Two Distinct Back-Arc Closure Phases of the East Sea: Stratigraphic Evidence From the SW Ulleung Basin Margin
- 1Department of Geological Sciences, Pusan National University, Busan, South Korea
- 2Department of Earth and Marine Sciences, Jeju National University, Jeju, South Korea
- 3Petroleum & Marine Research Division, Korea Institute of Geoscience and Mineral Resources, Dajeon, South Korea
- 4Domestic Exploration Department, Korea National Oil Corporation, Ulsan, South Korea
This study focuses on revisiting the tectostratigraphic framework of the Ulleung Basin and conceptualizing neotectonics around the western East Sea margin. Based on the analysis of 2D and 3D multi-channel seismic reflection data and offshore drill wells, we divided the entire sedimentary successions of the Ulleung Basin into four tectostratigraphic sequences, named TS1 (c. 23–16 Ma), TS2 (c. 16–9 Ma), TS3 (c. 9–4 Ma), and TS4 (c. 4 Ma–present), in ascending order. The results show that each sequence has been deformed once or multiple times in different periods by juxtaposing two major compressional structures named the Dolgorae Thrust-Fold Belt and the Gorae Anticline. Interpretation of the stratal deformation and termination patterns of the syn- and post-deformational sequences of each structures suggests that the thrusting and folding of the Dolgorae Thrust-Fold Belt was active from c. 16 Ma to c. 9 Ma under the NNW–SSE compressional stress regime (Stage-2), whereas the Gorae Anticline was active from 4 Ma to the present under the ENE–WSW compressional stress regime (Stage-4). Between these two compressional events, there was an intervening period of regional slow subsidence driven by thermal contraction of the back-arc lithosphere and isostatic sedimentary loading (Stage-3). Based on the stratigraphic and structural reconstruction, we propose a 4-stage tectonic model: Stage-1) back-arc opening stage associated with the southward drift of the Japanese islands (c. 23–16 Ma), Stage-2) tectonic-inversion stage in association with the reorganization of the Pacific and Philippine Sea plates and clockwise rotation of SW Japan (c. 16–9 Ma), Stage-3) post-inversion stage with regional thermal and isostatic subsidence (c. 9–4 Ma), and Stage-4) neotectonic stage in which embryonic subduction is nucleating on the East Sea margins under the E–W compressional stress regime (c. 4 Ma–present).
1 Introduction
The East Sea (Japan Sea) is a semi-enclosed marginal sea formed by the Cenozoic back-arc extension behind the Japanese Island Arc (Tamaki, 1988). Dextral pull-apart style extension with associated rotation of the southwestern Japanese Island Arc resulted in the characteristic parallelogramic geomorphological evolution of the East Sea (Lallemand and Jolivet, 1986; Jolivet, et al., 1991). Around 15 Ma, the young Philippine Sea Plate moving along the Ryukyu Trench reached south of present-day Kyushu in Japan by replacing the southern end of the pre-existing Pacific Plate (Hall, 2002). This plate reorganization caused cessation of back-arc extension (Jolivet and Tamaki, 1992; Lee et al., 2011), leaving behind a regional compressional structure on the southwestern margin of the East Sea (Lee et al., 1999; Kim et al., 2008). At present, the entire East Sea and surrounding regions are under the influence of the E–W compressional stress that started around 4 Ma (Taira, 2001). This stress regime appears to convert the strike-slip margins of the sea into compressional zones, which potentially evolve into incipient subduction zones (Tamaki and Honza, 1985; No et al., 2014; Gi-Bom Kim et al., 2018).
The Ulleung Basin, located in the southwest of the East Sea, was one of the three back-arc opening centers in the East Sea along with the Japan, and Yamato basins (Yoon and Chough, 1995). On the southern margin of the basin, over 10 km thick sedimentary successions preserve superimposed stratigraphic records of subsidence/uplift and structural deformations in association with the back-arc opening and subsequent tectonic inversions throughout the Miocene and post-Miocene periods. In particular, the two major compressional structures of this region, the Dolgorae Thrust-Fold Belt (DTFB) and the Gorae Anticline, might be markers for the two important compressional tectonic events initiated in the Middle Miocene and Pliocene, respectively (Yoon et al., 2003; Kim et al., 2008). However, the structures have not yet been properly investigated in terms of their activation periods and relations with changing background tectonics. Such a rough tectostratigraphic conception of this region hinders a proper understanding of the neotectonic nature of the East Sea and the Korean Peninsula.
In this study, we propose a new 4-stage tectostratigraphic model of the Ulleung Basin to better understand the tectonic evolution of the East Sea and obtain a proper conceptualization of the neotectonics around the Korean Peninsula. For this purpose, we precisely analyzed the stratigraphic characteristics of the DTFB and the Gorae Anticline, based on the entire large amounts of 2D and 3D multi-channel seismic reflection data of the southwestern marginal region of the East Sea provided by the Korea National Oil Corporation (KNOC). To control the age of newly defined sequence boundaries, we used foraminiferal biostratigraphy constructed based on six deep drill cores recovered in this region (Yi et al., 2020).
2 Regional Setting
The East Sea is a semi-enclosed marginal sea or back-arc basin located in northeastern Asia (Figure 1) (Chough et al., 2000). The sea comprises three sedimentary basins, namely the Japan, Yamato, and Ulleung basins, that are demarcated by three topographic highs, namely the Korea Plateau, the Yamato Rise, and the Oki Bank (Figure 1A). It is broadly accepted that the back-arc extension of the East Sea was initiated at the Early Oligocene (c. 32 Ma) (Tamaki, 1986; Ingle, 1992). Lallemand and Jolivet (1986) proposed that two dextral shear zones along the eastern and western margins of the sea guided the pull-apart style back-arc opening.
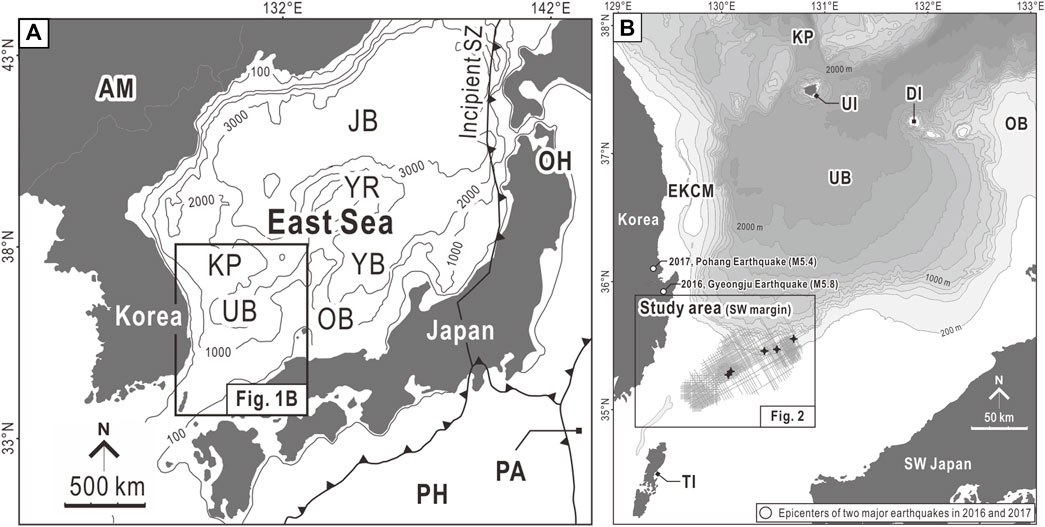
FIGURE 1. (A) Map showing the physiography and plate locations around the East Sea. (B) Bathymetric map of the Ulleung Basin showing the location of the study area. AM, Amur Plate; PH, Philippine Sea Plate; PA, Pacific Plate; OH, Okhotsk Plate; JB, Japan Basin; YB, Yamato Basin; UB, Ulleung Basin; YR, Yamato Rise; OB, Oki Bank; KP, Korea Plateau.
The pull-apart back-arc opening continued until the Middle Miocene (c. 15 Ma) when the Philippine Sea Plate started to induce clockwise pivotal rotation of the southwestern Japan (Jolivet and Tamaki, 1992; Hall, 2002). The rotation resulted in regional compressional deformation over the southwestern margin of the East Sea, which created Tsushima Island and Dolgorae-San’in Thrust-Fold Belts (Chough and Barg, 1987; Yamamoto, 1993; Fabbri et al., 1996; Kim et al., 2008). The reconstruction of the subsidence history from the ODP Leg 127/128 suggests that the clockwise rotation of the southwestern Japanese islands ceased in the late Middle Miocene (c. 12 Ma). Thereafter, the entire East Sea subsided slowly by the thermal contraction of the back-arc lithosphere until the onset of the post-Miocene neotectonic phase of the sea (Ingle, 1992; Jolivet and Tamaki, 1992; Taira, 2001).
Previous studies have summarized the neotectonics of the East Sea as an incipient subduction system, where the back-arc crust of the East Sea (the eastern margin of the Amur Plate) thrusts down below the northeastern Japanese islands (the Okhotsk Plate or the western end of the North American Plate) Nakamura, 1983; Tamaki and Honza, 1985; Lallemand and Jolivet, 1986; Jolivet and Tamaki, 1992; No et al., 2014). Lines of evidence, including the M 6.9–7.7 five earthquakes from 1940 to 1983, east-dipping and linear aftershock distribution, and p-wave velocity structures, strongly support rupture of the lithosphere and incipient subduction along the eastern margin of the East Sea (Tamaki and Honza, 1985; Sato et al., 1986). The uplifting terraces on western Honshu are also circumstantial evidence of subduction initiation (Okada and Ikeda, 2012).
In contrast to the eastern margin of the East Sea, where a new subduction zone is initiating, the western counterpart has long been regarded as a tectonically inactive intraplate setting, despite evidence of active compressional tectonics, such as coastal terraces (Choi et al., 2008), frequent earthquakes (Choi et al., 2012), and Quaternary faults along the onland and offshore regions of the eastern Korean Peninsula (Han-Joon Kim et al., 2016; Cheon et al., 2020). Such a perception began to change since the Magnitude 5.8 and 5.4 earthquakes in 2016 and 2017 respectively (YoungHee Kim et al., 2016; Kwang-Hee Kim et al., 2018). Gi-Bom Kim et al. (2018) suggested the possibility of creating an incipient subduction zone with associated crustal buckling along the western margin of the East Sea.
The Ulleung Basin, located in the southwestern corner of the East Sea, is a deep marine basin (over 2,000 m) (Figure 1B), filled with sedimentary sequences with a total thickness of ∼10 km in the southern part and ∼5 km in the northern part. The tectonic evolution of the Ulleung Basin was divided into two stages. The first stage was initiated in the Early Miocene when the rhombic-shaped Ulleung Basin was constructed via a pull-apart-style back-arc extension that associated two dextral strike-slip principal deformation zones on the western and eastern boundaries (Yoon and Chough, 1995). Kim and Yoon (2017) proposed a simple-shear-style lithospheric extension model that explains juxtaposed asymmetric features in syn-extensional volcanism, fault pattern, sediment thickness, and crustal structure. The back-arc extension of the Ulleung Basin ceased in the Middle Miocene (c. 15 Ma), when the background stress regime was inverted from NNW–SSE extension to NNW–SSE compression in association with the rotation of the southwestern Japanese islands (Kim et al., 2008; Lee et al., 2011; Yoon et al., 2014). The tectonic inversion induced regional compressional deformation of the southwestern margin of the Ulleung Basin and constructed the Tsushima and Goto islands and Dolgorae-San’in Thrust-Fold belts (Yamamoto, 1993; Fabbri et al., 1996; Kim et al., 2008).
Hitherto, this two-stage tectonic scenario is most widely referred to as the evolutionary framework of the Ulleung Basin (Bahk et al., 2017; Cukur et al., 2018; Park et al., 2019; Horozal et al., 2021; Park et al., 2021). However, the scenario omits the final tectonic phase of the East Sea, marked by the nucleation of a new embryonic subduction system under E–W compressional stress since the Early Pliocene.
3 Data and Methods
To reconstruct the tectostratigraphic evolution of the southwestern margin of the Ulleung Basin, we analyzed the stratal termination pattern, seismic facies, and deformation structures of the Neogene sedimentary succession based on 2D (a total of 6,448 line-km) and 3D multi-channel seismic reflection dataset acquired by the KNOC in 1983, 1988, 1989, 1992, 2005, and 2006 (Figure 2). The migrated seismic reflection data were fully digitized into SEG-Y format and uploaded to the PETREL™ (Schlumberger, Ltd., France) software for defining stratigraphic boundaries, correlating absolute ages, tracing the 3-dimensional geometry of the deformation structures, and creating time-structural and isochronal maps.
4 Tectostratigraphy of the Southwestern Ulleung Basin
For the last 40 years, considerable efforts have been made to understand the structural evolution and depositional history of the southwestern Ulleung Basin. Yet, the stratigraphic framework of this region is not in consensus (Figure 3). Yi et al. (2020) recently proposed an integrated chronostratigraphic framework of this region based on microfossil analysis of recovered sediment cores and correlation of drill wells with 2D and 3D multi-channel seismic reflection data (Figure 3). The new stratigraphic model divides the entire sedimentary succession of the southwestern Ulleung Basin into 20 chronostratigraphic units. We followed this framework.
In this study, we regrouped the 20 units into four tectostratigraphic sequences based on changes in deposition trends and characteristics of regional deformation, which are entitled TS1, TS2, TS3, and TS4, respectively (Figure 4). The respective boundaries of these sequences are named SB1, SB2, and SB3, which are coeval with the boundaries, MM10_SB (c. 16 Ma), UM30_SB (c. 9 Ma), and PL20_SB (c. 4 Ma), proposed by Yi et al. (2020) (Figure 3). The terminology used to describe the external forms and reflection configurations of the tectostratigraphic sequences of this study follows Mitchum et al. (1977).
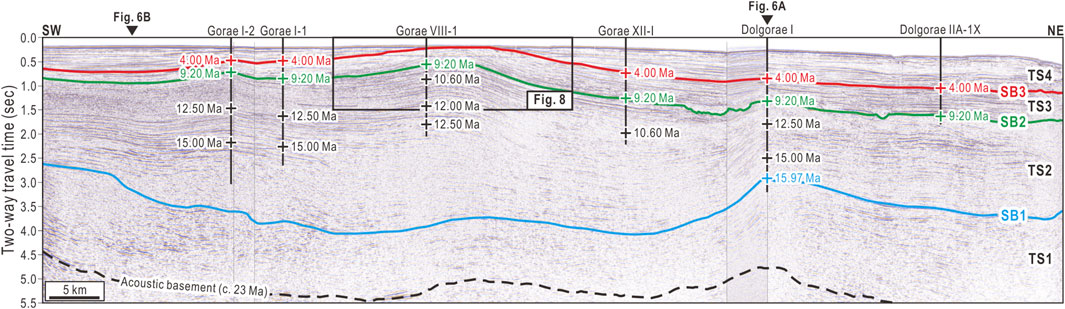
FIGURE 4. NE–SW composite seismic reflection profile showing four tectostratigraphic sequences and ages of three sequence boundaries. For the locations of the seismic profile, see Figure 2. TS: Tectostratigraphic Sequence, SB: Sequence Boundary.
4.1 TS1 (c. 23? –16 Ma)
TS1 is the lowermost tectostratigraphic sequence of the Ulleung Basin, which as a whole exhibits a wedge-shaped fill-type external form (Mitchum et al., 1977) (Figure 5). The sequence gradually thickens toward the center of the basin with thickness ranging from 0.5 to 2.0 s TWT (two-way traveltime). On the southwestern slope of the Ulleung Basin, TS1 comprises discontinuous high-amplitude reflectors with lenticular or mounded depositional structures (Figure 5). These stratal characteristics are interpreted as accumulated submarine lobe or deep-sea fan deposits transported by high-concentration sediment gravity-flows, such as submarine debris flows (Weimer, 1990).
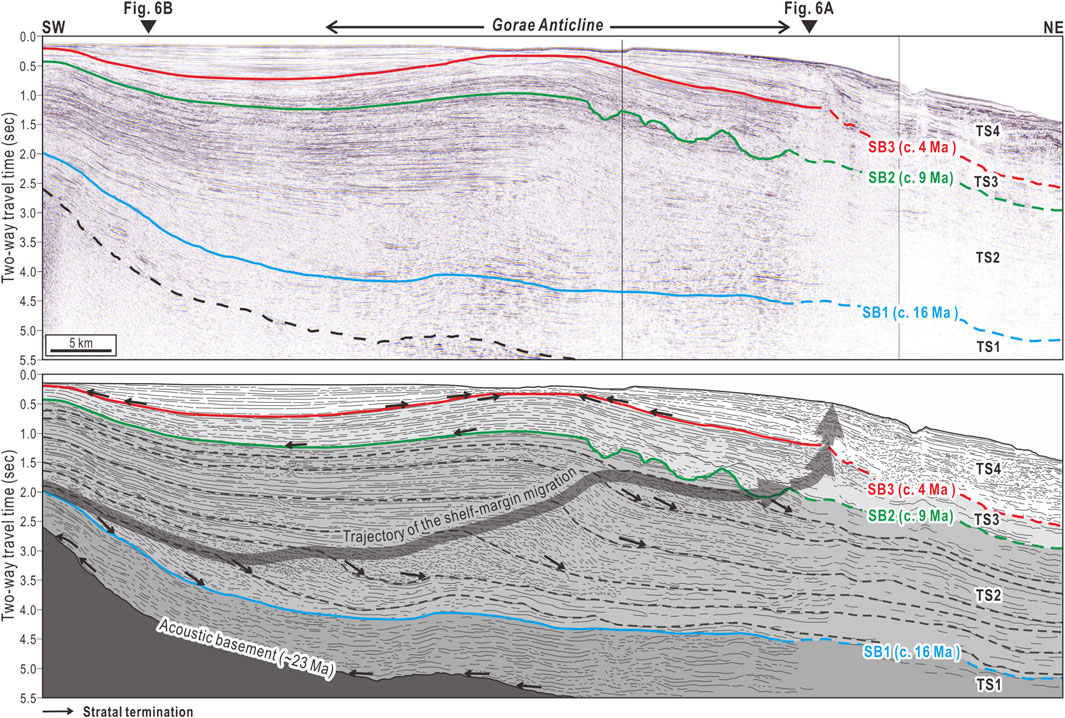
FIGURE 5. NE–SW composite seismic reflection profile showing stratal configurations and external forms of the four tectostratigraphic sequences. For the locations of the seismic profile, see Figure 2. TS: Tectostratigraphic Sequence, SB: Sequence Boundary.
Toward the center of the basin, the proximal debris flow facies changes into distal dilute sediment gravity-flow facies, such as turbidite, marked by continuous, and subparallel reflectors with low reflection amplitudes (Figure 5) (Weimer, 1990). The strata in the lower part of TS1 show onlap termination against the slope of the acoustic basement (Figure 5). The lateral facies transition and lap-out character suggest that the TS1 comprises submarine mass-transport succession deposited in deep-sea-slope and basin-floor environments during the syn-rift phase of the Ulleung Basin evolution.
The acoustic basement under TS1 has a topography gradually gentle toward the center of the Ulleung Basin, below which the reflection character is faintly stratified or chaotic (Figure 5). Such seismic reflection facies are interpreted as rifted continental crust comprising the Jurassic–Cretaceous extrusive/intrusive/accretionary complex (Chough and Sohn. 2010), and syn-rift volcanic/sedimentary complexes (Yoon et al., 2003). SB1, the upper boundary of TS1, is correlated with MM10_SB (c. 16 Ma) (Figure 3).
4.2 TS2 (c. 16–9 Ma)
TS2 is the thickest tectostratigraphic sequence of the Ulleung Basin, complicated by syn-depositional thrust faults and folds (Figure 6). The thrusts and folds generally have ENE–WSW strikes and as a whole comprise a regional belt of thrust faults and folds, named DTFB, in the southern part of the study area (Figure 7). The stratal characteristics and external form of the TS2 within the domain of the DTFB are substantially different from those found in the northwestern frontal area of the DTFB (Figure 7A).
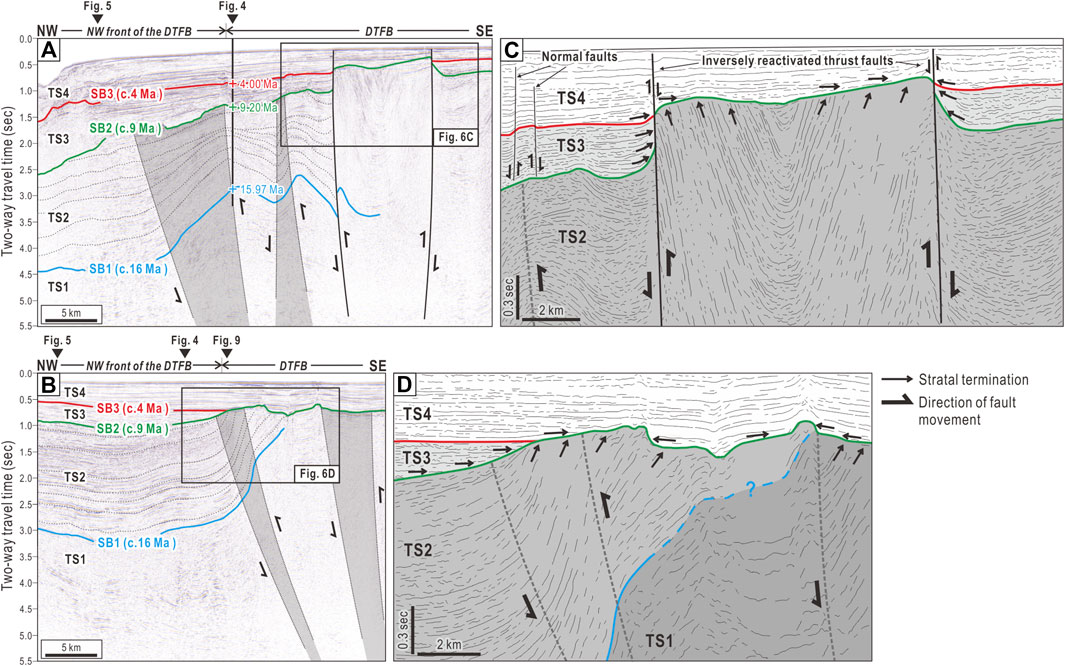
FIGURE 6. Selected NW–SE seismic reflection profiles showing the Dolgorae Thrust-Fold Belt (DTFB). For the locations of the seismic profiles, see Figure 2. TS: Tectostratigraphic Sequence, SB: Sequence Boundary.
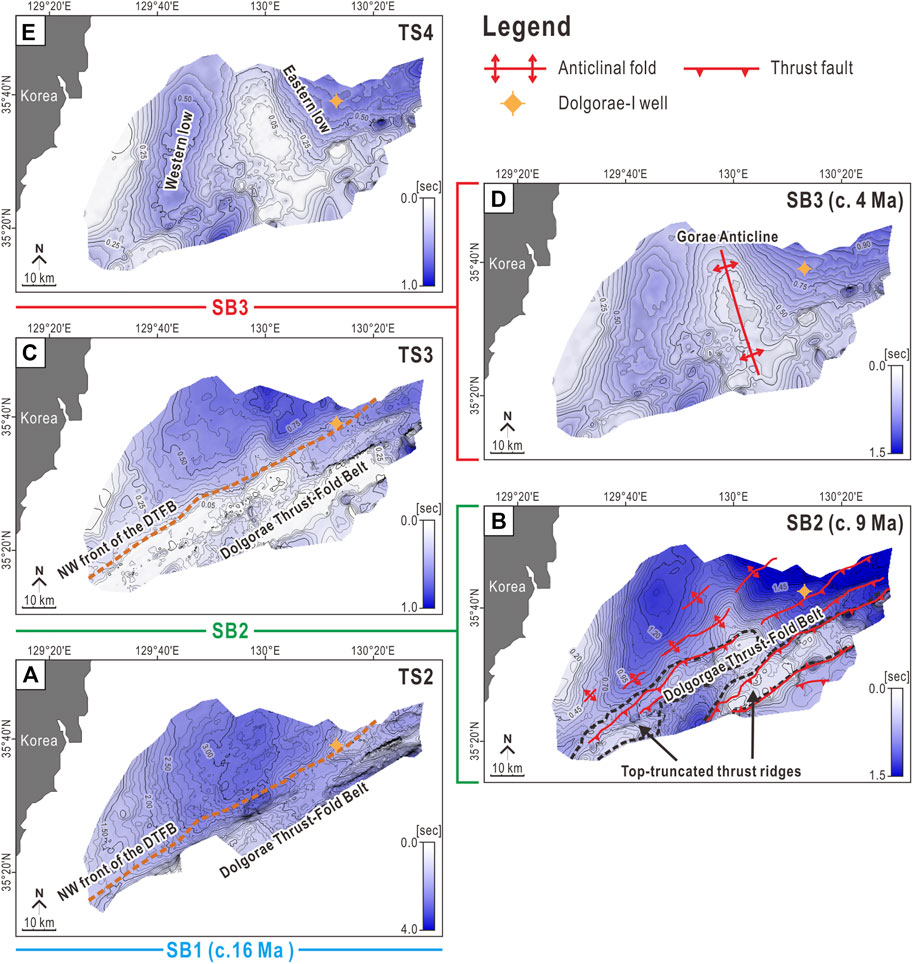
FIGURE 7. (A), (C), and (E) Isochronal maps showing spatial thickness variations of the TS2, TS3, and TS4. (B) and (D) Time-structural maps showing the topography of the SB2 and SB3. The thickness of the TS1 is not mappable due to poor definition of the acoustic basement and the SB1 in the study area. TS: Tectostratigraphic Sequence, SB: Sequence Boundary.
Within the DTFB, the original external form and stratal configurations of TS2 are destroyed by densely populated and imbricated thrust faults and folds (Figures 6A,B). Reflectors in this region show high-angle inclinations and truncational terminations against the upper boundary of the sequence (SB2) (Figures 6C,D). Such an erosional feature indicates shallow-marine wave planation of sedimentary substratum that emerged above sea level by compressional tectonic uplift (Kim et al., 2013). The top-truncational surfaces are situated on top of the two thrust ridges elongated more than 70 km toward the ENE in the study area (Figure 7B). Such a regional array of thrust ridges that accommodated a large amount of vertical tectonic uplift is identical to those found in the orogens and accretionary wedges (Miall, 1995).
On the northwestern front of the DTFB, TS2 comprises minorly deformed, continuous, and high-amplitude reflectors with divergent stratal configuration, indicating growth strata (Figures 6A,B). In this area, the sequence has a maximum thickness of approximately 3.5 s TWT and exhibits a slope-front-fill external form (Mitchum et al., 1977) (Figure 6B). Toward the basin center, the strata show a sigmoid-oblique progradational configuration (Mitchum et al., 1977), clearly divided into topset, foreset, and bottomset (Figure 5). Such a stratal character is interpreted as a prograding sedimentary wedge developed in a shelf-margin environment (Porębski and Steel, 2003). The aggradation/progradation ratio (A/P ratio) of the prograding wedge progressively increases throughout TS2, but abruptly decreases near the top of the sequence (Figure 5). Such a variation in the A/P ratio suggests accelerated relative sea level rise and accommodation creation during the early to middle depositional stages of the TS2, followed by abrupt deceleration of both in the terminal stage. SB2, the upper boundary of TS2, marks the end of the progradational deposition of TS2. The boundary is correlated with the UM30_SB (c. 9 Ma) of the existing chronostratigraphic framework (Figure 3).
4.3 TS3 (c. 9–4 Ma)
TS3 is the Late Miocene tectostratigraphic sequence of the Ulleung Basin, showing variable fill-type external forms. Within the domain of the DTFB, the TS3 fills topographic lows between ENE–WSW elongated thrust ridges with a maximum thickness of approximately 0.4 s TWT (Figure 7C). In this region, the strata composing the interior of the TS3 do not show any divergent configurations, but they show parallel alignment with high-angle onlap termination against fault scarps and fold limbs of the DTFB (Figures 6C,D). This stratal character suggests that TS3 was deposited not during but after the structural deformation of the DTFB.
On the northwestern front of the DTFB, TS3 has a wedge-shaped external form (Mitchum et al., 1977) that gradually thickens toward the northeast (Figures 4, 5). In contrast to the underlying TS2, the TS3 in this region comprises strata not progradational but parallel, which terminates at a low angle against the lower boundary, SB2 (Figure 5). Ubiquitous channels are found near the base of TS3. The channels are generally 1–4 km wide and scour the marginal topset of the uppermost TS2 with a maximum erosional depth of 500 m (Figure 5). Such an erosional structure is interpreted as a shelf-margin channel formed by underloaded subaqueous sediment gravity-flows generated under condition of decreased sediment supply associated with relative sea level rise (Catuneanu, 2006; Arnott, 2010).
The upper boundary of the TS3 (SB3), correlated with the PL20_SB (c. 4 Ma) (Figure 3), has a conformable relationship with the overlying TS4 in almost all locations of the study area (Figures 4, 5), except for the top of the NNW–SSE elongated anticlinal fold, named Gorae Anticline (Figure 7D).
4.4 TS4 (c. 4 Ma–present)
TS4 is the uppermost tectostratigraphic sequence of the Ulleung Basin that divergently fills two topographic lows to the west and east of the Gorae Anticline (Figures 8, 9). TS4 is in a lenticular-fill external form on the west of the Gorae Anticline with a maximum thickness of approximately 0.5 s TWT, whereas in a wedge form on the east of the anticline, the thickness gradually increases toward the center of the Ulleung Basin (Figure 7E). Some of thrust faults in the DTFB extend upward and separate the strata of the TS3 and TS4 with inversed sense compared with that of the underlying TS2 (Figure 6C). This feature indicates that the thrust faults in the DTFB were normally reactivated during the deposition of the TS4 and possibly the TS3. The TS4 showing pronounced divergent-fill stratal configuration is interpreted as growth strata, suggesting that TS4 is the syn-deformational sedimentary sequence of the Gorae Anticline.
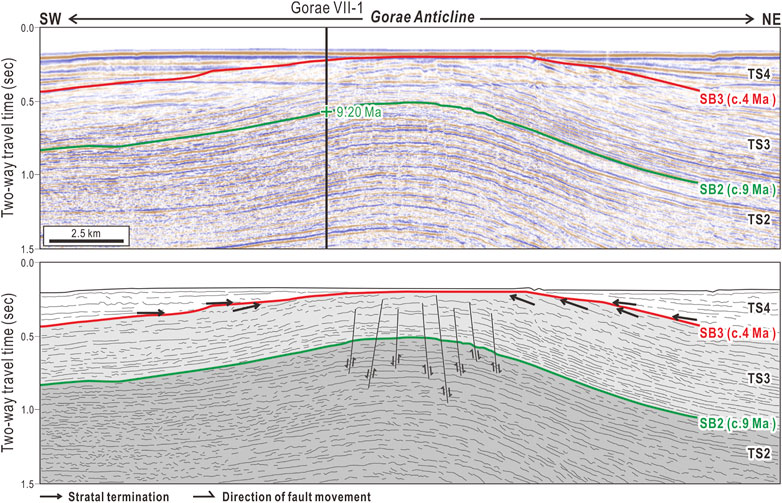
FIGURE 8. Magnified NE–SW seismic reflection profile showing the details of the Gorae Anticline. For the position of the seismic profile, see Figure 4. TS: Tectostratigraphic Sequence, SB: Sequence Boundary.
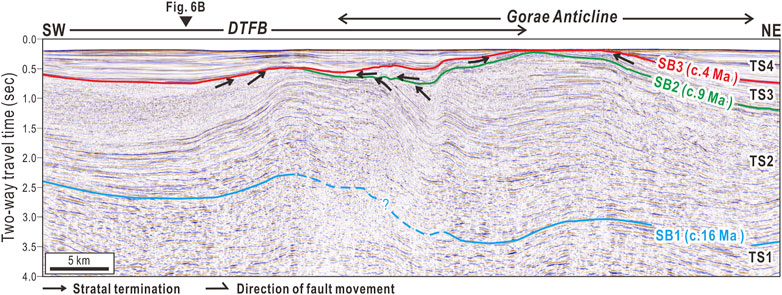
FIGURE 9. Selected NE–SW seismic reflection profile showing the superposed feature of the DTFB and the Gorae Anticline. Two syn-deformational sequences (TS2 and TS4) of each structures are separated by intervening TS3 deposited during the Late Miocene. For the locations of the seismic profile, see Figure 2. TS: Tectostratigraphic Sequence. SB: Sequence Boundary.
5 Discussion
5.1 Stratigraphic Implications of the DTFB
We investigated the geometry and duration of the DTFB and Gorae Anticline based on the deformation and termination patterns of sedimentary strata in the syn- and post-deformational sequences of each structures. One of our key findings is that the DTFB and Gorae Anticline were formed during different periods under different tectonic settings.
The DTFB, comprising thrust faults and fault-propagation folds dipping southeastward, deforms TS1 and TS2 on the southwestern margin of the Ulleung Basin (Figure 6). The duration of structural deformation in the DTFB is difficult to constrain based only on seismic reflection analysis because densely populated thrust faults and folds disrupt the original sedimentary strata of the pre-and syn-deformational sequences. However, paleomagnetic and structural evidence found in the Korean Peninsula and the Tsushima-Goto islands region suggests that the DTFB started to develop at approximately 15 Ma (Kim, 1992; Fabbri et al., 1996; Lee et al., 1999; Kim et al., 2008). For the tectonic control of this regional compressional deformation, a hypothesis of pivotal rotation of SW Japan by the northeastward migration of the young Philippine Sea Plate during the early Middle Miocene was proposed (Kim, 1992; Lee et al., 1999). The pivotal rotation resulted in a dramatic change in tectonic stress from NNW–SSE extensional to NNW–SSE compressional. We entitle this period of tectonic regime change as the “tectonic-inversion stage” (Figure 10).
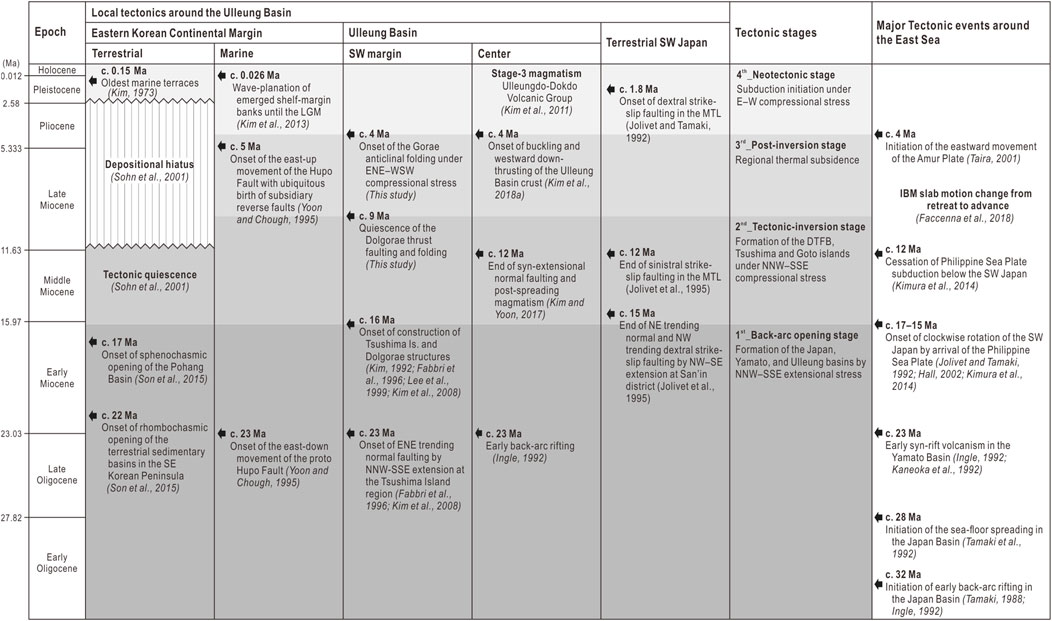
FIGURE 10. Stratigraphic chart showing major tectonic events in the Ulleung Basin and surrounding regions. MTL: Median Tectonic Line, IBM: Izu-Bonin-Mariana.
Fabbri et al. (1996) suggested that the compressional deformation of the southwestern Ulleung Basin continued until approximately 10 Ma based on fault fracture analysis on the outcrop and radiometric ages of extrusive alkaline basalts that overlie the syn-deformational sedimentary units. This age constraint corresponds well with our results showing that the thrust faults of the DTFB cutting the TS1 and TS2 do not further deform the overlying TS3 deposited since c. 9 Ma (Figures 6, 9). The parallel strata with high-angle onlap termination against fault scarps and fold limbs of the DTFB indicate that TS3 is a post-compressional sedimentary sequence that fills the topography of SB2.
The flattened top of the thrust ridges situated along the center of the DTFB is interpreted to have formed by wave planation of the emerged thrust blocks (Kim et al., 2013). These time-transgressive erosional surfaces are correlated with SB2 (c. 9 Ma) in the northwestern frontal area of the DTFB (Figures 6A,B). This suggests that the wave planation of the emerged thrust blocks ceased at approximately 9 Ma.
5.2 Gorae Anticline: A Product of Compressional Neotectonics on Eastern Eurasia
The Gorae Anticline is a typical symmetrical fold with a near-vertical axial plane oriented ∼ N22W (Figure 9C). The fold deforms the entire sedimentary succession below SB3 (c. 4 Ma). This indicates that anticlinal deformation initiated at approximately 4 Ma. The TS4 around the Gorae Anticline has a typical growth stratal configuration, which implies that the deformation is in progress during the Quaternary period.
The tectonic control underlying the Gorae Anticline remains unsettled. However, given the age and fold-axis orientation, the anticlinal fold is inferred to have a genetic relationship with the major thrust and crustal buckles in the Ulleung Basin, which may imply embryonic subduction initiated in the Early Pliocene (Gi-Bom Kim et al., 2018). Gi-Bom Kim et al. (2018) proposed that the regional E–W compressional far-field stress predominated over eastern Eurasia since the Early Pliocene induced crustal shortening and embryonic subductions along the western and eastern margins of the East Sea. We envisage that the Gorae Anticline, located on the southwestern margin of the Ulleung Basin, is also a product of the compressional neotectonics governing eastern Eurasia since the Early Pliocene.
5.3 Post-Inversion Tectonic Quiescence
Tectonic stresses gradually deform the topography of the sedimentary basins over time. The progressive increase in sedimentary accommodation by tectonic deformation results in a characteristic style of stratal configuration, the so-called growth strata. If the tectonic event is over, subsequent sediment deposition proceeds in a way that sediments simply fill the topography, leaving behind parallel onlap-fill stratal configurations. Thus, people utilize the seismic facies boundary between the growth and onlap-fill stratal configurations as a marker of the cessation of a certain tectonic event (Shaw et al., 2005).
The TS3 overlying the growth strata of TS2 shows apparent parallel onlap-fill stratal patterns (Figure 6). Reverse faults and fault-tip folds of the DTFB are no longer found in this sequence. Such lines of evidence indicate that TS3 was deposited after the cessation of the preceding compressional tectonics. The TS3 as a whole gradually thickens toward the center of the Ulleung Basin without any progradational sedimentary feature (Figures 4, 5). This infers a slow and consistent sagging of the entire southwestern Ulleung Basin region, most likely due to the combined influence of sedimentary loading and thermal contraction of the back-arc lithosphere. We entitle this quiescent tectonic stage from 9 to 4 Ma to the “post-inversion stage” (Figure 10). In this period, the motion of the Izu-Bonin- Mariana (IBM) slab was changed from retreat to advance (Faccenna et al., 2018). We infer that this post-inversion tectonic quiescence is genetically related with the cessation of the Philippine Sea Plate subduction below the SW Japan (Kimura et al., 2014).
The subsidence rates during this post-inversion stage of the East Sea, calculated from the ODP and DSDP cores on the East Sea margins, range from 50 to 100 m/Myr, which is significantly lower than the subsidence rate during the period of the Miocene back-arc extension (>900 m/Myr) (Ingle, 1992). The paleobathymetric data of the Dolgorae-1 well shows that the uplift of the DTFB was terminated at approximately 9 Ma, which was followed by slow subsidence (∼100 m/Myr in total subsidence rate) continued until the present (Figure 11) (Chough and Barg, 1987; Ingle, 1992).
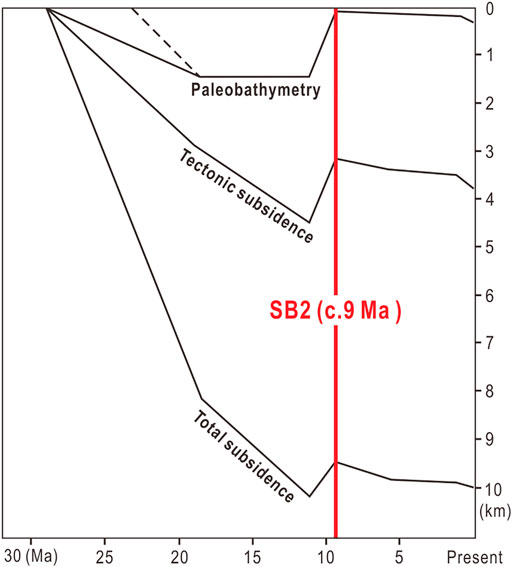
FIGURE 11. Subsidence curves recovered from paleobatymetric data of the Dolgorae-I exploratory well (modified after Chough and Barg, 1987 and Ingle, 1992). Total subsidence is the sum of the thermotectonic subsidence, isostatic sedimentary loading, and sediment compaction. Note: the red line marks the inflection point of subsidence rate from negative to positive. The abrupt change in subsidence rate implies that the uplifting of the DTFB was ceased at c. 9 Ma.
5.4 A New 4-Stage Tectonic Model of the Ulleung Basin
Previous seismic reflection studies of the southwestern Ulleung Basin have interpreted the Gorae anticlinal folding as a continuous deformation feature of the DTFB (Lee et al., 2011; Yoon et al., 2014). Based on this structural interpretation, the tectonic evolution of the Ulleung Basin was divided into two phases: the back-arc opening phase (c. 23–16 Ma) and the back-arc closure phase (after c. 16 Ma). In this study, we report that the DTFB and the Gorae Anticline are temporally and tectonically separable events with an intervening phase of tectonic quiescence lasting for more than 5 Myr. Based on this new structural interpretation, we propose a new 4-stage tectonic model of the Ulleung Basin (Figure 12).
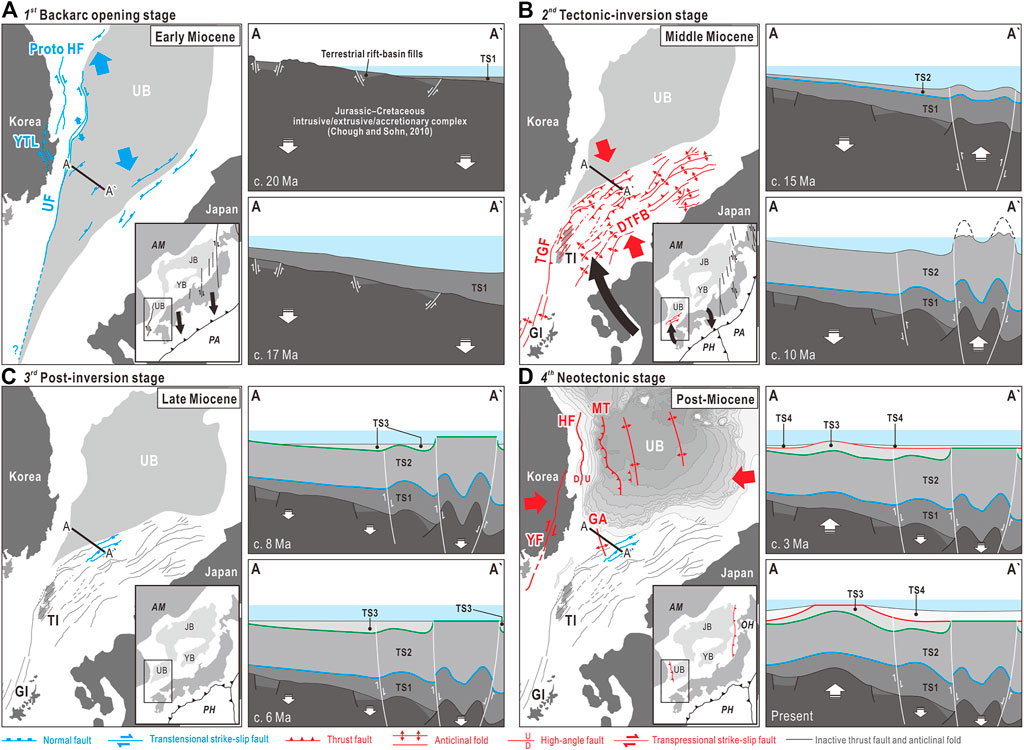
FIGURE 12. Four-stage tectonic model of the Ulleung Basin showing details of structural characteristics in association with changes in relative locations of the surrounding tectonic plates. (A) Back-arc opening stage (c. 23–16 Ma). (B) Tectonic-inversion stage (c. 16–9 Ma). (C) Post-inversion stage (c. 9–4 Ma). (D) Neotectonic stage (c. 4 Ma–present). AM: Amur Plate, PA: Pacific Plate, PH: Philippine Sea Plate, OH: Okhotsk Plate, UB: Ulleung Basin, YB: Yamato Basin, JB: Japan Basin, TI: Tsushima Island, GI: Goto Island, YTL: Yeonil Tectonic Line, UF: Ulleung Fault. TGF: Tsushima-Goto Fault, DTFB: Dolgorae Thrust-Fold Belt, YF: Yangsan Fault, HF: Hupo Fault, MT: Major Thrust, GA: Gorae Anticline.
5.4.1 Stage I: Back-Arc Opening Stage (c. 23–16 Ma)
The first-stage tectonic evolution of the Ulleung Basin is marked by a pull-apart-style back-arc opening during the Early Miocene (Lallemand and Jolivet, 1986). At this stage, continental rifting was guided by NNW-oriented strike-slip faults, such as the Ulleung Fault, the proto Hupo Fault, and the Yeonil Tectonic Line, together comprising the western principal deformation zone (Figure 12A) (Yoon and Chough, 1995; Yoon et al., 1997; Son et al., 2015). In the southwestern margin of the Ulleung Basin, early continental rifting began to form graben or half-graben basins on the Jurassic or Cretaceous basement (Chough and Sohn, 2010). Around 20 Ma, it appears that the entire Ulleung Basin region was converted to a marine environment (Kim et al., 2013). The study area might have been located on the southwestern lower slope of this early marine basin, where sediment gravity-flows pile up a thick syn-extensional sedimentary sequence of the TS1 (Figure 12A).
Given the Early Miocene marine sandstones and siltstones exposed on Tsushima and Goto islands (Fabbri et al., 1996), we infer that the early Ulleung Basin covered a much wider area toward the SW compared with the present realm of the basin.
5.4.2 Stage II: Tectonic-Inversion Stage (c. 16–9 Ma)
At approximately 16 Ma, the young Philippine Sea Plate migrating northeastward along the Ryukyu Trench began to replace the pre-existing Pacific Plate (Hall, 2002). The onset of a young subduction zone began to rotate the southwestern Japanese islands clockwise (Jolivet and Tamaki, 1992), which consequently induced crustal shortening in the southwestern Ulleung Basin margin. This stage of stress regime change from extension to compression is called the tectonic-inversion stage.
In this tectonic-inversion stage, a regional NNE-to ENE-trending curvilinear array of contractile structures, such as the DTFB and the Tsushima and Goto islands, were formed (Figure 12B). The TS2 deposited during the tectonic inversion was gradually compressed and uplifted to form ENE-elongated submarine thrust ridges. When the thrust ridges reached sea level, wave action began to cut and bevel the emerged summits of the ridges, and leaving behind broad wave planation surfaces (Figure 12B). Our stratigraphic correlation indicates that the compressional uplifting and wave planation of the TS2 continued until approximately 9 Ma.
Sediments originating from variable terrestrial sources and slope-failure events during the tectonic inversion were transported toward the northwestern frontal area of the DTFB, which constructed a gigantic progradational sedimentary structure (Figure 5).
5.4.3 Stage III: Post-Inversion Stage (c. 9–4 Ma)
At approximately 10 Ma, the Philippine Sea Plate appears to have arrived at the present-day position (Hall, 2002). Given the parallel onlap-fill stratal configurations of the TS3 lacking deformation by reverse fault, the inversion tectonics of the DTFB has not been active since this time. Paleobathymetric investigation of the sediment drill core recovered from the Dolgorae-I exploratory well (see Figures 2, 7 for the well location) show that the period of tectonic uplift ceased at approximately 9 Ma (Figure 12C). The significantly decreased subsidence rates thereafter (∼100 m/Myr) may suggest that a new tectonic stage of slow thermal subsidence began at this time (Chough and Barg, 1987; Ingle, 1992).
As the regional compressional stress perished at approximately 9 Ma, the DTFB and Tsushima-Goto islands began to subside slowly by isostatic loading and thermal contraction of the back-arc lithosphere. Some of the thrust faults in the DTFB began to reactivate normally (Figure 12C). Moreover, the wave planation process on the thrust ridges ceased as the southwestern Ulleung Basin region began to subside again. Sediments supplied during this post-inversion stage formed TS3 by simply filling the deformation topography.
5.4.4 Stage IV: Neotectonic Stage (c. 4 Ma–Present)
Geodetic investigations suggested that the Amur Plate initiated its present eastward movement relative to the Pacific Plate at approximately 4 Ma (Taira, 2001). This new relative plate motion since 4 Ma induced a regional E–W compressional stress over the northeastern Asia, which began to tectonically deform the East Sea and adjacent regions in a style entirely different from those during the preceding tectonic stages (Figure 12D).
Such an E–W compression made the extended back-arc crust of the Ulleung Basin buckled and thrusted down below the eastern Korean continental margin (Gi-Bom Kim et al., 2018). Along with this compressional deformation of the Ulleung Basin center, the southwestern margin began to develop the Gorae anticlinal fold. Given the identical ages (c. 4 Ma) and fold-axis orientations (∼N22W), we infer that the Gorae Anticline and the crustal buckles have a common tectonic origin.
In the eastern Korean continental margin, the proto Hupo Fault with east-down normal fault-plane geometry was reactivated in the present east-up sense (Yoon and Chough, 1995). This fault reactivation formed a wedge-shaped post-Miocene sedimentary basin, named the Hupo Basin on the offshore Korean margin (Figure 12D). The NNE–SSW trending terrestrial Yangsan Fault on the southeastern Korean Peninsula was reactivated into a dextral strike-slip fault under this Quaternary E–W compressional stress regime (Han-Joon Kim et al., 2016).
On the eastern margin of the East Sea, there have been at least five abnormally large-magnitude seismic events over M6.9 since 1940 (Tamaki and Honza, 1985). To understand the tectonic origin of such earthquakes in this marginal region, a large amount of geological and geophysical efforts has been made (Nakamura, 1983; Tamaki and Honza, 1985; Lallemand and Jolivet, 1986; Sato et al., 1986; Tamaki, 1986; Tamaki, 1988; Jolivet et al., 1991; Ingle, 1992; Jolivet and Tamaki, 1992; Jolivet et al., 1995; Taira, 2001; No et al., 2014). Based on the monitoring of large-magnitude earthquakes and their aftershock distributions, people visualize crustal-scale rupture along the boundary between NE Japan and the East Sea, and which may imply a modern subduction initiation (Sato et al., 1986; No et al., 2014). Based on geodetic and structural investigations, the northeastern Japanese islands are broadly accepted as a plate independent from the Amur Plate, and named the Okhotsk Plate (Figure 13) (Nakamura, 1983; Taira, 2001).
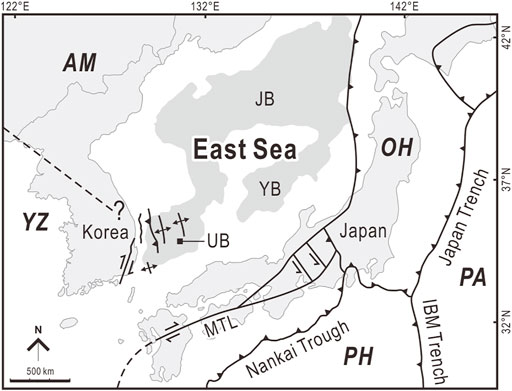
FIGURE 13. Regional map showing parallelly aligned neotectonic structures over the East Sea. AM: Amur Plate, OH: Okhotsk Plate, PA: Pacific Plate, PH: Philippine Sea Plate, YZ: Yangtze Plate, MTL: Median Tectonic Line, IBM: Izu-Bonin-Mariana, UB: Ulleung Basin, YB: Yamato Basin, JB: Japan Basin.
In contrast to the Japanese side, the Korean side of the East Sea has long been regarded as tectonic dormancy. This is largely due to the absence of large-magnitude earthquakes during the last decades in the Korean Peninsula and partly to the poor understanding of the Quaternary structures around the eastern Korean margin. However, such an old stereotype is slowly changing since two earthquakes with magnitudes of 5.8 and 5.4 occurred in 2016 and 2017, respectively, on the southeastern margin of the Korean Peninsula (YoungHee Kim et al., 2016; Kwang-Hee Kim et al., 2018). More recently, Gi-Bom Kim et al. (2018) reported crustal buckling and westward underthrusting of the Ulleung Basin, which may indicate the early stage of incipient subduction (Figure 13). The incipient subduction is very subtle and unclear as to whether it is still active. However, one thing is obvious that the eastern margin of the Korean Peninsula is not tectonically dormant, given lines of evidence, such as the Holocene marine terraces (Choi et al., 2008) and frequent moderate-magnitude seismic activities (Choi et al., 2012). We believe that our new tectostratigraphic concept will aid in understanding the nature of the Korean and East Sea neotectonics.
6 Conclusion
In this study, we investigated tectostratigraphic evolution of the southwestern Ulleung Basin margin based on the 2D and 3D multi-channel seismic reflection data set and offshore drilled wells. This research has drawn following conclusions:
1) Sedimentary successions on the southwestern margin of the Ulleung Basin are divided into four tectostratigraphic sequences (TS1, TS2, TS3, and TS4).
2) The DTFB was active from approximately 16 Ma to 9 Ma under NNW–SSE compressional stress regime.
3) The Gorae Anticline has been active from approximately 4 Ma to the present.
4) Tectonic quiescent stage existed between the formations of the DTFB and the Gorae Anticline.
4) The tectonic evolution of the Ulleung Basin is summarized into four stages: Stage-1) back-arc opening stage (c. 23–16 Ma), Stage-2) tectonic-inversion stage (c. 16–9 Ma), Stage-3) post-inversion stage (c. 9–4 Ma), and Stage-4) neotectonic stage (c. 4 Ma–present).
The post-Miocene neotectonic stage of the Ulleung Basin is first conceptualized in our study. This tectonic regime contains variable geological features juxtaposed along the western margin of the East Sea (i.e., the major thrust, crustal buckles, Hupo Fault, marine terraces, and Gorae Anticline). To understand their genetic relationship, more surgical investigations based on geodetic, and geophysical methods are required.
Data Availability Statement
The seismic reflection and well data used for this study are important properties of the KNOC and are not open access. However, the data may become accessible to people who have been permitted by KNOC.
Author Contributions
J-HL: formal analysis, investigation, and writing—original draft, J-HL: formal analysis, S-HY: validation, H-SL: validation and project administration, H-YS: data curation, G-BK: conceptualization, writing—original draft, writing—review and editing, and supervision.
Funding
This research was supported by the Korea Institute of Geoscience and Mineral Resources (No. GP2020-006), Korea Meteorological Administration Research Development Program (KMI 2018-02810), National Research Foundation of Korea (NRF-2018R1A1A1A0507746513), Basic Research Program of Pusan National University (202014360001 and 202015720001), and Ministry of the Interior and Safety as Human Resource Development Project in Earthquake Disaster Management.
Conflict of Interest
Author H-YS is employed by the KNOC.
The remaining authors declare that the research was conducted in the absence of any commercial or financial relationships that could be construed as a potential conflict of interest.
Publisher’s Note
All claims expressed in this article are solely those of the authors and do not necessarily represent those of their affiliated organizations, or those of the publisher, the editors and the reviewers. Any product that may be evaluated in this article, or claim that may be made by its manufacturer, is not guaranteed or endorsed by the publisher.
Acknowledgments
We appreciate editor, H-JK, and two reviewers, SL and LJ, for their kind comments. We also thank the KNOC for permission to use data set.
References
Arnott, R. W. C. (2010). “Deep-Marine Sediments and Sedimentary Systems,” in Facies Models 4. Editors N. P. James, and R. W. Dalrymple (Canada: Geological Association of Canada Press), 295–322.
Bahk, J.-J., Kang, N. K., Yi, B.-Y., Lee, S.-H., Jeong, S.-W., Urgeles, R., et al. (2017). Sedimentary Characteristics and Processes of Submarine Mass-Transport Deposits in the Ulleung Basin and Their Relations to Seismic and Sediment Physical Properties. Mar. Geology. 393, 124–140. doi:10.1016/j.margeo.2017.05.010
Cheon, Y., Choi, J.-H., Kim, N., Lee, H., Choi, I., Bae, H., et al. (2020). Late Quaternary Transpressional Earthquakes on a Long-Lived Intraplate Fault: A Case Study of the Southern Yangsan Fault, SE Korea. Quat. Int. 553, 132–143. doi:10.1016/j.quaint.2020.07.025
Choi, S.-J., Merritts, D. J., and Ota, Y. (2008). Elevations and Ages of Marine Terraces and Late Quaternary Rock Uplift in Southeastern Korea. J. Geophys. Res. 113 (B10), 1–15. doi:10.1029/2007JB005260
Choi, H., Hong, T.-K., He, X., and Baag, C.-E. (2012). Seismic Evidence for Reverse Activation of a Paleo-Rifting System in the East Sea (Sea of Japan). Tectonophysics 572-573 (17), 123–133. doi:10.1016/j.tecto.2011.12.023
Chough, S. K., and Barg, E. (1987). Tectonic History of Ulleung Basin Margin, East Sea (Sea of Japan). Geology 15 (1), 45–48. doi:10.1130/0091-7613(1987)15<45:thoubm>2.0.co;2
Chough, S. K., and Sohn, Y. K. (2010). Tectonic and Sedimentary Evolution of a Cretaceous Continental Arc–Backarc System in the Korean Peninsula: New View. Earth-Science Rev. 101 (3–4), 225–249. doi:10.1016/j.earscirev.2010.05.004
Chough, S. K., Lee, H. J., and Yoon, S. H. (2000). Marine Geology of Korean Seas. Amsterdam: Elsevier Press.
Cukur, D., Um, I.-K., Bahk, J.-J., Chun, J.-H., Horozal, S., Kim, S.-R., et al. (2018). Seismic Stratigraphy and Structural Characteristics of the Northeastern Continental Margin of Korea in the East Sea (Sea of Japan). Mar. Pet. Geology. 98, 706–717. doi:10.1016/j.marpetgeo.2018.09.013
Fabbri, O., Charvet, J., and Fournier, M. (1996). Alternate Senses of Displacement along the Tsushima Fault System during the Neogene Based on Fracture Analyses Near the Western Margin of the Japan Sea. Tectonophysics 257 (2–4), 275–295. doi:10.1016/0040-1951(95)00151-4
Faccenna, C., Holt, A. F., Becker, T. W., Lallemand, S., and Royden, L. H. (2018). Dynamics of the Ryukyu/Izu-Bonin-Marianas Double Subduction System. Tectonophysics 746, 229–238. doi:10.1016/j.tecto.2017.08.011
Hall, R. (2002). Cenozoic Geological and Plate Tectonic Evolution of SE Asia and the SW Pacific: Computer-Based Reconstructions, Model and Animations. J. Asian Earth Sci. 20 (4), 353–431. doi:10.1016/S1367-9120(01)00069-4
Horozal, S., Chae, S., Seo, J. M., Lee, S. M., Han, H. S., Cukur, D., et al. (2021). Quaternary Evolution of the Southeastern Korean Continental Shelf, East Sea: Paleo-Incised Valley and Channel Systems. Mar. Pet. Geology. 128, 105011. doi:10.1016/j.marpetgeo.2021.105011
Ingle, J. C. J. (1992). Subsidence of the Japan Sea: Stratigraphic Evidence from ODP Sites and Onshore Sections. Proc. ODP. Sci. Results 127/128 (2), 1197–1218. doi:10.2973/odp.proc.sr.127128-2.132.1992
Jolivet, L., and Tamaki, K. (1992). Neogene Kinematics in the Japan Sea Region and Volcanic Activity of the Northeast Japan Arc. Proc. ODP. Sci. Results 127/128 (2), 1311–1331. doi:10.2973/odp.proc.sr.127128-2.239.1992
Jolivet, L., Huchon, P., Brun, J. P., Le Pichon, X., Chamot-Rooke, N., and Thomas, J. C. (1991). Arc Deformation and Marginal basin Opening: Japan Sea as a Case Study. J. Geophys. Res. 96 (B3), 4367–4384. doi:10.1029/90JB02455
Jolivet, L., Shibuya, H., and Fournier, M. (1995). “Paleomagnetic Rotations and the Japan Sea Opening,” in Active Margins and Marginal Basins of the Western Pacific, Geophys. Monogr. Ser. 88. Editors B. Taylor, and J. Natland (Washington D. C.: AGU Press), 355–369. doi:10.1029/GM088p0355
Kaneoka, I., Notsu, K., Takigami, Y., Fujioka, K., and Sakai, H. (1990). Constraints on the Evolution of the Japan Sea Based on 40Ar-39Ar Ages and Sr Isotopic Ratios for Volcanic Rocks of the Yamato Seamount Chain in the Japan Sea. Earth Planet. Sci. Lett. 97 (1–2), 211–225. doi:10.1016/0012-821X(90)90109-B
Kaneoka, I., Takigami, Y., Takaoka, N., Yamashita, S., and Tamaki, K. (1992). 40Ar-39Ar Analysis of Volcanic Rocks Recovered from the Japan Sea Floor: Constraints on the Age of Formation of the Japan Sea. Proc. ODP. Sci. Results 127/128 (2), 819–836. doi:10.2973/odp.proc.sr.127128-2.200.1992
KIGAM (Korea Institute of Geoscience and Mineral Resources) (2006). Research on Stratigraphy and Tectonics of Southeastern Korean Offshore Using Seismic Exploration Technology OAA2004005-2006 (3), 332.
Kim, G. B., and Yoon, S.-H. (2017). An Insight into Asymmetric Back-Arc Extension: Tecto-Magmatic Evidences from the Ulleung Basin, the East Sea (Sea of Japan). Tectonophysics 717, 182–192. doi:10.1016/j.tecto.2017.07.016
Kim, H. G., Song, C. W., Kim, J. S., Son, M., and Kim, I. S. (2008). Tertiary Geological Structures and Deformation History of the Southern Tsushima Island, Japan. J. Geo. Soc. Korea 44 (2), 175–198.
Kim, G. B., Yoon, S. H., Chough, S. K., Kwon, Y. K., and Ryu, B. J. (2011). Seismic Reflection Study of Acoustic Basement in the South Korea Plateau, the Ulleung Interplain Gap, and the Northern Ulleung Basin: Volcano-Tectonic Implications for Tertiary Back-Arc Evolution in the Southern East Sea. Tectonophysics 504 (1–4), 43–56. doi:10.1016/j.tecto.2011.02.004
Kim, G. B., Yoon, S. H., Sohn, Y. K., and Kwon, Y. K. (2013). Wave-Planation Surfaces in the Mid-Western East Sea (Sea of Japan): Indicators of Subsidence History and Paleogeographic Evolution of Back-Arc Basin. Mar. Geology. 344, 65–81. doi:10.1016/j.margeo.2013.07.008
Kim, H.-J., Moon, S., Jou, H.-T., Lee, G. H., Yoo, D. G., Lee, S. H., et al. (2016). The Offshore Yangsan Fault Activity in the Quaternary, SE Korea: Analysis of High-Resolution Seismic Profiles. Tectonophysics 693, 85–95. doi:10.1016/j.tecto.2016.10.034
Kim, Y., Rhie, J., Kang, T.-S., Kim, K.-H., Kim, M., and Lee, S.-J. (2016). The 12 September 2016 Gyeongju Earthquakes: 1. Observation and Remaining Questions. Geosci. J. 20 (6), 747–752. doi:10.1007/s12303-016-0033-x
Kim, G.-B., Yoon, S.-H., Kim, S.-S., and So, B.-D. (2018). Transition from Buckling to Subduction on Strike-Slip Continental Margins: Evidence from the East Sea (Japan Sea). Geology 46 (7), 603–606. doi:10.1130/G40305.1
Kim, K.-H., Ree, J.-H., Kim, Y., Kim, S., Kang, S. Y., and Seo, W. (2018). Assessing whether the 2017 Mw 5.4 Pohang Earthquake in South Korea Was an Induced Event. Science 360 (6392), 1007–1009. doi:10.1126/science.aat6081
Kim, S. W. (1973). A Study on the Terraces along the Southeastern Coast (Bang-Eojin–Pohang) of the Korean Peninsula. J. Geo. Soc. Korea 9 (2), 89–121. doi:10.3348/jkrs.1973.9.1.16
Kim, I. S. (1992). Origin and Tectonic Evolution of the East Sea (Sea of Japan) and the Yangsan Fault System: A New Synthetic Interpretation. J. Geo. Soc. Korea 28 (1), 84–109.
Kimura, G., Hashimoto, Y., Kitamura, Y., Yamaguchi, A., and Koge, H. (2014). Middle Miocene Swift Migration of the TTT Triple Junction and Rapid Crustal Growth in Southwest Japan: A Review. Tectonics 33 (7), 1219–1238. doi:10.1002/2014TC003531
Lallemand, S., and Jolivet, L. (1986). Japan Sea: A Pull-Apart Basin? Earth Planet. Sci. Lett. 76 (3–4), 375–389. doi:10.1016/0012-821X(86)90088-9
Lee, Y. S., Ishikawa, N., and Kim, W. K. (1999). Paleomagnetism of Tertiary Rocks on the Korean Peninsula: Tectonic Implications for the Opening of the East Sea (Sea of Japan). Tectonophysics 304 (1–2), 131–149. doi:10.1016/S0040-1951(98)00270-4
Lee, G. H., Yoon, Y., Nam, B. H., Lim, H., Kim, Y.-S., Kim, H. J., et al. (2011). Structural Evolution of the Southwestern Margin of the Ulleung Basin, East Sea (Japan Sea) and Tectonic Implications. Tectonophysics 502 (3), 293–307. doi:10.1016/j.tecto.2011.01.015
Miall, A. D. (1995). “Collision-Related Foreland Basins,” in Tectonics of Sedimentary Basins. Editors C. J. Busby, and R. V. Ingersoll (Massachusetts: Blackwell Science Press), 393–424.
Mitchum, R. M., Vail, P. R., and Sangree, J. B. (1977). “Seismic Stratigraphy and Global Changes of Sea Level, Part 6: Stratigraphic Interpretation of Seismic Reflection Patterns in Depositional Sequences1,” in Seismic Stratigraphy – Applications to Hydrocarbon Exploration. Editor C. E. Payton (Tulsa: AAPG Press), 117–133. doi:10.1306/M26490
Nakamura, K. (1983). Possible Nascent Trench along the Eastern Japan Sea as the Convergent Boundary between Eurasian and North American Plates. Bull. Earthq. Res. Inst. Univ. Tokyo 58, 711–722.
No, T., Sato, T., Kodaira, S., Ishiyama, T., Sato, H., Takahashi, N., et al. (2014). The Source Fault of the 1983 Nihonkai-Chubu Earthquake Revealed by Seismic Imaging. Earth Planet. Sci. Lett. Sci. Lett. 400 (15), 14–25. doi:10.1016/j.epsl.2014.05.026
Okada, S., and Ikeda, Y. (2012). Quantifying Crustal Extension and Shortening in the Back-Arc Region of Northeast Japan. J. Geophys. Res. 117 (B1), 1404. doi:10.1029/2011JB008355
Park, Y., Yoo, D., Kang, N., and Yi, B. (2019). Origin and Evolution of Stacked Cut-And-Fill Structures on the Southwestern Margin of the Ulleung Basin, East Sea (Japan Sea). J. Sediment. Res. 89 (7), 679–700. doi:10.2110/jsr.2019.36
Park, Y., Yoo, D., Kang, N., Yi, B., and Kim, B. (2021). Tectonic Control on Mass‐Transport Deposit and Canyon‐Fed Fan System in the Ulleung Basin, East Sea (Sea of Japan). Basin Res. 33 (2), 991–1016. doi:10.1111/bre.12501
Porębski, S. J., and Steel, R. J. (2003). Shelf-Margin Deltas: Their Stratigraphic Significance and Relation to Deepwater Sands. Earth-Sci. Rev. 62 (3–4), 283–326. doi:10.1016/S0012-8252(02)00161-7
Sato, T., Kosuga, M., and Tanaka, K. (1986). Aftershock Distribution of the 1983 Nihonkai-Chubu (Japan Sea) Earthquake Determined from Relocated Hypocenters. J. Phys. Earth. 34 (3), 203–223. doi:10.4294/jpe1952.34.203
Shaw, J. H., Connors, C., and Suppe, J. (2005). Seismic Interpretation of Contractional Fault-Related Folds: An AAPG Seismic Atlas. Tulsa, Oklahoma: American Association of Petroleum Geologists, 53. doi:10.1306/St531003
Sohn, Y. K., Rhee, C. W., and Shon, H. (2001). Revised Stratigraphy and Reinterpretation of the Miocene Pohang Basinfill, SE Korea: Sequence Development in Response to Tectonism and Eustasy in a Back-Arc Basin Margin. Sediment. Geol. 143 (3–4), 265–285. doi:10.1016/S0037-0738(01)00100-2
Son, M., Song, C. W., Kim, M.-C., Cheon, Y., Cho, H., and Sohn, Y. K. (2015). Miocene Tectonic Evolution of the Basins and Fault Systems, SE Korea: Dextral, Simple Shear during the East Sea (Sea of Japan) Opening. J. Geol. Soc. 172 (5), 664–680. doi:10.1144/jgs2014-079
Taira, A. (2001). Tectonic Evolution of the Japanese Island Arc System. Annu. Rev. Earth Planet. Sci. 29 (1), 109–134. doi:10.1146/annurev.earth.29.1.109
Tamaki, K., and Honza, E. (1985). Incipient Subduction and Deduction along the Eastern Margin of the Japan Sea. Tectonophysics 119 (1–4), 381–406. doi:10.1016/0040-1951(85)90047-2
Tamaki, K., Suyehiro, K., Allan, J., Ingle, J. C. J., and Pisciotto, K. A. (1992). Tectonic Synthesis and Implications of Japan Sea ODP Drilling. Proc. ODP. Sci. Results 127/128 (2), 1333–1348. doi:10.2973/odp.proc.sr.127128-2.240.1992
Tamaki, K. (1986). Age Estimation of the Japan Sea on the Basis of Stratigraphy, Basement Depth, and Heat Flow Data. J. Geomagn. Geoelec 38 (5), 427–446. doi:10.5636/jgg.38.427
Tamaki, K. (1988). Geological Structure of the Japan Sea and its Tectonic Implications. Bull. Geol. Surv. Jpn. 39 (5), 269–365.
Weimer, P. (1990). Sequence Stratigraphy, Facies Geometries, and Depositional History of the Mississippi Fan, Gulf of Mexico (1). Bulletin 74 (4), 425–453. doi:10.1306/0C9B2321-1710-11D7-8645000102C1865D
Yamamoto, H. (1993). Submarine Geology and Post-Opening Tectonic Movements in the Southern Region of the Sea of Japan. Mar. Geol. 112 (1–4), 133–150. doi:10.1016/0025-3227(93)90165-R
Yi, S., Yun, H., Park, B., Koo, W. M., Yoo, S., and Kang, S. (2020). Biostratigraphy of the Ulleung Basin, East Sea (Japan Sea). Mar. Pet. Geology. 122, 104697. doi:10.1016/j.marpetgeo.2020.104697
Yoon, S. H., and Chough, S. K. (1995). Regional Strike Slip in the Eastern Continental Margin of Korea and its Tectonic Implications for the Evolution of Ulleung Basin, East Sea (Sea of Japan). Geol. Soc. America Bull. 107 (1), 83–97. doi:10.1130/0016-7606(1995)107<0083:rssite>2.3.co;2
Yoon, S. H., Park, S. J., and Chough, S. K. (1997). Western Boundary Fault Systems of Ulleung Back-Arc Basin: Further Evidence of Pull-Apart Opening. Geosci. J. 1 (2), 75–88. doi:10.1007/BF02910479
Yoon, S. H., Park, S. J., and Chough, S. K. (2002). Evolution of Sedimentary Basin in the Southwestern Ulleung Basin Margin: Sequence Stratigraphy and Geologic Structures. Geosci. J. 6 (2), 149–159. doi:10.1007/BF03028286
Yoon, S. H., Chough, S. K., and Park, S. J. (2003). Sequence Model and its Application to a Miocene Shelf–Slope System in the Tectonically Active Ulleung Basin Margin, East Sea (Sea of Japan). Mar. Pet. Geology. 20 (10), 1089–1103. doi:10.1016/j.marpetgeo.2003.08.001
Keywords: neotectonics, post-Miocene tectonic deformations, tectostratigraphy, seismic stratigraphy, Ulleung Basin, Dolgorae Thrust-Fold Belt, Gorae Anticline
Citation: Lee J-H, Lee J-H, Yoon S-H, Lee H-S, Song H-Y and Kim G-B (2022) Two Distinct Back-Arc Closure Phases of the East Sea: Stratigraphic Evidence From the SW Ulleung Basin Margin. Front. Earth Sci. 10:839712. doi: 10.3389/feart.2022.839712
Received: 20 December 2021; Accepted: 24 January 2022;
Published: 25 February 2022.
Edited by:
Han-Joon Kim, Korea Institute of Ocean Science and Technology, South KoreaReviewed by:
Serge Lallemand, UMR5243 Géosciences Montpellier, FranceLaurent Jolivet, Sorbonne Universités, France
Copyright © 2022 Lee, Lee, Yoon, Lee, Song and Kim. This is an open-access article distributed under the terms of the Creative Commons Attribution License (CC BY). The use, distribution or reproduction in other forums is permitted, provided the original author(s) and the copyright owner(s) are credited and that the original publication in this journal is cited, in accordance with accepted academic practice. No use, distribution or reproduction is permitted which does not comply with these terms.
*Correspondence: Gi-Bom Kim, kimgb@pusan.ac.kr