Genetic testing and microstructure characterization of biological crusts on the rammed soil surface at the Shanhaiguan great wall in China
- 1School of Earth Sciences and Engineering, Nanjing University, Nanjing, China
- 2Hebei Bureau of Geology and Mineral Resources Exploration, Qinhuangdao, China
- 3School of Civil Engineering, Geotechnical Institute, Tianjin University, Tianjin, China
- 4National Aquatic Biological Resource Center, Institute of Hydrobiology, Chinese Academy of Sciences, Wuhan, China
- 5School of Civil Engineering and Mechanics, Yanshan University, Qinhuangdao, China
Protection of cultural relics and sites is of great significance. In this study, the new gray-green thin-layer biological crust on the rammed soil surface at the Shanhaiguan Great Wall in China was found. The emergence of this material has substantially improved the resistance of the rammed Earth Great Wall to rain erosion. 16S rRNA gene sequencing on the surface crusts of rammed Earth was performed. Results show the biological crusts were mainly algae-based composite crusts containing fungi. At the genus level, microalgae and Sphingomycetes were predominant. Under scanning electron microscopy (SEM), algae filaments dominated by filamentous algae overlapped and intertwined with each other. Furthermore, polysaccharide organic matter secreted by algae formed a covering film. The two formed a complex spatial network structure to envelop soil particles, which enhances erosion resistance. The conformable biological crusting is expected to be used as a new civil engineering material for the protection of rammed Earth sites in the future.
Introduction
The Great Wall is an important military defense system in ancient China and a world historical and cultural heritage site (Chen et al., 2015; Chen et al., 2018a). The Shanhaiguan Great Wall was built during the Ming Dynasty, and the wall is mainly composed of outer bricks and rammed Earth inside the wall. Due to climatic and historical reasons, some of the city bricks disappeared, leaving only the rammed Earth wall core. The rammed Earth wall core has experienced collapse and erosion damage due to rain, causing irreversible damage to cultural relics. Therefore, civil engineering materials used to protect the rammed Earth of the Great Wall urgently need to be developed.
The Great Wall is a world cultural site, and the protection of the rammed Earth of the Great Wall needs to be implemented under the consideration of the principle of in situ protection in accordance with the requirements for the protection of cultural relics and sites (Li et al., 2011b). That is, no change in the status quo and minimal intervention. This creates a challenge in the selection of protective materials. Therefore, some scholars have used inorganic materials and organic polymer materials to carry out protective experiments on rammed Earth surfaces (Maravelaki-Kalaitzaki et al., 2008; Kim et al., 2009; Fang et al., 2015b; Chen et al., 2016; Chen et al., 2018b). The advantages of organic reinforcement are good permeability, strong adhesion and flexibility, and good hydrophobicity; however, the disadvantage is that most are toxic and susceptible to environmental degradation due to environmental influences (Zhao et al., 2008; Li et al., 2011a; Wang et al., 2011). Inorganic materials have good antiaging performance, long service life, and relatively low costs; however, the disadvantages are high shrinkage, poor water resistance, and low reinforcement ability. Therefore, a new type of civil engineering material suitable for the rammed Earth of the Great Wall remains to be found (Chen et al., 2015; He et al., 2019).
Notably, a gray-green thin-layer biological crusting material was found through on-site investigation. The presence of this material has substantially improved the resistance of rammed Earth sites to rain erosion. So, it is essential to study the biological composition and microstructure of the material to further understand the erosion resistance mechanism. In this study, five groups of biocrust materials from different locations are collected. The 16S rRNA gene was sequenced to determine its biological composition and dominant attributes. And SEM was adopted to observe the microstructure.
Materials and methods
Biological crusts on the rammed soil surface of the shanhaiguan great wall
Figure 1 shows photos of rammed Earth Great Wall biological crust. As shown in Figure 1A, there is clearly no rainwater erosion of the biological crusts found on the surface of the Great Wall rammed Earth (Fang et al., 2015a). However, traces of rainwater erosion are clearly visible in the crusts of non-growing organisms. The biological crusts of rammed Earth surfaces are gray-green, with a thickness of approximately 1–3 mm. Under the crustal layer is primitive rammed Earth, the soil structure is dense, silty clay, and contains crushed stone particles, as shown in Figure 1B. The experimental samples were collected from the Shanhaiguan Great Wall in China, and a total of five sets of samples were selected and named JP1∼JP5. JP1, JP2, JP3, and JP4 are the biological crust samples taken from the Great Wall itself, and JP5 are the biological crust samples taken from the natural ground at the foot of the Great Wall slope.
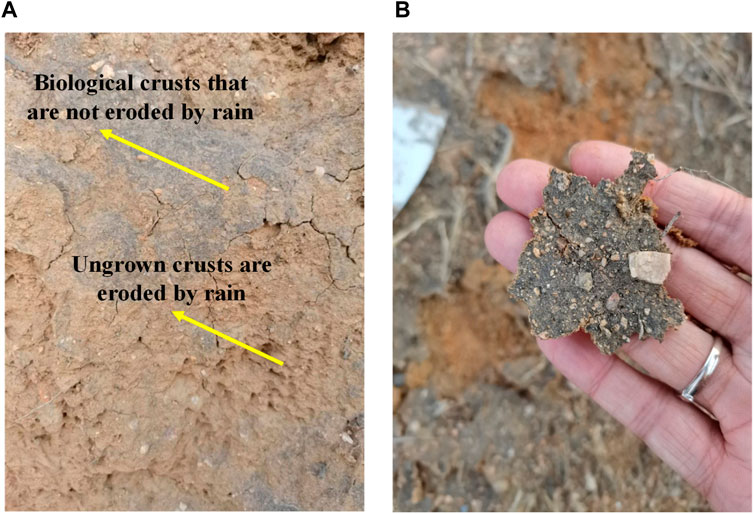
FIGURE 1. Rammed Earth Great Wall biological crust: (A) The biological crusts are resistant to rain erosion, (B) Biological Crusts sample.
Genomic DNA sequencing
The 16S rRNA gene was sequenced from five groups of biological crust samples, the test was conducted in a gene laboratory, Wuhan. It was used to identify the genetic characteristics and dominant species of the biological crusts. The test process was as follows: DNA extraction → polymerase chain reaction (PCR) amplification → amplicon library construction → machine sequencing.
SEM testing
Gray-green biological crust samples were selected for drying for SEM observation. The VEGA3 (LM) fully automated tungsten filament scanning electron microscope produced by TESCAN in the Czech Republic was used for this test. The experimental magnification was 500–20,000 times.
Results and DISCUSSION
Biodiversity analysis
Figure 2A shows OUTs and rank abundance of five samples, where OTUs refer to the number of per unit volume. From Figure 2A, it could be found that JP5 samples collected from the foot of the Great Wall had the largest number of organisms, with 1699 OTUs (OTU is the abbreviation for Operational Taxonomic Units). The OTU numbers of JP2, JP3, and JP4 were basically the same, with 770, 949, and 940, respectively. The number of OTUs in JP1 was between them, with 1338. This shows that the number of ground organisms exceeds the number of wall organisms, and the ground biological crusts are more complex.
The rank abundance curve is shown in Figure 3. The abscissa indicates the abundance ranking of the OTU, and the ordinate indicates its relative abundance. This reflects the diversity of species composition and the distribution of species abundance. From Figure 2B, JP5 has the largest sample abundance, followed by the JP1 sample, JP3 is close to JP4, and JP2 is the smallest. The biodiversity of the five sampling points had certain differences, and the foot of the Great Wall was the most different from the surface of the Great Wall.
Community composition of biological crusts
The dilution curves for 16S rRNA sequencing of five samples are shown in Figure 3. As shown in Figure 3, the dilution curves of each sample first increase rapidly and then flatten. This shows that the amount of sequencing data is reasonable, the sequencing depth is appropriate, and the data reflect the real situation of the microbial community.
Figure 4 presents the classification of organisms of five samples. It could be found that a total of 28 phyla, 65 classes, 140 orders, 177 families, 356 genera, and 403 species were included in five samples.
Figure 5A shows that the top five of the five groups of test samples at the phylum level include Cyanobacteria, Proteobacteria, Actinobacteriota, Chloroflexi, Bacteroidota, and Planctomycetota. The dominant phylum was Cyanobacteria for all groups.
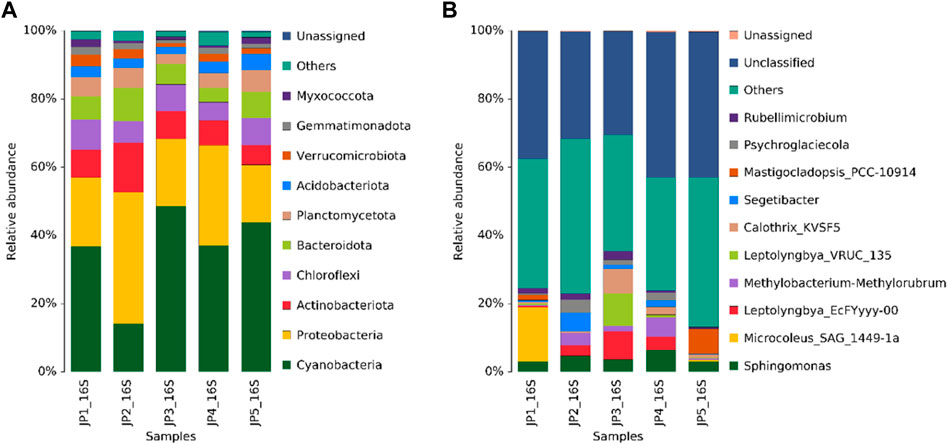
FIGURE 5. Bacterial community composition in crusts at different sampling points: (A) Colony column chart at the phylum, (B) Colony column chart at the genera.
Figure 5B presents colony column chart at the genera. Obviously, there are great differences in the distribution of horizontal communities among the samples. To compare the five samples more clearly, the top two genera for every sample are shown in Table 1. It could be found that the top two genera of JP1 include Microcoleus (15.77%) and Sphingomonas (3.14%); the top two genera of JP2 are Segetibacter (5.48%) and Sphingomonas (4.72%); the top two genera of JP3 are Leptolyngbya (17.83%) and Calothrix (7.20%); the top two genera of JP4 are Sphingomonas (6.55%) and Methylobacterium (5.70%); and the top two genera of JP5 are Mastigocladopsis (7.37%) and Sphingomonas (3.13%). Among them, Microcoleus, Leptolyngbya, Calothrix, and Mastigocladopsis all belong to Cyanobacteria. So, the dominant species are Microcoleus and Leptolyngbya of Cyanobacteria.
SEM microstructure
Figure 6 shows SEM results for a 500–5000 magnification of the biocortex. Under a scanning electron microscope, Figure 6A is a ×500 magnification photograph of the crust. The figure shows that the filamentous algae grow densely and overlap with each other, forming a spatial network pattern. Figure 6B shows the crust magnified at 1000x. Filamentous algae can be seen intertwined and covering the surface of soil particles, tightly locking the soil particles. Figure 6C shows a ×2000 magnified view of the crust. From Figure 6C, the polysaccharide mucus secreted by algae covers the surface of the soil particles, forming a dragnet membrane-like structure that covers the soil below. Figure 6D shows a magnified 5000x photo of the crust, and the state of the filamentous algae can be clearly seen.
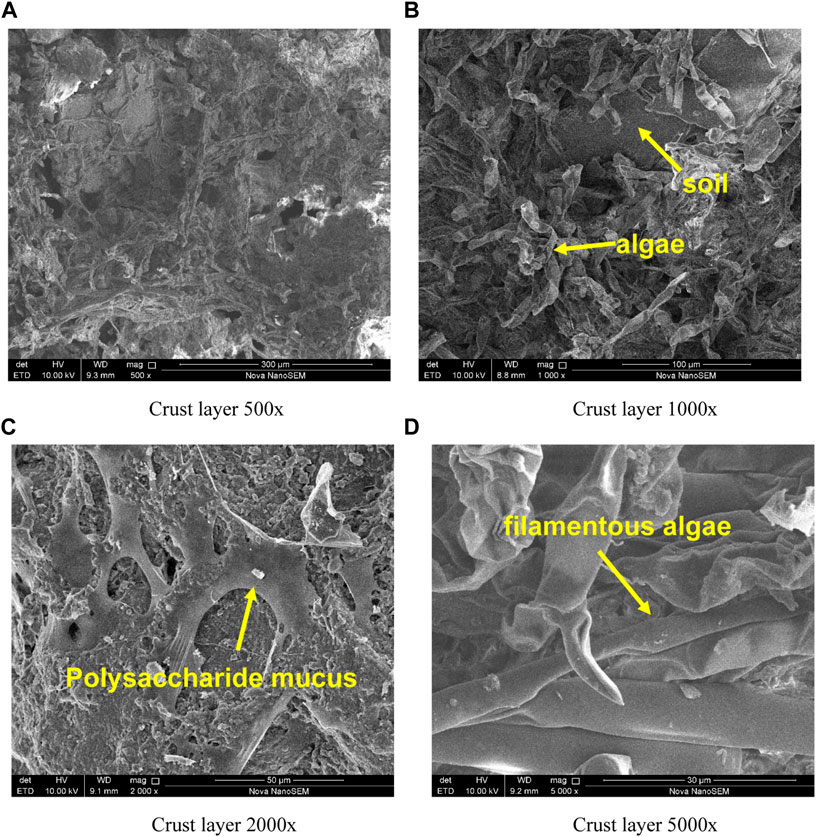
FIGURE 6. SEM photo of the crust layer under different magnifications: (A) 500, (B) 1000, (C) 2000, and (D) 5000.
From the survey site, it was found that algal biological crusts can effectively prevent rainwater erosion and washing. The anti-erosion mechanism of algal crusts is explained as follows: algae secrete polysaccharides, lipids and proteins under photosynthesis, which have a bonding effect and can effectively bind soil particles (Mazor et al., 1996; Hokputsa, 2003). On the other hand, algae silk, mycelium and soil particles form a wrap and entanglement, which can firmly lock the soil particles (Belnap and Gillette, 1998; Hu et al., 2002). Furthermore, it forms a special multilayer network structure with polysaccharide mucus secretions so that the stability of the soil surface is enhanced (Ye et al., 2020).
Conclusion
From the on-site investigation, it is known that the biological crusts of the rammed Earth of the Shanhaiguan Great Wall have strong rain erosion resistance. These biological crusts are a new material for civil engineering with great potential in the future. Through genome sequencing and SEM testing of biological crust samples, we have reached the following conclusions:
1) Biological crusts conform to biological crusts that are dominated by the phylum Cyanobacteria and contain a variety of fungi. At the genus level, filamentous algae, dominated by microalgae and Sphingomycetes, predominate.
2) Algae algae silk, mycelium and soil particles form a wrap and entanglement, which can firmly lock the soil particles. In addition, it forms a special multilayer network structure with polysaccharide mucus secretions so that the stability of the soil surface is enhanced.
3) Future work can analyze and extract the dominant algal species through the analysis of the crustal biodiversity of native algae of the Great Wall. The dominant algae can be inoculated on the surface of the rammed Earth of the Great Wall for rain erosion test analysis. Native algae can be turned into usable civil engineering protection materials.
Data availability statement
The original contributions presented in the study are included in the article/supplementary material, further inquiries can be directed to the corresponding authors.
Author contributions
LL: Data curation, Formal Analysis, Funding acquisition, Investigation, Methodology, Visualization, Writing–original draft, Writing–review and editing. YZ: Investigation, Resources, Supervision, Writing–review and editing. JL: Data curation, Formal Analysis, Software, Writing–review and editing, Investigation, Methodology. LG: Conceptualization, Methodology, Writing–review and editing. LZ: Methodology, Resources, Writing–review and editing. TL: Methodology, Software, Writing–original draft. HC: Conceptualization, Methodology, Resources, Supervision, Writing–review and editing.
Funding
The author(s) declare financial support was received for the research, authorship, and/or publication of this article. This work was supported by Hebei Bureau of Geology and Mineral Resources Exploration, China (No. 13000023P0069B410176J) and the Housing and Urban Rural Development Department of Hebei Province, China (No. 2023-2094).
Conflict of interest
Authors LL and LG were employed by Hebei Bureau of Geology and Mineral Resources Exploration.
The remaining authors declare that the research was conducted in the absence of any commercial or financial relationships that could be construed as a potential conflict of interest.
Publisher’s note
All claims expressed in this article are solely those of the authors and do not necessarily represent those of their affiliated organizations, or those of the publisher, the editors and the reviewers. Any product that may be evaluated in this article, or claim that may be made by its manufacturer, is not guaranteed or endorsed by the publisher.
References
Belnap, J., and Gillette, D. A. (1998). Vulnerability of desert biological soil crusts to wind erosion: the influences of crust development, soil texture, and disturbance. J. Arid Environ. 39, 133–142. doi:10.1006/jare.1998.0388
Chen, W., Dai, P., Yuan, P., and Zhang, J. (2016). Effect of inorganic silicate consolidation on the mechanical and durability performance of sandstone used in historical sites. Constr. Build. Mater. 121, 445–452. doi:10.1016/j.conbuildmat.2016.06.008
Chen, W., Du, Y., Cui, K., Fu, X., and Gong, S. (2018a). Architectural forms and distribution characteristics of beacon towers of the ming great wall in qinghai Province. J. Asian Archit. Build. Eng. 16, 503–510. doi:10.3130/jaabe.16.503
Chen, W., Zhang, Y., Zhang, J., and Dai, P. (2018b). Consolidation effect of composite materials on earthen sites. Constr. Build. Mater. 187, 730–737. doi:10.1016/j.conbuildmat.2018.07.239
Chen, W. W., Guo, Z. Q., Xu, Y. R., Chen, P. F., Zhang, S., and Ye, F. (2015). Laboratory tests on rammed earth samples of earthen sites instilled by reinforcement material SH. Chin. J. Geotechnical Eng. 37, 1517–1523. Chinese.
Fang, S., Yu, W., and Qi, Y. (2015a). Spectra and vegetation index variations in moss soil crust in different seasons, and in wet and dry conditions. Int. J. Appl. Earth Observation Geoinformation 38, 261–266. doi:10.1016/j.jag.2015.01.018
Fang, X. N., Chen, W. T., Feng, G. L., Liu, L. M., and Yi, X. G. (2015b). Syntheses of series of organosilicon-acrylate composite emulsions for the consolidation and conservation of historical earthen sites. Asian J. Chem. 27, 3523–3524. doi:10.14233/ajchem.2015.18643
He, F. G., Lv, R., Su, H. Z., Zhou, J., Zhang, J. K., and Wang, N. (2019). Durability test and reinforced mechanism on adding SH materials into soil of archaeological sites. Rock Soil Mech. 40 (S1), 297–307. Chinese.
Hokputsa, S. (2003). A physico-chemical comparative study on extracellular carbohydrate polymers from five desert algae. Carbohydr. Polym. 54, 27–32. doi:10.1016/s0144-8617(03)00136-x
Hu, C. X., Liu, Y. D., Song, L. R., and Zhang, D. L. (2002). Effect of desert soil algae on the stabilization of fine sands. J. Appl. Phycol. 14, 281–292. doi:10.1023/a:1021128530086
Kim, E. K., Won, J., Do, J. Y., Kim, S. D., and Kang, Y. S. (2009). Effects of silica nanoparticle and GPTMS addition on TEOS-based stone consolidants. J. Cult. Herit. 10 (2), 214–221. doi:10.1016/j.culher.2008.07.008
Li, Z. X., Shao, M. S., and Chen, R. (2011a). Impact of PS on permeability of unsaturated ruins clay. Rock Soil Mech. 32, 2039–2044. Chinese.
Li, Z. X., Wang, X. D., Sun, M. L., Chen, W., Guo, Q., and Zhang, H. (2011b). Conservation of Jiaohe ancient earthen site in China. J. Rock Mech. Geotechnical Eng. 3 (3), 270–281. doi:10.3724/sp.j.1235.2011.00270
Maravelaki-Kalaitzaki, P., Kallithrakas-Kontos, N., Agioutantis, Z., Maurigiannakis, S., and Korakaki, S. (2008). A comparative study of porous limestones treated with silicon-based strengthening agents. Prog. Org. Coatings 62 (1), 49–60. doi:10.1016/j.porgcoat.2007.09.020
Mazor, G., Kidron, G. J., Vonshak, A., and Abeliovich, A. (1996). The role of cyanobacterial exopolysaccharides in structuring desert microbial crusts. FEMS Microbiol. Ecol. 21, 121–130. doi:10.1111/j.1574-6941.1996.tb00339.x
Wang, T. R., Zhang, D. X., Wang, X. D., and Guo, Q. L. (2011). Experimental study of infrared imaging technology detecting earthen ruins soil reinforced by potassium silicate(PS). Rock Soil Mech. 32, 630–635. Chinese.
Ye, C. R., Tao, Y., Zhang, Y. R., Cao, J., Ke, T., Wei, S. J., et al. (2020). Monosaccharide composition of primary cell wall polysaccharides as a developmental level indicator of biological soil crusts. Catena 195, 104782. doi:10.1016/j.catena.2020.104782
Keywords: electron microscopy, slopes-erosion control, eosynthetic-reinforced soils walls and slopes, bio geotechnics, biopolymer
Citation: Liu L, Zhang Y, Liu J, Guo L, Zheng L, Li T and Cao H (2023) Genetic testing and microstructure characterization of biological crusts on the rammed soil surface at the Shanhaiguan great wall in China. Front. Earth Sci. 11:1314212. doi: 10.3389/feart.2023.1314212
Received: 10 October 2023; Accepted: 06 November 2023;
Published: 28 December 2023.
Edited by:
Zhenwei Dai, China Geological Survey (Geosciences Innovation Center of Central South China), ChinaReviewed by:
Yang Liu, University of Science and Technology Beijing, ChinaYijie Sun, Nanjing Tech University, China
Copyright © 2023 Liu, Zhang, Liu, Guo, Zheng, Li and Cao. This is an open-access article distributed under the terms of the Creative Commons Attribution License (CC BY). The use, distribution or reproduction in other forums is permitted, provided the original author(s) and the copyright owner(s) are credited and that the original publication in this journal is cited, in accordance with accepted academic practice. No use, distribution or reproduction is permitted which does not comply with these terms.
*Correspondence: Yun Zhang, zhangyun@nju.edu.cn; Lianjun Guo, qhdgebm@163.com; Haiying Cao, chyysu79@126.com