 132.1 (Ph-C), 129.1 (Ph-C), 128.8 (Ph-C), 127.8 (benzothiazole-C), 123.5 (C, d, JC−C−C−F = 10.10 Hz), 112.2 (C, d, JC−C−F = 24.24 Hz), 107.0 (C, d, JC−C−F = 24.24 Hz). Anal. Calcd for C14H9FN2OS: C, 61.75%; H, 3.33%; N, 10.29%; Found: C, 61.73%; H, 3.32%; N, 10.28%.
2,6-dichloro-N-(6-fluorobenzo[d]thiazol-2-yl) benzamide (C2) White solid; Yield/%: 51%; Mp/°C: >300; ESI-MS [M + H]+: 341.0; 1H NMR (400 MHz, DMSO-d6) δ ppm: 13.32 (s, 1H, -CONH), 8.09 (dd, J = 8.8, 5.5 Hz, 1H, Ph-H), 7.73–7.48 (m, 4H, Ph-H), 7.26 (td, J = 9.1, 2.5 Hz, 1H, Ph-H). 13C NMR (101 MHz, DMSO-d6) δ ppm: 163.8 (thiazole-C), 163.1 (Ph-C), 160.7 (Ph-C), 160.1 (Ph-C), 150.0 (C, d, JC−C−C−F = 12.12 Hz), 134.6 (Ph-C), 132.8 (Ph-C), 131.6 (Ph-C), 128.8 (benzothiazole-C), 127.8 (Ph-C), 123.7 (C, d, JC−C−C−F = 10.10 Hz), 112.7 (C, d, JC−C−F = 24.24 Hz), 107.6 (C, d, JC−C−F = 24.24 Hz). Anal. Calcd for C14H7Cl2FN2OS: C, 49.29%; H, 2.07%; N, 8.21%; Found: C, 49.31%; H, 2.08%; N, 8.22%.
N-(6-fluorobenzo[d]thiazol-2-yl)-3,4-dimethoxybenzamide (C3) White solid; Yield/%: 46%; Mp/°C: >300; ESI-MS [M + H]+: 333.1; 1H NMR (400 MHz, DMSO-d6) δ ppm: 12.86 (s, 1H, -CONH), 8.05 (dd, J = 8.7, 5.5 Hz, 1H, Ph-H), 7.93–7.74 (m, 2H, Ph-H), 7.60 (dd, J = 10.0, 2.2 Hz, 1H, Ph-H), 7.22 (td, J = 9.1, 2.4 Hz, 1H, Ph-H), 7.14 (d, J = 8.5 Hz, 1H, Ph-H), 3.88 (d, J = 7.1 Hz, 6H, -OCH3). 13C NMR (101 MHz, DMSO-d6) δ ppm: 165.6 (thiazole-C), 163.0 (Ph-C), 161.9 (Ph-C), 160.6 (Ph-C), 153.2 (Ph-C), 150.1 (benzothiazole-C), 148.8 (Ph-C), 127.8 (Ph-C), 123.7 (C, d, JC−C−C−F = 55.55 Hz), 122.8 (Ph-C), 111.8 (C, d, JC−C−F = 32.32 Hz), 111.6, 106.9 (C, d, JC−C−F = 25.25 Hz), 56.2 (-OCH3). Anal. Calcd for C16H13FN2O3S: C, 57.82%; H, 3.94%; N, 8.43%; Found: C, 57.79%; H, 3.93%; N, 8.42%.
N-(6-fluorobenzo[d]thiazol-2-yl)-4-nitrobenzamide (C4) White solid; Yield/%: 60%; Mp/°C:187.7∼189.4; ESI-MS [M + H]+: 319.1; 1H NMR (400 MHz, CF3COOD) δ ppm: δ 8.61 (dd, J = 63.9, 8.5 Hz, 4H, Ph-H), 8.21 (dd, J = 9.2, 4.4 Hz, 1H, Ph-H), 7.89 (d, J = 7.7 Hz, 1H, Ph-H), 7.64 (t, J = 8.9 Hz, 1H, Ph-H). 13C NMR (101 MHz, CF3COOD) δ ppm: 165.44 (thiazole-C), 151.2 (benzothiazole-C), 136.0 (C, d, JC−C−C−F = 13.13 Hz), 134.8 (Ph-C), 129.8 (benzothiazole-C), 124.4 (Ph-C), 121.1 (Ph-C), 118.6 (Ph-C), 116.7 (C, d, JC−C−F = 25.25 Hz), 115.8 (Ph-C), 113.0 (Ph-C), 110.18 (Ph-C), 103.3 (C, d, JC−C−F = 28.28 Hz). Anal. Calcd for C14H8FN3O3S: C, 53.00%; H, 2.54%; N, 13.24%; Found: C, 53.03%; H, 2.55%; N, 13.22%.
N-(6-chlorobenzo[d]thiazol-2-yl)-4-methoxybenzamide (C5) Gray solid; Yield/%: 70%; Mp/°C: >300, ESI-MS [M + H]+: 319.1; 1H NMR (600 MHz, CDCl3) δ ppm: 10.081 (s, 1H, -NH), 7.947 (d, J = 8.3 Hz, 2H, Ph-H), 7.813 (s, 1H, Ph-H), 7.376 (d, J = 8.6 Hz, 1H, Ph-H), 7.291 (d, J = 8.6 Hz, 1H, Ph-H), 3.862 (s, 3H, -OCH3). 13C NMR (150 MHz, DMSO-d6) δ ppm: 166.1 (thiazole-C), 163.3 (-C=O), 160.7 (Ph-C), 147.8 (benzothiazole-C), 133.7 (benzothiazole-C), 130.9 (Ph-C), 127.9 (Ph-C), 126.8 (Ph-C), 124.4 (Ph-C), 121.8 (Ph-C), 121.7 (Ph-C), 114.4 (Ph-C), 56.0 (-OCH3). Anal. Calcd for C15H11ClN2O2S: C, 56.52%; H, 3.48%; N, 8.79%; Found: C, 56.59%; H, 3.47%; N, 8.78%.
N-(6-chlorobenzo[d]thiazol-2-yl)benzamide (C6) Gray solid; Yield/%: 82%; Mp/°C: >300; ESI-MS [M + H]+: 289.1; 1H NMR (600 MHz, CDCl3) δ ppm: 11.011 (s, 1H, -NH), 7.985 (d, J = 7.86 Hz, 2H, Ph-H), 7.819 (s, 1H, Ph-H), 7.610 (t, J = 7.1 Hz, 2H, Ph-H), 7.481 (d, J = 7.5 Hz, 2H, Ph-H), 7.273 (s, 1H, Ph-H). 13C NMR (150 MHz, DMSO-d6) δ ppm: 166.9 (thiazole-C), 160.6 (-C=O), 147.7 (benzothiazole-C), 133.7 (Ph-C), 133.3 (benzothiazole-C), 132.5 (Ph-C), 129.1 (Ph-C), 128.7 (Ph-C), 128.0 (Ph-C), 126.9 (Ph-C), 121.9 (Ph-C), 121.7 (Ph-C). Anal. Calcd for C14H9ClN2OS: C, 58.24%; H, 3.14%; N, 9.70%; Found: C, 58.20%; H, 3.13%; N, 9.71%.
N-(6-chlorobenzo[d]thiazol-2-yl)-2-fluorobenzamide (C7) White solid; Yield/%: 77%; Mp/°C: >300; ESI-MS [M + H]+: 307.0; 1H NMR (600 MHz, CDCl3) δ ppm: 11.755 (s, 1H, -NH), 9.562 (s, 1H, Ph-H), 9.444 (d, J = 8.58 Hz, 1H, Ph-H), 9.350–9.385 (m, 1H, Ph-H), 9.105–9.155 (m, 2H, Ph-H), 9.006 (s, 1H, Ph-H), 8.971 (s, 1H, Ph-H). 13C NMR (1500 MHz, DMSO-d6) δ ppm: 166.0 (thiazole-C), 165.9 (-C=O), 164.2(benzothiazole-C), 133.4(benzothiazole-C), 131.7 (Ph-C), 131.6 (Ph-C), 128.2 (Ph-C), 127.0 (Ph-C), 122.0 (Ph-C), 121.8 (Ph-C), 116.2 (Ph-C), 116.1 (Ph-C). Anal. Calcd for C14H8ClFN2OS: C, 54.82%; H, 2.63%; N, 9.13%; Found: C, 54.79%; H, 2.62%; N, 9.14%.
6-chloro-N,N-bis(3,5-dimethoxybenzyl)benzo[d]thiazol-2-amine (D1) White solid; Yield/%: 20%; Mp/°C: 83.4∼85.3; ESI-MS [M + H]+: 485.1; 1H NMR (600 MHz, CDCl3) δ ppm: 7.533 (d, J = 2.04 Hz, 1H, Ph-H), 7.311 (d, J = 2.04 Hz, 1H, Ph-H), 6.331–6.549 (m, 7H, Ph-H), 4.663 (s, 4H, -CH2), 3.743 (s, 12H, -OCH3). 13C NMR (150 MHz, CDCl3) δ ppm: 169.2 (thiazole-C), 161.2 (Ph-C), 151.6 (benzothiazole-C), 138.6 (Ph-C), 132.3 (benzothiazole-C), 126.5 (Ph-C), 123.9 (Ph-C), 122.2 (Ph-C), 120.4 (Ph-C), 119.7 (Ph-C), 100.0 (Ph-C), 99.6 (Ph-C), 55.4 (-CH2-, -OCH3). Anal. Calcd for C25H25ClN2O4S: C, 61.91%; H, 5.20%; N, 5.78%; Found: C, 61.93%; H, 5.21%; N, 5.79%.
N,N-dibenzyl-6-chlorobenzo[d]thiazol-2-amine (D2) Gray solid; Yield/%: 21%; Mp/°C: 134.4∼136.4; ESI-MS [M + H]+: 365.1; 1H NMR (600 MHz, CDCl3) δ ppm: 7.321–7.360 (m, 10H, Ph-H), 7.282 (d, J = 2.34 Hz, 1H, Ph-H), 7.059 (dd, J = 8.7, 2.34 Hz, 1H, Ph-H), 6.962 (d, J = 8.52 Hz, 1H, Ph-H), 4.484 (s, 2H, -CH2), 4.178 (s, 2H, -CH2). 13C NMR (150 MHz, DMSO-d6) δ ppm: 136.1 (benzothiazole-C), 134.1 (Ph-C), 133.0 (Ph-C), 129.0 (Ph-C), 128.9 (Ph-C), 128.6 (Ph-C), 128.5 (Ph-C), 127.6 (Ph-C), 127.4 (Ph-C), 126.4 (Ph-C), 56.6 (-CH2-). Anal. Calcd for C21H17ClN2S: C, 69.13%; H, 4.70%; N, 7.68%; Found: C, 69.16%; H, 4.71%; N, 7.69%.
2.8 Oil-water partition coefficient (log P) measurement experiment
The partition coefficient between n-octanol and water (log Po/w) is the classical descriptor for Lipophilicity (Daina et al., 2017). The test methodology followed previously published protocols (Wang et al., 2021). At room temperature, two large Erlenmeyer flasks were taken and filled with n-octanol and water, respectively. The flasks were placed in a constant temperature shaker at 150 rpm for 24 h to saturate the solvents. The mixtures were then transferred to separating funnels under normal pressure to obtain water-saturated n-octanol and n-octanol-saturated water for subsequent use. The target compound (2 mg) was accurately weighed and placed in a 2 mL brown volumetric flask. Anhydrous methanol was added to ultrasonically dissolve the compound, and the mixture was diluted to volume and thoroughly shaken to prepare a 1 mg/mL stock solution. The stock solution was further diluted to obtain 1, 1.5, 2, 2.5, 5 and 10 μg/mL reference solutions for construction of a standard curve using a UV-visible spectrophotometer. An excess amount of the analyte was dissolved in water-saturated n-octanol, and the mixture was shaken at 150 rpm for 24 h at constant temperature to obtain a saturated solution. The saturated solution was allowed to stand, centrifuged, and 1 mL of the supernatant was transferred to a 4 mL centrifuge tube. N-octanol saturated water (1 mL) was added, and the mixture was shaken at 150 rpm for 24 h at constant temperature. After standing for 8 h, the mixture was centrifuged. Appropriate amounts of the n-octanol phase before and after equilibration were diluted with methanol, and concentrations were determined from the standard curve to obtain C0 and C1. The concentration in the n-octanol saturated aqueous phase, Cw, was calculated as Cw = C0 - C1. Thus, log Po/w = log10C0/Cw.
2.9 Cell lines and cell culture
Mouse monocyte macrophage leukemia cells RAW 264.7, human lung epithelial cells Beas-2b, human epidermoid carcinoma cells A431, non-small cell lung cancer cells A549 and H1299 were purchased from the Shanghai Cell Bank of the Chinese Academy of Sciences Committee. Beas-2b, A549 cells were cultured in DMEM/F12 (11330032, Gibco), and RAW264.7, A431, H1299 cells were cultured in high sugar DMEM (11965092, Gibco). Both media were supplemented with 10% FBS (12484028, Gibco) and 1% penicillin-streptomycin mixture (100×) (10378016, Gibco). Cells were grown in 37°C thermostat incubator (Thermo Fisher, United States) containing 5% CO2 and stored in −80°C for short-term storage, and in liquid nitrogen for long-term storage. None of the cell resuscitation passages used in the experiments herein exceeded 20 generations.
2.10 Cell proliferation and toxicity assay
Beas-2b, A431, A549 and H1299 cells were cultured in 96-well plates (5 × 103 cells/well) for 16 h, respectively. Subsequently, positive compound 4i and 25 newly synthesized compounds were added at a final concentration of 10 μM or gradient concentrations (0.01, 0.1, 0.5, 1, 5 and 10 μM), co-incubated with the cells for 48 h. MTT (M8180, Solarbio) solutions (5 mg/mL) were then added and incubated for 4 h in the dark. Then, formazan crystals were dissolved with DMSO (D8371, Solarbio), followed by shaking on a shaker (DRAGONLAB, China) for 10 min. Finally, absorbance at 490 nm wavelength for each well was measured with Microplate reader (Molecular Devices, United States) (Tang et al., 2020; Lee et al., 2021). The results are expressed as mean ± SD from three independent experiments. Cells inhibition rates or IC50 values were calculated using GraphPad Prism 9.5.0.
2.11 Anti-inflammation activity assay
RAW264.7 cells were cultured in 6-well plates (2×105 cells/well) for 24 h and treated with 25 newly synthesized compounds (final concentration at 10 μM) for 30 min, respectively. Subsequently, the cells were stimulated with 1 μL of LPS (500 ng/mL) (L8880, Solarbio). After 24 h, the supernatant was collected and analyzed using an ELISA kit (EK206 and EK182, MULTISCIENCES) to quantify the levels of inflammatory cytokines IL-6 and TNF-α (Li et al., 2021).
2.12 Flow cytometry analysis of cell apoptosis
The A431 and A549 cells were cultured in 6-well plates (1×105 cells/mL) for 16 h. Subsequently, cells were treated with different concentrations (1, 2 and 4 μM) of either B7 or 4i for 24 h. Then, the cells were collected, washed, and stained with the FITC Annexin V Apoptosis Detection Kit I (556547, BD) (Jiang et al., 2017). Sample testing was performed using a FACS Calibur Flow Cytometer (BD, United States), and subsequent data analysis was performed using FlowJo 10.6.2.
2.13 Flow cytometry analysis of cell cycle
The A431 and A549 cells were cultured in 6-well plates (1×105 cells/mL) for 16 h. Subsequently, they were treated with different concentrations (1, 2 and 4 μM) of either B7 or 4i (4 μM) for 24 h. Cells were collected, mixed by adding 75% ethanol with shaking on a vortex shaker, and placed in the refrigerator at 4°C for overnight fixation. The cells were incubated with PI-Raze solution for 15 min at room temperature, protected from light, according to the instructions of BD Cycletest Plus DNA Reagent Kit (340242, BD), and detected by FACS Calibur flow cytometry (Oh et al., 2023), and subsequent data analysis was performed using FlowJo 10.6.2.
2.14 Wound healing analysis of cell migration
The A431 and A549 cells were cultured in 6-well plates (5×105 cells/well). When the cell confluency reached approximately 90%, used a sterile 10 μL pipette tip to create three parallel scratches evenly. Subsequently, rinsed with PBS to remove floating cells, and then treated with B7 and 4i at final concentrations of 4 μM. Captured images using a microscope camera system (Nikon, JPN) at 0 and 48 h post-treatment (Xu et al., 2023).
2.15 Western blot analysis
The A431 and A549 cells were cultured in 6-well plates (2×105 cells/well) for 2 h and treated with different concentrations of B7(1, 5, and 10 μM) or 4i (10 μM). The corresponding cells were collected, washed with PBS (P1020, Solarbio), and lysed with RIPA buffer (R0010, Solarbio) to extract the total proteins. The extracted protein was loaded, subjected to SDS-PAGE electrophoresis (Bio-Rad) (Xu et al., 2023), and then the protein was transferred to a PDVF membrane (IPVH00010, Millipore) and incubated in the corresponding Primary Antibody AKT (9272, Cell Signaling Technology), phospho-AKT (4058, Cell Signaling Technology), ERK (A4782, ABclonal Technology), phospho-ERK (AP0974, ABclonal Technology) and GAPDH (AB0037, Abways Technology) overnight. Then, the Primary Antibody was recovered and enzyme-labeled secondary antibodies Goat Anti-Rabbit IgG HRP (H + L) (A0208, Beyotime Technology) were used. Finally, Imaging was performed on a gel Imaging System (Bio-Rad, United States) using the Ultra-sensitive ECL Chemiluminescence Assay Kit (P0018AS, Beyotime Technology).
2.16 ADMET analysis
The physicochemical parameters, pharmacokinetic properties and drug similarity of the active compound B7 were predicted using Swiss ADME web server (http://www.swissadme.ch/) (Daina et al., 2017). The toxicity associated with compound B7 was predicted by admetSAR web server (http://lmmd.ecust.edu.cn/admetsar2) (Cheng et al., 2012).
2.17 Statistical analysis
For the statistical analysis, Microsoft Excel 2016 and GraphPad Prism 9.5.0 software were used. The results were presented as the mean ± standard error of the mean. Statistical analyses were conducted via Student’s t-test. A value of p < 0.05 is defined as statistically significant. Statistical significance differences (compared to control group) are defined as follows: p > 0.05 (not significant, ns), p ≤ 0.05 (*), p ≤ 0.01 (**) and p ≤ 0.001 (***).
3 Results
3.1 Chemistry
The synthetic pathways for the compounds in series A, B, C, and D were elucidated in Scheme 2. Commencing with benzothiazole and 2-hydroxyiodobenzene, and utilizing DMSO as the solvent, 2-phenol-benzothiazole was synthesized through nucleophilic substitution reactions. Subsequent steps involved the use of 2-phenol-benzothiazole and various substituted benzyl bromides as initial reactants, leading to the formation of eight compounds in the A series through the Williamson synthesis method. The B series is created by employing 2-amino-halogenated benzothiazole and diverse substituted benzyl bromides, resulting in the synthesis of eight compounds via amine halogenation reactions. The synthesis of the C series involved the use of 2-amino-halogenated benzothiazole and various substituted acyl chlorides, resulting in the production of seven compounds via amide formation reactions. Similarly, the synthetic procedure for the D series compounds closely resembled that of the B series. To validate the synthesized compounds in this study, thorough analyses utilizing 1H NMR, 13C NMR, ESI-MS, HPLC and elemental analysis were conducted, confirming the accuracy of their structures (Supplementary Material).
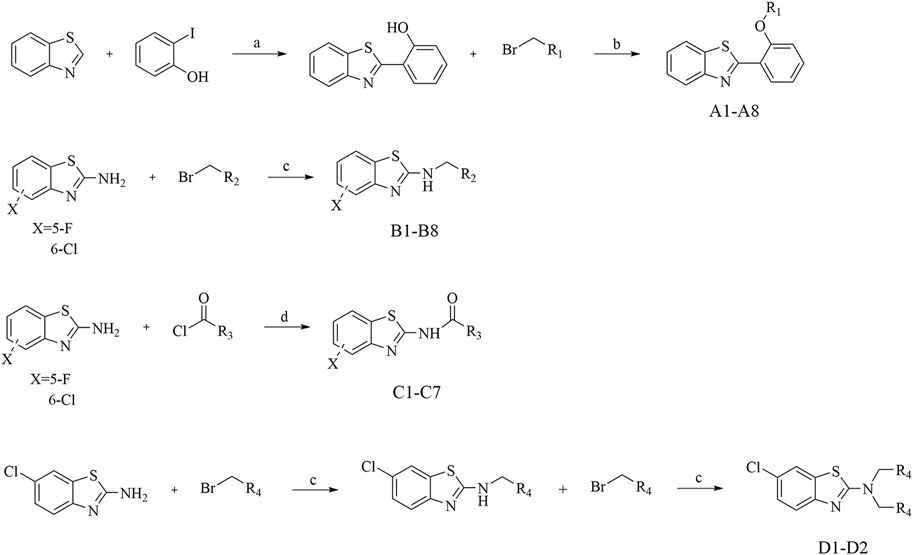
Scheme 2. Synthesis of A Series, B Series, C Series, and D Series. Reagents and conditions: (A) K2CO3, DMSO, 120°C, 6 h; (B) K2CO3, acetonitrile, rt, 3 h; (C) K2CO3, acetonitrile, 65°C, 6 h; (D) Et3N, dioxane, 100°C, 3 h.
3.2 Biological evaluation
3.2.1 Anti-proliferation assay in vitro
To assess the anti-proliferative effects of the compounds on cells, we employed the MTT method to analyze the impact of all 2-amino-benzo[d] thiazole derivatives, along with the lead compound 4i, at a concentration of 10 μM across Beas-2b, A431, A549, and H1299 cells (Tables 1–3). At the same time, we tested the log p values of 25 new compounds. According to Lipinski’s rule of five (Ro5), the calculated log p-value should be < 5 for compounds intended for oral administration (Lipinski et al., 2001; Danalev et al., 2023). As shown in Tables 1–3, almost all of the compounds had log p < 5, which indicates that our newly synthesized compounds have good lipid solubility and have the conditions for drug formation.
The results indicated that following treatment with various compounds, the cell viability of Beas-2b cells remained consistently above 70%, underscoring the safety and reliability of the synthesized compounds at this concentration. The inhibitory rates of A series compounds on A431, A549, and H1299 cancer cells were consistently below 50%. Conversely, compounds from the B series (B5, B7, and B8) as well as those from the C and D series (C5 and C6) demonstrated notable inhibitory effects on these cancer cells, surpassing 50% inhibition rates. Notably, compound B7 exhibited a potent anti-proliferative effect, with inhibition rates exceeding 75% for all three cancer cell lines.
In summary, the data suggested that the recently synthesized compounds (B5, B7, B8, C5, and C6) exhibit promising anti-cancer properties without causing notable toxicity. Subsequent research and exploration are deemed necessary.
Then compounds B5, B7, B8, C5, and C6 were assessed at gradient concentrations (0.01, 0.1, 0.5, 1, 5 and 10 μM), revealing significant inhibitory effects on A431, A549, and H1299 cell lines. Subsequently, the IC50 tests for A431, A549, and H1299 cells were conducted on the six selected compounds (Table 4). Notably, among these compounds, B7 exhibited superior inhibitory effects across all three cancer cell lines, with IC50 values of 1.51 ± 0.20 μM for A431, 0.96 ± 0.24 μM for A549, and 1.68 ± 1.32 μM for H1299 cells. Remarkably, B7 surpassed the efficacy of the other five compounds and demonstrated marginally superior inhibitory activity compared to the reference compound 4i. Determining the IC50 values for B7 lays the groundwork for further biological experiments, offering crucial insights for conducting experiments at specific dosage concentrations.
3.2.2 Anti-inflammatory activity assay in vitro
In consideration of the interconnected development of inflammation and tumors, we undertook an initial screening of recently synthesized compounds to assess their anti-inflammatory activity. The parameters under scrutiny encompassed IL-6 and TNF-α, both serving as representative cytokines expressed significantly post-stimulation (Lee et al., 2011; Kumar and Singh, 2021). The down-regulatory effects of each compound on IL-6 and TNF-α were evaluated using ELISA Kit. Specifically, the ELISA method was employed to examine the impact of all recently synthesized compounds on the expression of IL-6 and TNF-α inflammatory factors at a concentration of 10 µM (Figure 2). Combining the expression levels of both inflammatory factors, in comparison to the blank control, compounds A1, B7, and D2 demonstrated significant inhibitory effects on IL-6 and TNF-α expression at 10 μM, suggesting potent anti-inflammatory activity. Particularly, D2 exhibited the most favorable effects, followed by B7, which showed efficacy comparable to that of D2.
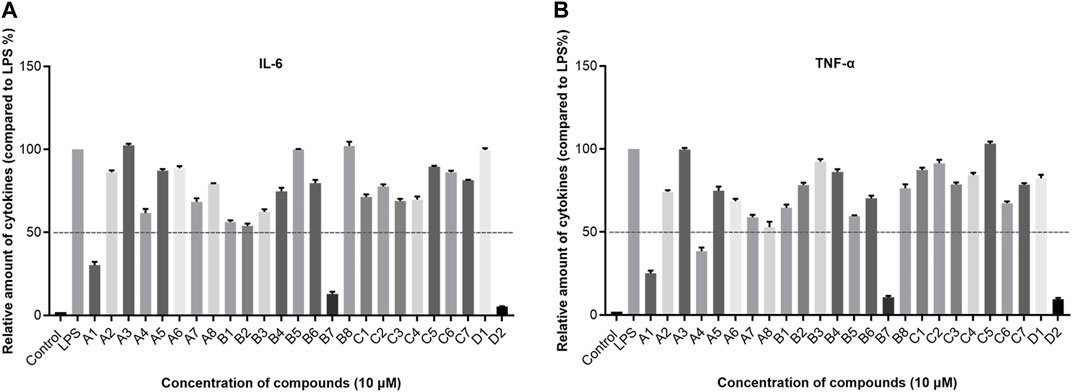
Figure 2. The expression levels of IL-6 and TNF-α in vitro. (A) The expression of IL-6 after compounds treatment; (B) The expression of TNF-α after compounds treatment.
3.2.3 B7 promoted apoptosis of A431 and A549 cells
In subsequent experiments, a concise mechanistic exploration was undertaken on the optimized compound B7. The objective was to examine whether B7 induces apoptosis in A431 and A549 cells. Flow cytometry was employed to assess the impact of B7 on cancer cell apoptosis 24 h post-treatment (Figure 3). The findings unveiled a gradual increase in the apoptosis rate of both A431 and A549 cells with the escalating concentration of B7. Notably, at a concentration of 4 μM, the pro-apoptotic effect of B7 equaled that of the lead compound 4i and, indeed, surpassed the efficacy of 4i.
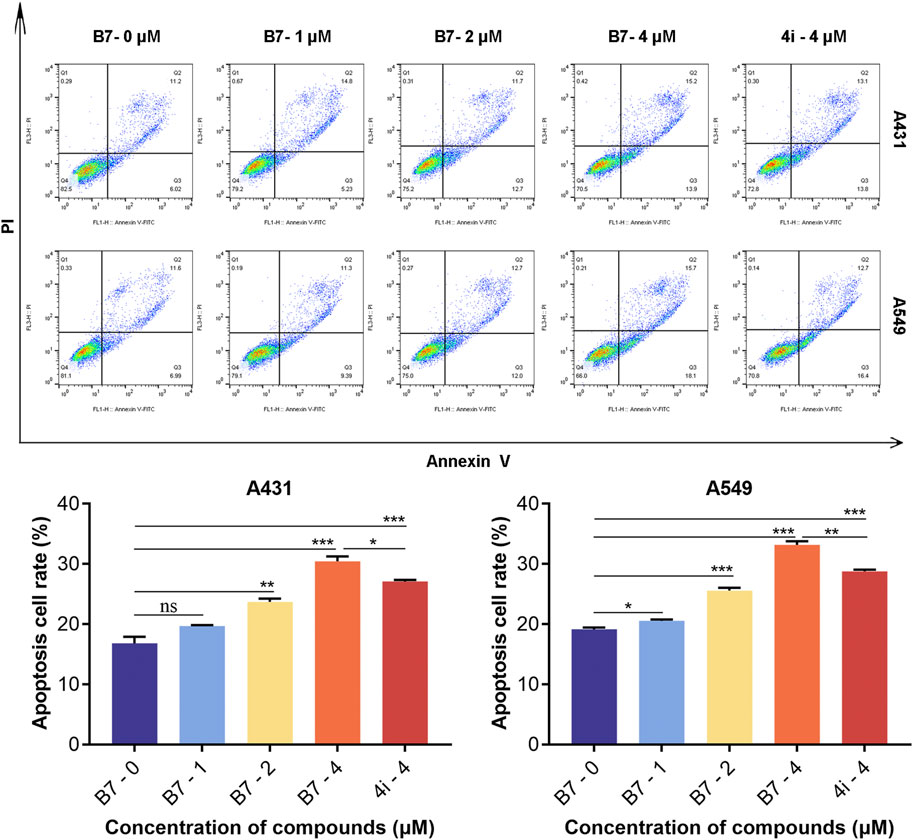
Figure 3. Apoptosis of A431 and A549 cells treated with B7 or 4i. Statistical significance differences were set to p > 0.05 (ns), p ≤ 0.05 (*), p ≤ 0.01 (**) and p ≤ 0.001 (***).
3.2.4 B7 blocked the cycle of A431 and A549 cells
In a parallel fashion, concentrations of 1, 2, and 4 μM were utilized for the application of flow cytometry to evaluate the cell cycle arrest induced by compound B7 in A431 and A549 cells. As shown in Figure 4, compound B7 and 4i manifested a G2 phase cell cycle arrest effect on cancer cells, with B7 exhibiting a noteworthy dose-dependent response. Notably, the cell cycle arrest induced by B7 in both A431 and A549 cells surpassed that of 4i at 4 μM.
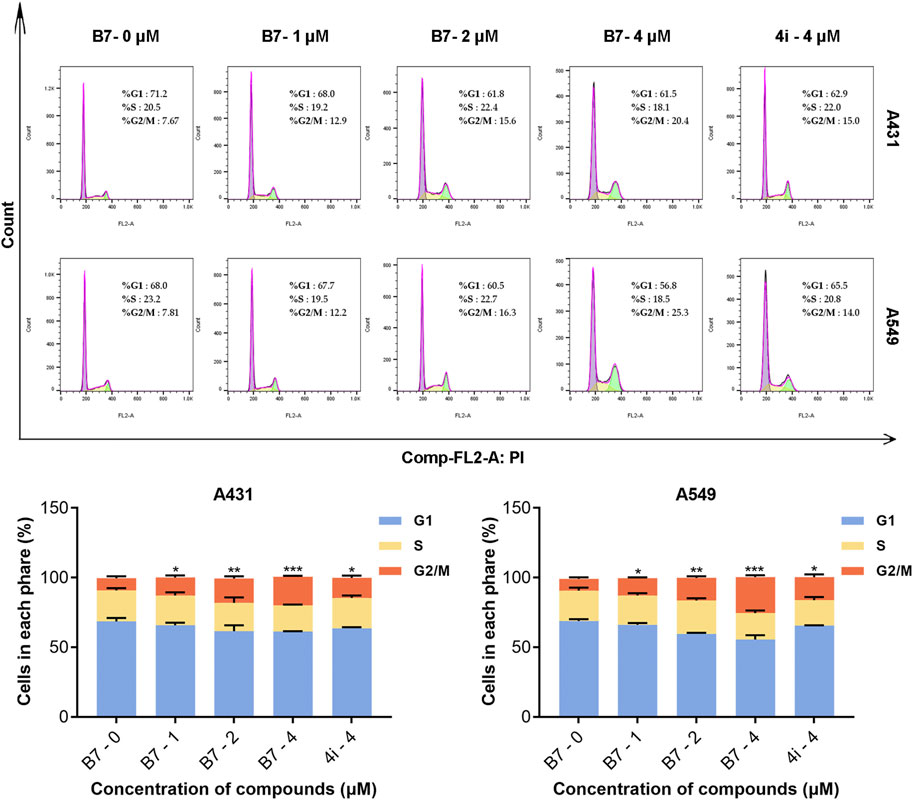
Figure 4. Cycle block of A431 and A549 cells treated with B7 or 4i. Statistical significance differences were set to p > 0.05 (ns), p ≤ 0.05 (*), p ≤ 0.01 (**) and p ≤ 0.001 (***).
3.2.5 B7 inhibited the migration of A431 and A549 cells
Tumor cells are recognized for their capacity for unrestricted proliferation and resistance to apoptosis, accompanied by a proclivity for facile metastasis (Khan et al., 2023). Consequently, we employed the wound healing assay to assess the migratory inhibitory effects of B7 and 4i on A431 and A549 cells across 4 μM for 48 h (Figure 5). The findings indicated that in A431 cells, the migration inhibitory effect of B7 slightly surpassed that of 4i. However, as none of the groups exhibited complete healing, extending the experiment duration until full healing is observed could yield more precise conclusions. It is noteworthy that in A549 cells, scratches in the DMSO blank control group had fully healed, highlighting the stronger inhibitory effect of B7 on cell migration compared to 4i. In conclusion, B7 demonstrated superior efficacy in inhibiting cancer cell migration.
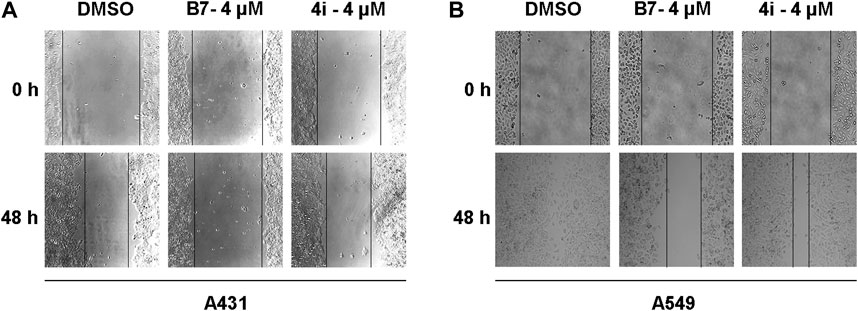
Figure 5. Migration of A431 and A549 cells treated with B7 or 4i. (A) The migration of A431 cells treated with B7 or 4i for 48 h; (B) The migration of A549 cells treated with B7 or 4i for 48 h.
3.2.6 B7 inhibited the phosphorylation of AKT and ERK
The anticipated experimental findings underscored the significant anti-promotion, pro-apoptotic, cell cycle arrest effects, and anti-inflammatory properties of B7 on A431 and A549 cells. A psychological inquiry into the pathways or key mechanisms through which B7 manifests its remarkable anti-cancer and anti-inflammatory activity prompted investigation. To this end, we examined the activity of common anti-tumor receptor kinases, revealing that B7 had minimal impact on them. Furthermore, employing Western blot analysis to scrutinize prevalent anti-tumor and anti-inflammatory signaling pathways, we observed that B7 can markedly inhibit both AKT and ERK protein phosphorylation simultaneously, surpassing the inhibitory effect observed with 4i (Figure 6). The synergistic effects of the AKT and ERK pathways in cancer therapy have garnered considerable attention. Some studies propose that co-inhibiting these two pathways may be more effective than inhibiting either one alone, as it can concurrently disrupt multiple biological processes in cells, thereby enhancing the overall effectiveness of treatment (Dimri et al., 2020; Wu et al., 2023). The concurrent inhibition of the AKT and ERK pathways by compound B7 may elucidate its efficacy in both anti-inflammatory and anticancer capacities.
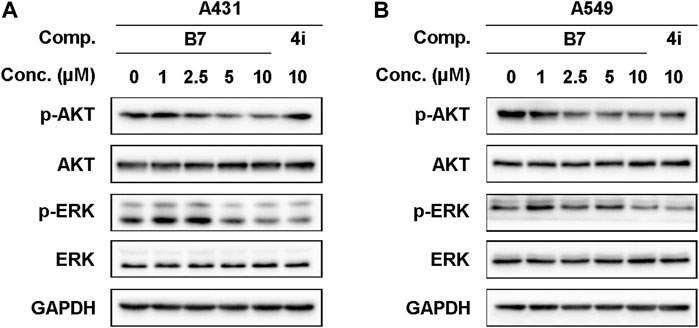
Figure 6. The protein expression levels of A431 and A549 cells treated with B7 or 4i. (A) The protein expression levels of A431 cells treated with B7 or 4i for 2 h; (B) The protein expression levels of A549 cells treated with B7 or 4i for 2 h.
3.2.7 In silico ADMET assessment of compound B7
The results of ADME and toxicity prediction were shown in Table 5. Based on the ADME prediction results, it could be seen that the physicochemical properties, ADME indexes and pharmacokinetic properties of B7 were within the permissible parameters (Ogbodo et al., 2023). From the toxicity prediction results, B7 had certain hepatotoxicity, but it was not irritating and corrosive to eyes and skin, and was not carcinogenic (Melo et al., 2022). Based on the above results, it was clear that B7 was a compound with better drug-like properties and deserved further studies.
4 Discussion
In this study, a series of novel benzothiazole derivatives was synthesized, and their anti-proliferative and anti-inflammatory activities were systematically assessed in cellular contexts. The results delineate variable inhibitory effects of these compounds on the A431, A549, and H1299 cell lines. Notably, compound B7 exhibits superior inhibitory effects across all three cancer cell lines. Furthermore, these compounds demonstrate a capacity to downregulate the expression of inflammatory factors IL-6 and TNF-α to varying extents. Specifically, compounds B7 and D2 significantly suppress the expression of IL-6 and TNF-α.
In light of the anti-tumor and anti-inflammatory activities of the target compounds, we conducted a comprehensive Structure-Activity Relationship (SAR) analysis on a novel series of 2-aminobenzothiazole compounds. The A-series compounds demonstrated inhibition rates below 50% for A549, A431, and H1299 cells, whereas the B, C, and D-series exhibited pronounced inhibitory effects on these cancer cells. It can be deduced that the benzylamine moiety, tethered to the benzothiazole ring in this compound class, serves as an indispensable pharmacophore and a pivotal pharmacophoric group. This necessity arises from the requirement for a larger substituent at this position to occupy, thereby promoting a more stable conformation. Upon comparison of the bioactivity between B-series and C-series compounds, it was observed that the overall bioactivity of B-series compounds surpasses that of the C-series. This observation implies that the introduction of a carbonyl group to form an amide bond did not yield an enhancement in anti-tumor and anti-inflammatory activities. Further scrutiny of various substituents on the benzothiazole ring unveiled a notable increase in bioactivity when chlorine is situated at the 6th position on the benzothiazole ring, in contrast to fluorine substitution at the 5th position. Exploring additional substituents on benzothiazole may hold promise for optimizing the activity of these compounds. The compound D2 exhibits significant advantages in anti-inflammatory aspects, which may be related to its unique bisubstituted structure, worthy of further study.
Additionally, the presence of a nitro group on compound B7 has captured our attention. The nitro group is a common and distinctive functional group in medicinal chemistry, prevalent in various classes of drugs including anticancer agents, antibiotics, antituberculosis medications, antiparasitic agents, sedatives, insecticides, and herbicides. This moiety exhibits a potent electron-withdrawing capacity, leading to the formation of localized electron-deficient sites within the molecule. It engages with nucleophilic reagents present in biological systems such as proteins, amino acids, nucleic acids, and enzymes through processes like nucleophilic addition, electron transfer, or complexation. Consequently, compounds containing nitro groups have been extensively studied in medicinal chemistry research. For instance, Nifurtimox (Rolon et al., 2022; Xin et al., 2022; Eslin et al., 2023), utilized in the treatment of Chagas disease and recurrent neuroblastoma, and Venetoclax (Li et al., 2024a), a Bcl-2 inhibitor used for managing chronic lymphocytic leukemia, both feature nitro structures. However, it is imperative to acknowledge that drugs incorporating nitro groups often elicit severe adverse reactions and toxicity, including carcinogenicity, hepatotoxicity, mutagenicity, and bone marrow suppression. Consequently, the nitro group is frequently considered a red flag structural motif, which somewhat impedes the exploration of its therapeutic potential. Nevertheless, in our study, the investigated compounds demonstrated negligible inhibitory effects on Beas-2b cells, indicating a lack of cytotoxicity in vitro. However, further investigation is warranted to evaluate their toxicity in vivo.
A key mechanism underlying B7’s anticancer efficacy appears to be its dual inhibition of the AKT and ERK signaling pathways. The AKT and ERK cascades play pivotal roles in tumor cell proliferation, survival, and metastasis (Chen et al., 2023; Cui et al., 2023). While previous benzothiazole-based inhibitors have targeted single pathways, B7 is unique in concurrently suppressing both AKT and ERK phosphorylation. This dual targeting likely contributes to B7’s potent induction of apoptosis, cell cycle arrest, and inhibition of cell migration in our experiments.
Additionally, B7 significantly reduces the production of the inflammatory cytokines IL-6 and TNF-α. As highlighted in the Introduction, chronic inflammation driven by these factors can create microenvironments conducive to tumor growth and progression. Therefore, B7’s anti-inflammatory properties may further augment its anticancer effects.
Future studies should focus on elucidating the precise molecular interactions enabling B7’s dual pathway inhibition. Testing B7’s efficacy in animal models will also be crucial to assess its potential for clinical translation. Limitations of this initial study include the narrow range of cancer cell lines examined. Expanding the panel of cell lines could provide further insights into B7’s spectrum of anticancer activity.
5 Conclusion
In summary, this study has innovatively developed a series of benzothiazole derivatives, with a focus on compound B7 due to its notable dual anticancer and anti-inflammatory activities. B7 stands out for its ability to significantly reduce cancer cell proliferation in A431, A549, and H1299 cell lines and lower the levels of inflammatory cytokines IL-6 and TNF-α. These results position B7 as a promising candidate for dual-action cancer therapy. The study’s mechanistic exploration, highlighting B7’s simultaneous inhibition of the AKT and ERK pathways, offers a novel strategy for addressing both the survival mechanisms of tumor cells and the inflammatory milieu facilitating cancer progression.
Data availability statement
The original contributions presented in the study are included in the article/Supplementary Material, further inquiries can be directed to the corresponding authors.
Author contributions
XX: Writing–original draft, Conceptualization, Data curation, Funding acquisition. ZZ: Conceptualization, Investigation, Writing–original draft, Software. SC: Conceptualization, Investigation, Writing–original draft. YF: Methodology, Writing–original draft. JZ: Formal Analysis, Writing–original draft. YG: Resources, Writing–original draft. ZX: Data curation, Writing–original draft. YX: Supervision, Writing–original draft. XW: Funding acquisition, Supervision, Writing–original draft. FY: Writing–review and editing, Conceptualization. HC: Funding acquisition, Writing–review and editing. XX: Funding acquisition, Writing–review and editing. XY: Conceptualization, Formal Analysis, Investigation, Software, Writing–review and editing.
Funding
The author(s) declare that financial support was received for the research, authorship, and/or publication of this article. This research was funded by the Basic social development science and technology projects of Wenzhou (grant no. Y20210214, Y20220206); The Basic social development science and technology projects of Taizhou (grant no. 20ywb67) and the scientific research projects of Zhejiang Provincial Education Department (grant no. Y202249707).
Acknowledgments
The authors extend their appreciation to the Analysis and Testing Center of Wenzhou Medical University for generously providing the essential equipment utilized in this study.
Conflict of interest
The authors declare that the research was conducted in the absence of any commercial or financial relationships that could be construed as a potential conflict of interest.
Publisher’s note
All claims expressed in this article are solely those of the authors and do not necessarily represent those of their affiliated organizations, or those of the publisher, the editors and the reviewers. Any product that may be evaluated in this article, or claim that may be made by its manufacturer, is not guaranteed or endorsed by the publisher.
Supplementary material
The Supplementary Material for this article can be found online at: https://www.frontiersin.org/articles/10.3389/fchem.2024.1384301/full#supplementary-material
References
Al-Tel, T. H., Al-Qawasmeh, R. A., and Zaarour, R. (2011). Design, synthesis and in vitro antimicrobial evaluation of novel Imidazo[1,2-a]pyridine and imidazo[2,1-b] [1,3]benzothiazole motifs. Eur. J. Med. Chem. 46, 1874–1881. doi:10.1016/j.ejmech.2011.02.051
Awadh, A. A. A. (2023). Biomedical applications of selective metal complexes of indole, benzimidazole, benzothiazole and benzoxazole: A review (from 2015 to 2022). Saudi Pharm J. 31, 101698. doi:10.1016/j.jsps.2023.101698
Bray, F., Laversanne, M., Weiderpass, E., and Soerjomataram, I. (2021). The ever-increasing importance of cancer as a leading cause of premature death worldwide. Cancer 127, 3029–3030. doi:10.1002/cncr.33587
Chang, L., Ruiz, P., Ito, T., and Sellers, W. R. (2021). Targeting pan-essential genes in cancer: challenges and opportunities. Cancer Cell 39, 466–479. doi:10.1016/j.ccell.2020.12.008
Cheng, F., Li, W., Zhou, Y., Shen, J., Wu, Z., Liu, G., et al. (2012). admetSAR: a comprehensive source and free tool for assessment of chemical ADMET properties. J. Chem. Inf. Model 52, 3099–3105. doi:10.1021/ci300367a
Chen, L., Zhu, S., Liu, T., Zhao, X., Xiang, T., Hu, X., et al. (2023). Aberrant epithelial cell interaction promotes esophageal squamous-cell carcinoma development and progression. Signal Transduct. Target Ther. 8, 453. doi:10.1038/s41392-023-01710-2
Choi, M. M., Kim, E. A., Hahn, H. G., Nam, K. D., Yang, S. J., Choi, S. Y., et al. (2007). Protective effect of benzothiazole derivative KHG21834 on amyloid β-induced neurotoxicity in PC12 cells and cortical and mesencephalic neurons. Toxicology 239, 156–166. doi:10.1016/j.tox.2007.07.010
Cowan, A. J., Green, D. J., Kwok, M., Lee, S., Coffey, D. G., Holmberg, L. A., et al. (2022). Diagnosis and management of multiple myeloma: a review. Jama 327, 464–477. doi:10.1001/jama.2022.0003
Craig, M., Jenner, A. L., Namgung, B., Lee, L. P., and Goldman, A. (2021). Engineering in medicine to address the challenge of cancer drug resistance: from micro- and nanotechnologies to computational and mathematical modeling. Chem. Rev. 121, 3352–3389. doi:10.1021/acs.chemrev.0c00356
Cui, Y., Wu, X., Jin, J., Man, W., Li, J., Li, X., et al. (2023). CircHERC1 promotes non-small cell lung cancer cell progression by sequestering FOXO1 in the cytoplasm and regulating the miR-142-3p-HMGB1 axis. Mol. Cancer 22, 179. doi:10.1186/s12943-023-01888-7
Daina, A., Michielin, O., and Zoete, V. (2017). SwissADME: a free web tool to evaluate pharmacokinetics, drug-likeness and medicinal chemistry friendliness of small molecules. Sci. Rep. 7, 42717. doi:10.1038/srep42717
Danalev, D., Iliev, I., Dobrev, S., Angelova, S., Petrin, S., Dzimbova, T., et al. (2023). Synthesis, antiproliferative effect and in silico LogP prediction of BIM-23052 analogs containing tyr instead of phe. Pharmaceutics 15, 1123. doi:10.3390/pharmaceutics15041123
D'Angelo, N. D., Kim, T. S., Andrews, K., Booker, S. K., Caenepeel, S., Chen, K., et al. (2011). Discovery and optimization of a series of benzothiazole phosphoinositide 3-kinase (PI3K)/mammalian target of rapamycin (mTOR) dual inhibitors. J. Med. Chem. 54, 1789–1811. doi:10.1021/jm1014605
Dimri, M., Humphries, A., Laknaur, A., Elattar, S., Lee, T. J., Sharma, A., et al. (2020). NAD(P)H quinone dehydrogenase 1 ablation inhibits activation of the phosphoinositide 3-kinase/akt serine/threonine kinase and mitogen-activated protein kinase/extracellular signal-regulated kinase pathways and blocks metabolic adaptation in hepatocellular carcinoma. Hepatology 71, 549–568. doi:10.1002/hep.30818
Dinić, J., Efferth, T., GarcíA-Sosa, A. T., Grahovac, J., PadróN, J. M., Pajeva, I., et al. (2020). Repurposing old drugs to fight multidrug resistant cancers. Drug Resist Updat 52, 100713. doi:10.1016/j.drup.2020.100713
El-Helby, A. A., Sakr, H., Eissa, I. H., Al-Karmalawy, A. A., and El-Adl, K. (2019). Benzoxazole/benzothiazole-derived VEGFR-2 inhibitors: design, synthesis, molecular docking, and anticancer evaluations. Arch. Pharm. Weinh. 352, e1900178. doi:10.1002/ardp.201900178
Eslin, D., Zage, P. E., Bergendahl, G., Lewis, E., Roberts, W., Kraveka, J., et al. (2023). A phase II trial of nifurtimox combined with topotecan and cyclophosphamide for refractory or relapsed neuroblastoma and medulloblastoma. Int. J. Cancer 153, 1026–1034. doi:10.1002/ijc.34569
Ferlay, J., Soerjomataram, I., Dikshit, R., Eser, S., Mathers, C., Rebelo, M., et al. (2015). Cancer incidence and mortality worldwide: sources, methods and major patterns in GLOBOCAN 2012. Int. J. Cancer 136, E359–E386. doi:10.1002/ijc.29210
Fitzmaurice, C., Dicker, D., Pain, A., Hamavid, H., Moradi-Lakeh, M., Macintyre, M. F., et al. (2015). The global burden of cancer 2013. JAMA Oncol. 1, 505–527. doi:10.1001/jamaoncol.2015.0735
Ghanbar, M. I., and Suresh, K. (2024). Pulmonary toxicity of immune checkpoint immunotherapy. J. Clin. Invest. 134, e170503. doi:10.1172/jci170503
Hastir, J. F., Delbauve, S., Larbanoix, L., Germanova, D., Goyvaerts, C., Allard, J., et al. (2020). Hepatocarcinoma induces a tumor necrosis factor-dependent kupffer cell death pathway that favors its proliferation upon partial hepatectomy. Front. Oncol. 10, 547013. doi:10.3389/fonc.2020.547013
Jiang, S. X., Qi, B., Yao, W. J., Gu, C. W., Wei, X. F., Zhao, Y., et al. (2017). Berberine displays antitumor activity in esophageal cancer cells in vitro. World J. Gastroenterol. 23, 2511–2518. doi:10.3748/wjg.v23.i14.2511
Kamal, A., Reddy, K. S., Khan, M. N., Shetti, R. V., Ramaiah, M. J., Pushpavalli, S. N., et al. (2010). Synthesis, DNA-binding ability and anticancer activity of benzothiazole/benzoxazole-pyrrolo[2,1-c] [1,4]benzodiazepine conjugates. Bioorg Med. Chem. 18, 4747–4761. doi:10.1016/j.bmc.2010.05.007
Kamal, A., Srikanth, Y. V., Naseer Ahmed Khan, M., Ashraf, M., Kashi Reddy, M., Sultana, F., et al. (2011). 2-Anilinonicotinyl linked 2-aminobenzothiazoles and [1,2,4]triazolo[1,5-b] [1,2,4]benzothiadiazine conjugates as potential mitochondrial apoptotic inducers. Bioorg Med. Chem. 19, 7136–7150. doi:10.1016/j.bmc.2011.09.060
Khan, S. U., Fatima, K., Malik, F., Kalkavan, H., and Wani, A. (2023). Cancer metastasis: molecular mechanisms and clinical perspectives. Pharmacol. Ther. 250, 108522. doi:10.1016/j.pharmthera.2023.108522
Kumar, G., and Singh, N. P. (2021). Synthesis, anti-inflammatory and analgesic evaluation of thiazole/oxazole substituted benzothiazole derivatives. Bioorg Chem. 107, 104608. doi:10.1016/j.bioorg.2020.104608
Kumbhare, R. M., Kosurkar, U. B., Janaki Ramaiah, M., Dadmal, T. L., Pushpavalli, S. N., and Pal-Bhadra, M. (2012). Synthesis and biological evaluation of novel triazoles and isoxazoles linked 2-phenyl benzothiazole as potential anticancer agents. Bioorg Med. Chem. Lett. 22, 5424–5427. doi:10.1016/j.bmcl.2012.07.041
Lee, H. J., Pham, P. C., Pei, H., Lim, B., Hyun, S. Y., Baek, B., et al. (2021). Development of the phenylpyrazolo[3,4-d]pyrimidine-based, insulin-like growth factor receptor/Src/AXL-targeting small molecule kinase inhibitor. Theranostics 11, 1918–1936. doi:10.7150/thno.48865
Lee, Y. R., Jin, G. H., Lee, S. M., Park, J. W., Ryu, J. H., Jeon, R., et al. (2011). Inhibition of TNF-α-mediated inflammatory responses by a benzodioxolylacetylamino-linked benzothiazole analog in human fibroblast-like synoviocytes. Biochem. Biophys. Res. Commun. 408, 625–629. doi:10.1016/j.bbrc.2011.04.073
Li, F., Liu, J., Liu, C., Liu, Z., Peng, X., Huang, Y., et al. (2024a). Cyclic peptides discriminate BCL-2 and its clinical mutants from BCL-XL by engaging a single-residue discrepancy. Nat. Commun. 15, 1476. doi:10.1038/s41467-024-45848-1
Li, L., Yu, M., Li, Y., Li, Q., Yang, H., Zheng, M., et al. (2021). Synergistic anti-inflammatory and osteogenic n-HA/resveratrol/chitosan composite microspheres for osteoporotic bone regeneration. Bioact. Mater 6, 1255–1266. doi:10.1016/j.bioactmat.2020.10.018
Lipinski, C. A., Lombardo, F., Dominy, B. W., and Feeney, P. J. (2001). Experimental and computational approaches to estimate solubility and permeability in drug discovery and development settings. Adv. Drug Deliv. Rev. 46, 3–25. doi:10.1016/s0169-409x(96)00423-1
Li, X., Shen, M., Yang, J., Liu, L., and Yang, Y. W. (2024b). Pillararene-based stimuli-responsive supramolecular delivery systems for cancer therapy. Adv. Mater, e2313317. doi:10.1002/adma.202313317
Makowska, A., Wolff, L., Sączewski, F., Bednarski, P. J., and Kornicka, A. (2019). Synthesis and cytotoxic evaluation of benzoxazole/benzothiazole-2-imino-coumarin hybrids and their coumarin analogues as potential anticancer agents. Pharmazie 74, 648–657. doi:10.1691/ph.2019.9664
Melo, D. S., Nery Neto, J. A. O., Santos, M. S. D., Pimentel, V. D., Carvalho, R. C. V., Sousa, V. C., et al. (2022). Isopropyl gallate, a gallic acid derivative: in silico and in vitro investigation of its effects on leishmania major. Pharmaceutics 14, 2701. doi:10.3390/pharmaceutics14122701
Meza, K., Biswas, S., Zhu, Y. S., Gajjar, A., Perelstein, E., Kumar, J., et al. (2021). Tumor necrosis factor-alpha is associated with mineral bone disorder and growth impairment in children with chronic kidney disease. Pediatr. Nephrol. 36, 1579–1587. doi:10.1007/s00467-020-04846-3
Mokesch, S., Cseh, K., Geisler, H., Hejl, M., Klose, M. H. M., Roller, A., et al. (2020). Investigations on the anticancer potential of benzothiazole-based metallacycles. Front. Chem. 8, 209. doi:10.3389/fchem.2020.00209
Mortimer, C. G., Wells, G., Crochard, J. P., Stone, E. L., Bradshaw, T. D., Stevens, M. F., et al. (2006). Antitumor benzothiazoles. 26. 2-(3,4-Dimethoxyphenyl)-5-fluorobenzothiazole (GW 610, NSC 721648), a simple fluorinated 2-arylbenzothiazole, shows potent and selective inhibitory activity against lung, colon, and breast cancer cell lines. J. Med. Chem. 49, 179–185. doi:10.1021/jm050942k
Noolvi, M. N., Patel, H. M., and Kaur, M. (2012). Benzothiazoles: search for anticancer agents. Eur. J. Med. Chem. 54, 447–462. doi:10.1016/j.ejmech.2012.05.028
Ogbodo, U. C., Enejoh, O. A., Okonkwo, C. H., Gnanasekar, P., Gachanja, P. W., Osata, S., et al. (2023). Computational identification of potential inhibitors targeting cdk1 in colorectal cancer. Front. Chem. 11, 1264808. doi:10.3389/fchem.2023.1264808
Oh, E. T., Kim, H. G., Kim, C. H., Lee, J., Kim, C., Lee, J. S., et al. (2023). NQO1 regulates cell cycle progression at the G2/M phase. Theranostics 13, 873–895. doi:10.7150/thno.77444
Park, J. W., Chang, H. J., Yeo, H. Y., Han, N., Kim, B. C., Kong, S. Y., et al. (2020). The relationships between systemic cytokine profiles and inflammatory markers in colorectal cancer and the prognostic significance of these parameters. Br. J. Cancer 123, 610–618. doi:10.1038/s41416-020-0924-5
Racané, L., Ptiček, L., Fajdetić, G., Tralić-Kulenović, V., Klobučar, M., Kraljević Pavelić, S., et al. (2020). Green synthesis and biological evaluation of 6-substituted-2-(2-hydroxy/methoxy phenyl)benzothiazole derivatives as potential antioxidant, antibacterial and antitumor agents. Bioorg Chem. 95, 103537. doi:10.1016/j.bioorg.2019.103537
Rolon, M., Hanna, E., Vega, C., Coronel, C., Dea-Ayuela, M. A., Serrano, D. R., et al. (2022). Solid nanomedicines of nifurtimox and benznidazole for the oral treatment of Chagas disease. Pharmaceutics 14, 1822. doi:10.3390/pharmaceutics14091822
Steinbrueck, A., Sedgwick, A. C., Brewster, J. T., Yan, K. C., Shang, Y., Knoll, D. M., et al. (2020). Transition metal chelators, pro-chelators, and ionophores as small molecule cancer chemotherapeutic agents. Chem. Soc. Rev. 49, 3726–3747. doi:10.1039/c9cs00373h
Sung, H., Ferlay, J., Siegel, R. L., Laversanne, M., Soerjomataram, I., Jemal, A., et al. (2021). Global cancer statistics 2020: GLOBOCAN estimates of incidence and mortality worldwide for 36 cancers in 185 countries. CA Cancer J. Clin. 71, 209–249. doi:10.3322/caac.21660
Tang, L., Hamid, Y., Liu, D., Shohag, M. J. I., Zehra, A., He, Z., et al. (2020). Foliar application of zinc and selenium alleviates cadmium and lead toxicity of water spinach - bioavailability/cytotoxicity study with human cell lines. Environ. Int. 145, 106122. doi:10.1016/j.envint.2020.106122
Wang, X., Wu, K., Fang, L., Yang, X., Zheng, N., Du, Z., et al. (2021). Discovery of N-substituted sulfamoylbenzamide derivatives as novel inhibitors of STAT3 signaling pathway based on Niclosamide. Eur. J. Med. Chem. 218, 113362. doi:10.1016/j.ejmech.2021.113362
Wu, Q., Qian, W., Sun, X., and Jiang, S. (2022). Small-molecule inhibitors, immune checkpoint inhibitors, and more: FDA-approved novel therapeutic drugs for solid tumors from 1991 to 2021. J. Hematol. Oncol. 15, 143. doi:10.1186/s13045-022-01362-9
Wu, Q., Tan, L., Ren, X., Fu, C., Chen, Z., Ren, J., et al. (2023). Metal-organic framework-based nano-activators facilitating microwave combined therapy via a divide-and-conquer tactic for triple-negative breast cancer. ACS Nano 17, 25575–25590. doi:10.1021/acsnano.3c09734
Xin, Y., Chen, S., Tang, K., Wu, Y., and Guo, Y. (2022). Identification of nifurtimox and chrysin as anti-influenza virus agents by clinical transcriptome signature reversion. Int. J. Mol. Sci. 23, 2372. doi:10.3390/ijms23042372
Keywords: organic synthesis, benzothiazole derivatives, anticancer, antiinflammatory, biological evaluation
Citation: Xu X, Zhu Z, Chen S, Fu Y, Zhang J, Guo Y, Xu Z, Xi Y, Wang X, Ye F, Chen H and Yang X (2024) Synthesis and biological evaluation of novel benzothiazole derivatives as potential anticancer and antiinflammatory agents. Front. Chem. 12:1384301. doi: 10.3389/fchem.2024.1384301
Received: 09 February 2024; Accepted: 28 February 2024;
Published: 18 March 2024.
Edited by:
Mehlika Dilek Altıntop, Anadolu University, TürkiyeCopyright © 2024 Xu, Zhu, Chen, Fu, Zhang, Guo, Xu, Xi, Wang, Ye, Chen and Yang. This is an open-access article distributed under the terms of the Creative Commons Attribution License (CC BY). The use, distribution or reproduction in other forums is permitted, provided the original author(s) and the copyright owner(s) are credited and that the original publication in this journal is cited, in accordance with accepted academic practice. No use, distribution or reproduction is permitted which does not comply with these terms.
*Correspondence: Faqing Ye, yfq664340@163.com; Huijun Chen, chjtzs@163.com; Xiaojiao Yang, yxj9597@wmu.edu.cn
†These authors have contributed equally to this work