- 1Center for Plant Sciences and Biodiversity, University of Swat, Swat, Pakistan
- 2State Key Laboratory of Molecular Developmental Biology, Institute of Genetics and Developmental Biology, Chinese Academy of Sciences, Beijing, China
- 3Instituto de Conservación y Mejora de la Agrodiversidad Valenciana, Universitat Politécnica de Valéncia, Valencia, Spain
- 4Botany and Microbiology Department, King Saud University, Riyadh, Saudi Arabia
- 5Department of Plant Sciences, Quaid-i-Azam University, Islamabad, Pakistan
Crop wild resources are excellent sources of new genetic variation for resilience against climate extremes. However, detailed characterization of the desirable phenotypes is essential before using these crop wild resources in breeding programs. This current study was, therefore, conducted to investigate the water stress responses of eight wild Elymus species and two wheat cultivars. The experiment was carried out under varying levels of osmotic stress induced by polyethylene glycol and progressive water stress through different field capacities. Water stress significantly reduced both physiological and biochemical traits compared to control, ranging from 7.1% (protein content) to 34.5% (chlorophyll) under moderate stress and 9.1–45.8% under severe stress. The anatomical features were also affected under progressive water stress, including a reduction in xylem vessel diameter (7.92 and 16.50%), phloem length (4.36 and 7.18%), vascular bundle length (3.09 and 6.04%), and ground tissue thickness (2.36 and 5.52%), respectively. Conclusively, Elymus borianus (endemic to Swat, Pakistan), E. russelli, E. caninus, E. longioristatus, and E. dauhuricus outperformed the check wheat cultivar, Pirsabak 2005, which is a rainfed variety. The results revealed that Elymus species belonging to the tertiary gene pool of bread wheat could be an excellent drought tolerance source for use in a breeding program.
Introduction
Wheat (Triticum aestivum L.) is the most widely grown cereal crop and essential staple food, with a global production of more than 768 million metric tons per annum (Erenstein et al., 2022). The wheat requirement increases with each passing day due to stagnant yield per unit area and a rise in the global population (Ray et al., 2015). It has been estimated that worldwide wheat production needs to be increased by 60% to feed a population of 9.1 billion by the year 2050 (Grote et al., 2021). Drought stress affects morphological and anatomical features of roots, stems, and leaves, which ultimately reduces plant physiological functions (Ali et al., 2013; Salam et al., 2022). Drought stress at pre-anthesis reduces grain yield by affecting photosynthetic activity, and decreasing the accumulation of reserves in the stem (Ehdaie et al., 2006; Ali et al., 2014a; Fatima et al., 2014). At reproductive stages, the wheat crop is highly vulnerable to drought stress, which ultimately reduces the fertility of spikelet, the weight of a single grain, and the duration of grain filling (Prasad et al., 2011; Mahrookashani et al., 2017). Further, drought stress also affects flowering times in plants (Ali et al., 2022a,b). It increases the production of harmful substances like reactive oxygen species (ROS), which damages the metabolic activities, structures, and functions of plant cells (Suneja et al., 2017). Plants produce different types of antioxidants to detoxify ROS and protect themselves from its negative effects by inducing drought tolerance (Ashraf, 2010; Verma et al., 2014). Under drought stress, increased accumulation of antioxidants results in ROS scavenging, which may lead to increased proline and decreased protein content in plants (Hessini et al., 2009; Zhang and Nan, 2010).
Establishing the agronomic superiority of wheat germplasm derived from crop-wild introgression under abiotic stress conditions is of prime importance in breeding (Afzal et al., 2018). Utilization of wild crop resources is a promising approach for producing improved crop varieties (Masoomi-Aladizgeh et al., 2015). Crop wild resources can provide a variety of traits to cultivated crops with the potential to decrease yield loss due to various environmental stresses (Kiani et al., 2015; Rafique et al., 2017; Mammadov et al., 2018). Genetic diversity in wild relatives of wheat is an ideal source for producing new tolerant varieties predominantly for drought, salinity, cold, and heat stresses (Rasheed et al., 2018). Wheat wild relatives are categorized into three gene pools: primary, secondary, and tertiary gene pools (Zhang et al., 2017). The primary gene pool includes hexaploid landraces, cultivated tetraploids, A and D genome diploid donors, and wild T. dicoccoides. The secondary gene pool includes Aegilops species and polyploid Triticum. Further, the tertiary gene pool includes Secale cereale, Thinopyrum intermedium, and species of the genus Elymus (Chaudhary et al., 2014).
Elymus L. is the largest genus of the family Poaceae and tribe Triticeae, comprising about 150 species mainly distributed in temperate zones of the northern hemisphere (Dewey, 1984). Cytologically, species of the genus Elymus contain St, H, Y, P, and W subgenomes from Pseudoroegneria, Hordeum, Agropyron, and Australopyrum genomes, respectively (Jensen, 1990). In Pakistan, the genus is represented by 22 species (Cope, 1982). Due to compact and determinate floral structure, self-pollination ability, and other characteristics, several Elymus species have been developed as forage cultivars, including E. glaucus, E. lanceolatus, E. Canadensis, E. trachycaulus, E. wawawaiensis, and E. virginicus (Aubry et al., 2005). Species of the genus Elymus have close taxonomic relations to wheat, rye, and barley and thus serve as a genetic reservoir of potential alien genes for improving these crops against stresses and other agronomic traits (Sasanuma et al., 2002). Recently, the introgression of Elymus repens-3St chromatin into wheat and its characterization by Gong et al. (2019) has demonstrated high resistance to stripe rust and Fusarium head blight by the translocation lines in comparison to the wheat parent.
Species of the genus Elymus have received much attention, as they carry various characteristics such as resistance to environmental stresses, diseases, and pests (Schooler and Anderson, 1980; Aung, 1991). Some species like Elymus dahuricus, E. nutans, and E. elongatus are considered potential sources for desirable traits like salinity, drought, and cold tolerance (Gorham et al., 1984; Gazanchian et al., 2007). Therefore, these species might be beneficial as tertiary genetic resources for improving common bread wheat and other forage grasses (Zhao et al., 2013). Drought adaptability of the Elymus species may be explored through detailed characterization of its physio biochemical and anatomical attributes is essential. The current study aimed to investigate the water stress responses of Elymus species and explore potential species to be utilized in wheat breeding for drought tolerance.
Materials and methods
Plant specimen and seed collection
Plant samples and seeds of Elymus (Triticeae) were collected from northern Pakistan's temperate and alpine regions in July–September 2019. Northern Pakistan encompasses northern Khyber Pakhtunkhwa and Gilgit-Baltistan. Geographically, the region lies in a temperate climate zone, between 34° and 37°N and 71.4° and 77.6°E, with high alpine meadows, glaciers, snow-covered peaks, high waterfalls, and coniferous forests (Ullah et al., 2015). The region is a meeting point for the world's three highest mountain ranges, i.e., the Himalayas, Karakorum, and Hindukush, and is home to some of the highest peaks, including K2, the world's 2nd highest peak. The region is also rich in diverse ethnicities, languages, cultures, and religious beliefs (Abbas et al., 2016). The huge topographic, edaphic, and climatic variability gives rise to diverse and rich flora and fauna. The climate in the upper elevations is extremely harsh, with little rain and plenty of snow, and the areas are described as snow deserts. The temperature in winter may fall well below freezing. The summers in low-lying areas are pleasant. These mountain ecosystems are home to every third species of plant and provide plenty of ecosystem services (Khan et al., 2012). Elymus is a common component of these alpine and subalpine meadows.
A sampling of plants and seeds was collected from eight Elymus species, including Elymus longiaristatus (Boiss.) Tzvelev, E. dahuricus Griseb., E. schrenkianus (Fisch. & C.A.Mey.) Tzvelev, E. semicostatus (Nees ex Steud.) Melderis, E. nutans Griseb., E. russelli (Melderis) Cope, E. borianus (Melderis) Cope, and E. caninus (L.) L.), growing in wild habitats in northern Pakistan. During field collection, the field notebook noted geographic coordinates, associated species, phenology, and habitat features for each specimen locality. The specimens were dried and preserved, and voucher specimens were submitted to the Swat University Herbarium (SWAT). Botanical taxa were identified by expert taxonomists using the flora of Pakistan (Cope, 1982). Seeds of two wheat varieties, Pirsabak-2005 and Faisalabad-2008, were executed at the National Agriculture Research Center (NARC), Islamabad, Pakistan (Table 1).
Germination experiment
Seeds of eight Elymus species and two wheat genotypes were sterilized for 10 min with 5% sodium hypochlorite (NaOCl) and rinsed thoroughly with deionized water. Ten seeds of each species replicated three times were germinated under osmotic stress conditions induced by polyethylene glycol (PEG6000). Three treatments, i.e., 0% (control), 7.5%, and 15% PEG (w/v), were used in a completely randomized design (CRD) to explore variations in germination characteristics of the experimental plant (Ali et al., 2015). Germination percentage, GP (Larsen and Andreasen, 2004); mean germination time, MGT (Ellis and Roberts, 1981); and germination index, GI [Association of Official Seed Analysts (AOSA), 1983] were calculated accordingly.
Pot experiment
An experiment was carried out in a greenhouse at the Center for Plant Sciences and Biodiversity, the University of Swat, to compare the physio, biochemical, and anatomical responses of the experimental plant materials, comprising Elymus species and wheat cultivars, under water stress conditions. Experimental soil used as a growth medium in the experiment was collected from the Agriculture Research Institute Mingora Swat, Pakistan, which was thoroughly examined for its physical and chemical properties (Supplementary material 1). Seeds were germinated for all the species, and uniform-sized seedlings were transplanted into plastic pots (24 × 18, 30 cm) filled with the same soil and exposed to sunlight for better growth with an average daily temperature of 20/26°C and 65% humidity. The field capacity (FC) of the soil was determined gravimetrically, as previously discussed in Graber et al. (2010). Treatments in the pot experiment were applied as previously reported in Liu and Li (2005), which included control (80% FC, i.e., well-watered condition), moderate stress (MS; 55% FC), and severe stress (SS; 30% FC). The experimental design employed during the study was a randomized complete block design (RCBD) replicated three times. Accordingly, each pot was watered to its field capacity until 60 d of seedlings were transferred, after which water stress was initiated. Water stress was monitored by weighing each pot daily and adding water lost by evapotranspiration till the respective FC regimes were retained. Normal weeding and agricultural practices were carried out to provide proper environmental conditions. Thirty days after the imposition of stress, when wilting and curling appeared in SS treatment, an indication of permanent wilting, leaf samples were collected, weighted, wrapped in aluminum foil, and kept in a refrigerator for determination of various anatomical, physiological, and biochemical attributes.
Micromorphological characterization of leaf epidermis
For obtaining epidermal leaf peel, about a 2 cm long segment from the mid-portion of five mature leaves was taken and boiled in 88% lactic acid in a water bath at 100°C for about 30 min (Clark, 1960). The leaves, softened with lactic acid, were subjected to the removal of abaxial and adaxial epidermal peels using a sharp razor blade. Epidermal peels were stained with 1% safranin and placed in glycerol or lactic acid on glass slides. Qualitative and quantitative features of the epidermis were observed and measured using an Olympus light microscope equipped with a digital camera (Meiji infinity DK-5000, Japan). Qualitative traits included cell shape, cell wall morphology, presence, and absence of stomatal complexes and prickles, while quantitative traits included long cell length (LCL), long cell width (LCW), stomatal complex length (SCL), and stomatal complex width (SCW).
Anatomical characterization of leaves and culm
An anatomical investigation of leaves and culm from all experimental plant materials was carried out to explore variations in response to drought stress treatments following the method of Ruzin (1999). This was accomplished by taking small fragments of flag leaf and culm of all the studied plant species when permanent wilting appeared under severe stress treatment. Specimens were then fixed in formalin acetic alcohol (FAA) solution, which comprised formalin (10 mL), 70% ethyl alcohol (85 mL), and glacial acetic acid (5 mL) for 24 h. Freehand sectioning was employed for cutting sections (~20 μm), which were then serially dehydrated by ethanol (30, 50, 70, and 90%, respectively). In order to enhance visibility, the sections were double stained with safranin—light green. The prepared slides were then analyzed through a light microscope, which was followed by photomicrography for five sections per replication. Various internal anatomical features of the culm, including phloem length (PL), phloem width (PW), vascular bundle length (VBL), vascular bundle width (VBW), and ground tissue thickness (GT), were recorded. Similarly, leaf upper epidermis thickness (UET), lower epidermis thickness (ET), xylem vessels diameter (XVD), phloem length (PL), phloem width (PW), vascular bundle length (VBL), vascular bundle width (VBW), and mesophyll thickness (MT) were also recorded.
Physiological and biochemical analysis
Chlorophyll content was measured by following the method described in Ali et al. (2014b) using dimethyl sulphoxide (DMSO). Proline content in leaf samples was determined using 3% sulfosalicylic acid according to the method of Bates et al. (1973). The determination of protein was performed following the method of Lowry et al. (1951), using bovine serum albumin (BSA) as a standard. Soluble sugar was determined by following the method described by Dubois et al. (1956) using phenol sulfuric acid. Antioxidant enzymes were extracted as described in Ali et al. (2014a,b), with minor modifications. ~0.5 g of fresh plant tissues were ground in 10 mL of 50 mM chilled phosphate buffer in an ice bath using a pestle and mortar. The homogenate was collected and filtered through cloth. It was then centrifuged at 15000 rpm for 25 min at 4 °C (Bian and Jiang, 2009). Finally, the supernatant was used for antioxidant enzymatic activity analysis. An assay for superoxide dismutase was carried out by using the method of Giannopolitis and Ries (1977). Peroxidase activity was determined by following the method described by Gorin and Heidema (1976).
Statistical analysis
The obtained data were subjected to analysis of variance (ANOVA) using STATISTIX 10.0. as described in Steel et al. (1997). The data was also subjected to principal component analysis (PCA) using a multivariate option in PAST v. 2.12. A heatmap of Pearson's correlation was created to visualize the association between different variables. Summary statistics of the studied anatomical traits were graphically visualized through box-violin plots, which were prepared by utilizing the Jamovi software (version 1.8; The jamovi project, 2021) and package ggplot2 (Wickham et al., 2018). The relative difference among treatment means of all the studied attributes was calculated as previously described in Ahmadi et al. (2018) by using the formula:
where and are the means of a studied trait under controlled and water stress treatments.
Results
Results revealed that both osmotic and water stresses (moderate, FC 30%, and severe FC 50%) significantly affected all the studied morpho-physiological and biochemical attributes in Elymus species. and wheat genotypes. The mean square results of the studied traits showed that MGT, GI, GP, PRC, Chla, Chlb, TChl, and SOD were highly different in osmotic and water stress treatments. However, no significant differences were observed regarding SS, PC, and POD. Similarly, PC, PRC, Chla, Chlb, and TChl were found to be significantly different in water stress treatments (Table 2).
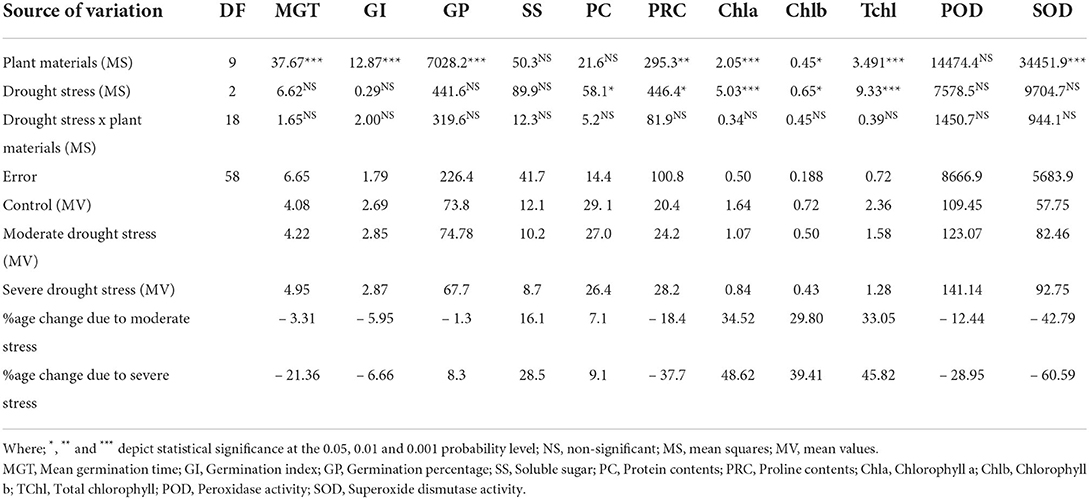
Table 2. Analysis of variance (mean squares), mean values, and percentage change in biochemical and physiological traits in the studied Elymus species and wheat cultivars.
Germination characteristics
Water stress increased mean germination time (MGT) and germination index (GI). However, the studied plant materials reduced germination percentage (GP) under osmotic stress conditions. The observed increase in MGT was 3.3%, GI 5.9% under moderate stress, while it was 21.4% and 6.7% under severe stress, respectively. However, mean GP declined by 1.3% in moderate and 8.3% under severe water stress (Table 2). A maximum increase in MGT (32.1%) was recorded in E. schrekianus under moderate stress, while a minimum increase of 4.4% was recorded in E. borianus (Table 3). Similarly, maximum increased GI (73.6%) was observed in E. caninus, while minimum increase (8.0%) in E. borianus. A maximum reduction in germination percentage of 5.6% was observed in E. nutans under moderate stress (7.5% PEG). Similarly, E. semicostatus exhibited a decline in germination percentage (31.2 and 81.2%) under moderate and severe osmotic stress, respectively (Table 3).
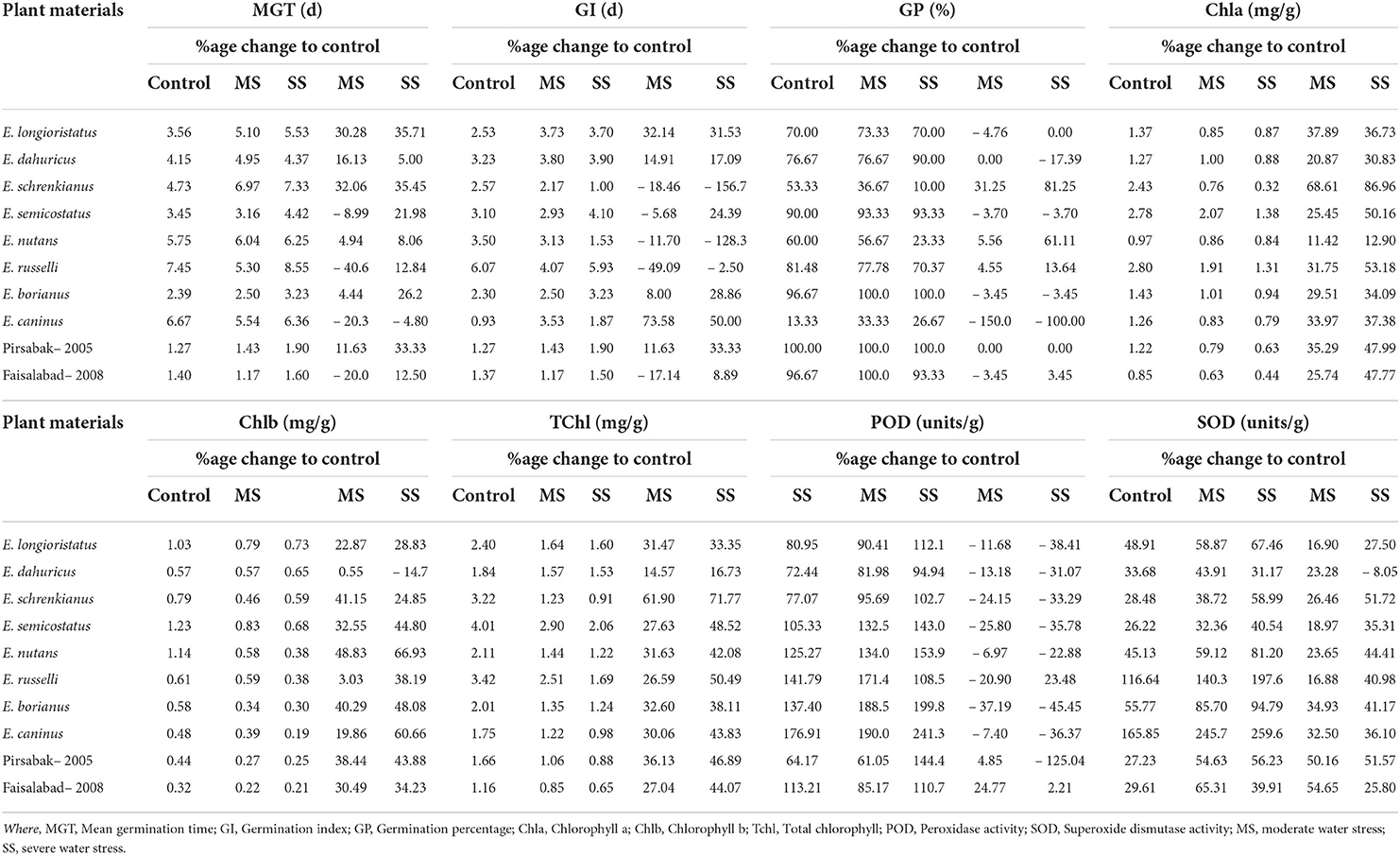
Table 3. Variability in mean germination time, germination index, germination percentage, chlorophyll contents, and antioxidants enzymatic activities among Elymus species and wheat cultivars under drought stress.
Photosynthetic pigments
Photosynthetic pigments were also affected by water stress in comparison to control treatment. The average Chla content declined by 34.5%, Chlb by 29.8%, and TChl by 33.1% under moderate stress, while the decrease was 48.6, 39.4, and 45.8% under severe stress, respectively (Table 2). The maximum reduction in Chla content (68.6 and 86.9%) among Elymus species was recorded in E. schrenkianus under moderate and severe water stress, respectively. However, E. nutans was the least affected species, exhibiting 11.4 and 12.9% reduced Chla. Similarly, a maximum percentage decrease in Chlb of 48.8 and 66.9% was also recorded in E. nutans. In E. dahuricus, Chlb was reduced by 0.5% only under moderate water stress; however, the reduction was 14.67% under severe water stress. A maximum percentage decrease in TChl was recorded in E. schrenkianus, reduced by 61.9 and 71.8% under moderate and severe stress, respectively (Table 3). SOD and POD activity increased by 42.8 and 12.4% in moderate and 60.6 and 28.9% under severe water stress, respectively. Maximum POD in terms of percentage change was 37.2 and 45.4%, reported in E. borianus under MS and SS, respectively. The maximum change in SOD activity was reported in E. borianus and E. schrenkianus, in which SOD activity increased by 34.9% under MS and 51.7% under severe water stress (Table 3).
Biochemical attributes
Biochemical attributes were significantly affected by both moderate and severe water stresses. Protein contents declined by 7.1 and 9.1% in moderate and severe water stress, respectively (Table 2). A minimum reduction in PC (1.7 and 2.1%) was reported in E. russelli under MS and SS treatment, respectively, which was followed by E. dauhuricus—which exhibited a 2.0 reduced PC under MS treatment. Similarly, SS declined by 16.1 and 28.5% in moderate and severe water stress, respectively. A maximum decrease in SS (38.5 and 49.8%) due to moderate and severe water stress was observed in E. semicostatus. Proline content increased by 18.4% in moderate water stress and 37.7% in severe water stress. When a comparison was made with the control treatment, increased PRC (71.0, 54.9, 51.3, and 35.6%) was observed in E. borianus, E. dahuricus, E. schrenkianus, and E. caninus under MS and SS, respectively (Table 4).
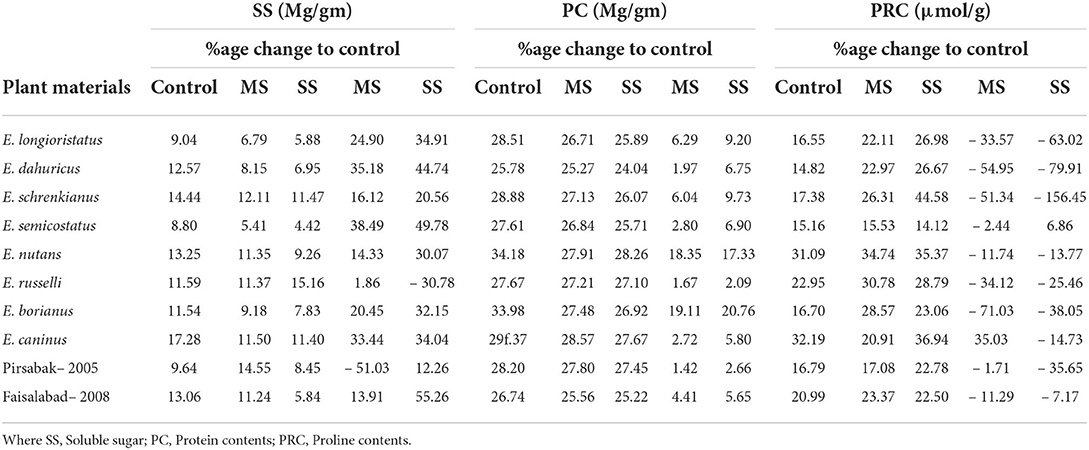
Table 4. Variability in soluble sugars, protein content, and proline content in Elymus species and wheat cultivars under drought stress.
Characterization of anatomical features
Regarding leaf epidermal anatomy, high differences were revealed between experimental plant materials and drought stress treatments for the studied traits, including AbLCL, AbLCWD, AbSCL, AbSCW, AdLCL, AdLCW, AdSCL, and AdSCW. However, the interaction between Elymus species and drought stress treatments yielded differences with respect to AbSCL and AdSCL only. Among the studied traits, AbSCL and AdSCL were the most persistent, with an an18.8 and 20.9% coefficient of variation (CV), respectively. The long cell length of both abaxial and adaxial surfaces decreased by 12.2 and 9.3% in MS, while it was reduced by 18.2 and 14.6% in SS, respectively. Similarly, the LCW of both surfaces decreased by 9.6 and 8.1% in MS, while 14.9 and 14.8% in SS, respectively. The stomatal length of the abaxial and adaxial surfaces increased by 4.8 and 1.1% in MS, while 8.1% and 7.5% in SS, respectively. Stomatal width of the abaxial and adaxial surface declined by 6.1 and 5.2% in the MS, while it decreased by 8.6 and 11.1% in SS). In general, drought stress reduced all the traits of both epidermal surfaces in studied Elymus species and wheat genotypes (Figures 1, 2; Supplementary materials 2, 5).
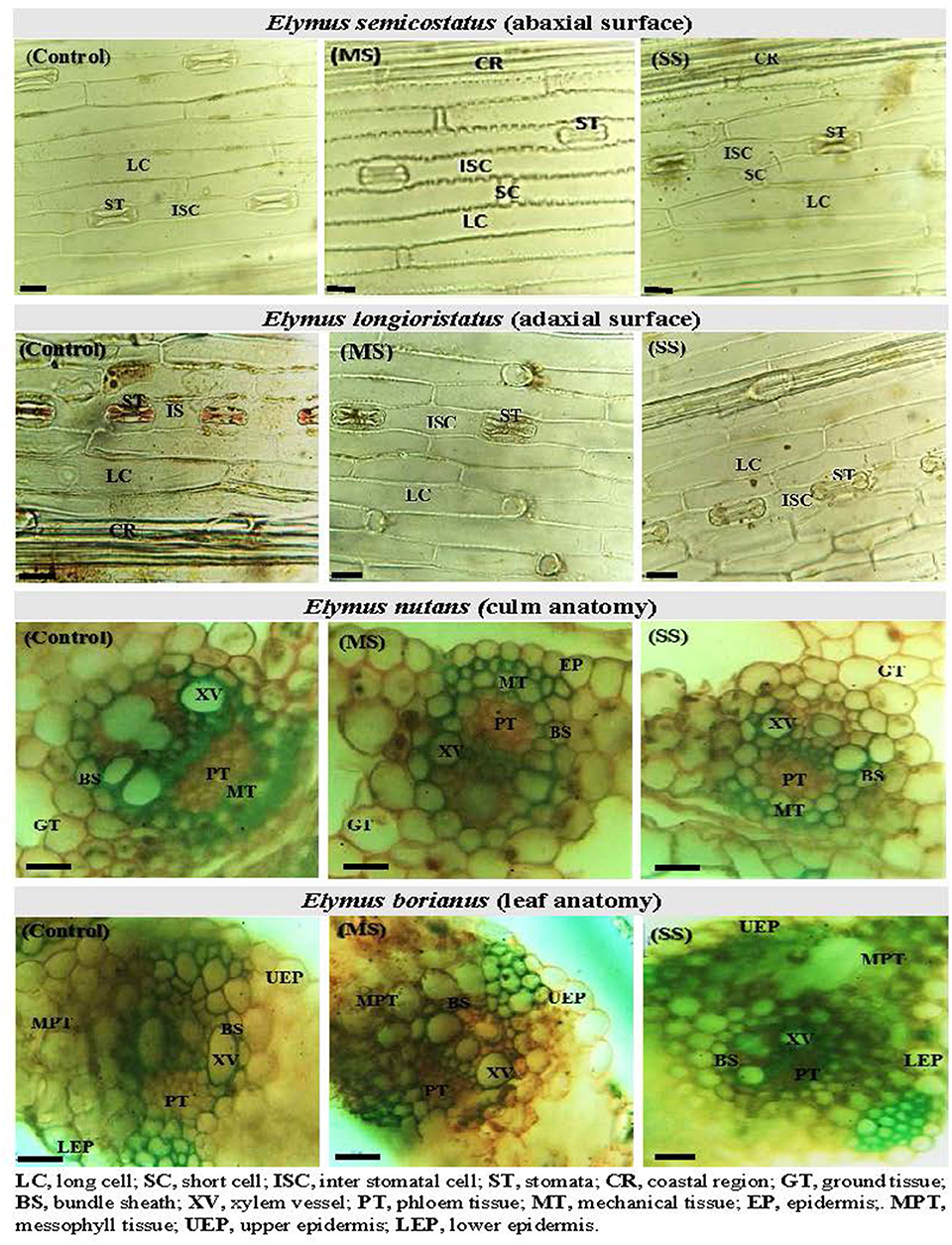
Figure 1. Leaf epidermal anatomy (abaxial surface and adaxial surface) of Elymus longioristatus; culm and leaf anatomy of E. nutans under control (C), moderate (MS), and severe (SS) water stress conditions. Bar scale 100 μm.
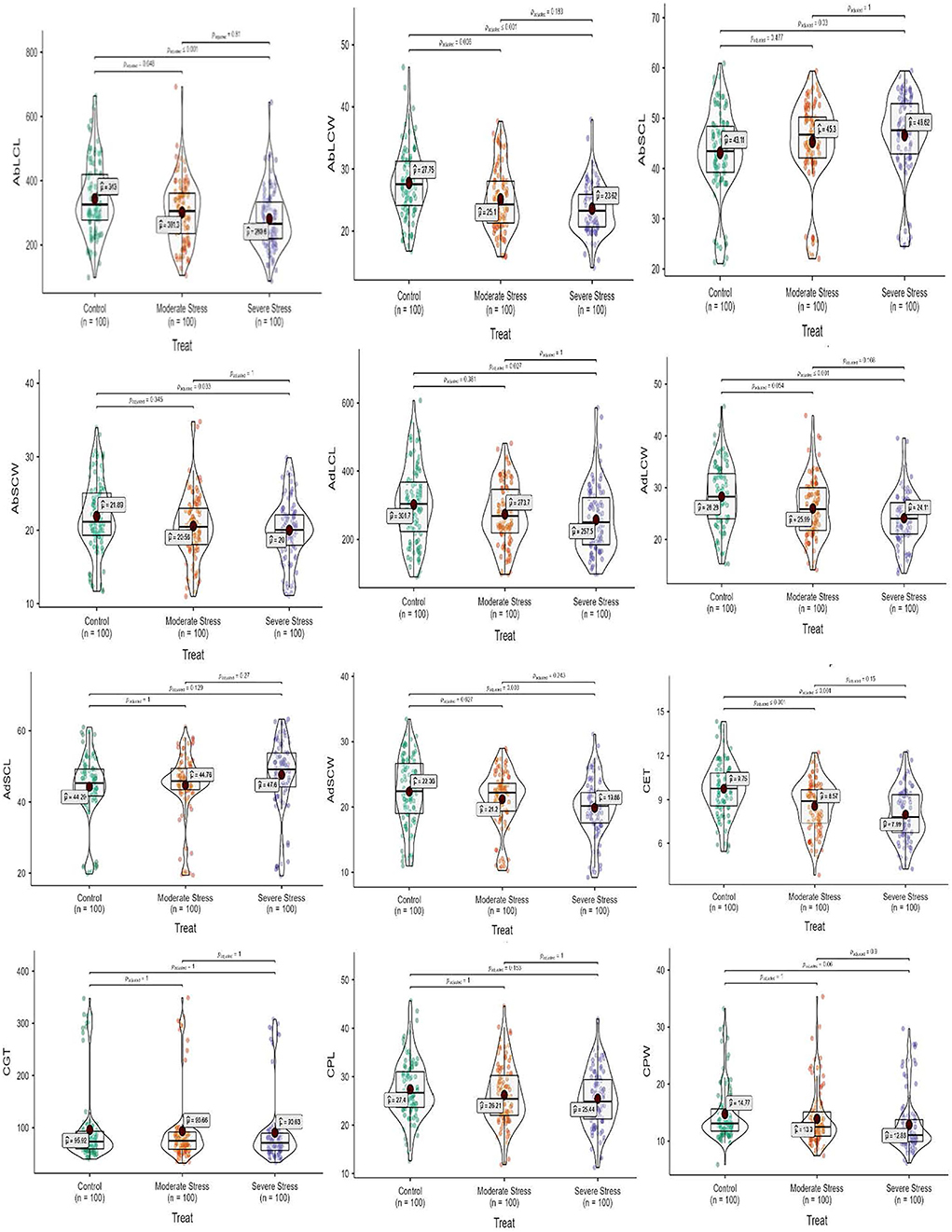
Figure 2. Box-violin plots presenting leaf epidermal (abaxial and adaxial surface) and culm anatomical features under control (C), moderate (MS), and severe (SS) water stress conditions. The interquartile range is depicted by the length of the boxes, the median by the horizontal line within the box, the mean by the red diamonds within the box, and the probability density of the data at different values by the widths of the violin plots.
Mean squares of the culm anatomical features revealed that epidermal thickness, xylem vessel diameter, phloem length and width, vascular bundle length and width, and ground tissue thickness were highly different in the studied plant materials and drought stress treatments. However, no such difference was observed in the interaction between plants and stress treatments. The epidermal thickness of the culm declined by 12.1 and 18.0% under MS and SS, respectively. Culm xylem vessel diameter decreased by 7.9 and 16.5% in the MS and SS. Culm phloem length and width declined by 4.4 and 5.9% in MS and by 7.2 and 12.9 under SS, respectively. Similarly, vascular bundle length and width declined by 3.1 and 2.6% in MS, while 6.0 and 4.1% in SS, respectively. In general, drought stress reduced all culm anatomical traits in studied Elymus species and wheat genotypes (Figures 1–3; Supplementary materials 3, 6).
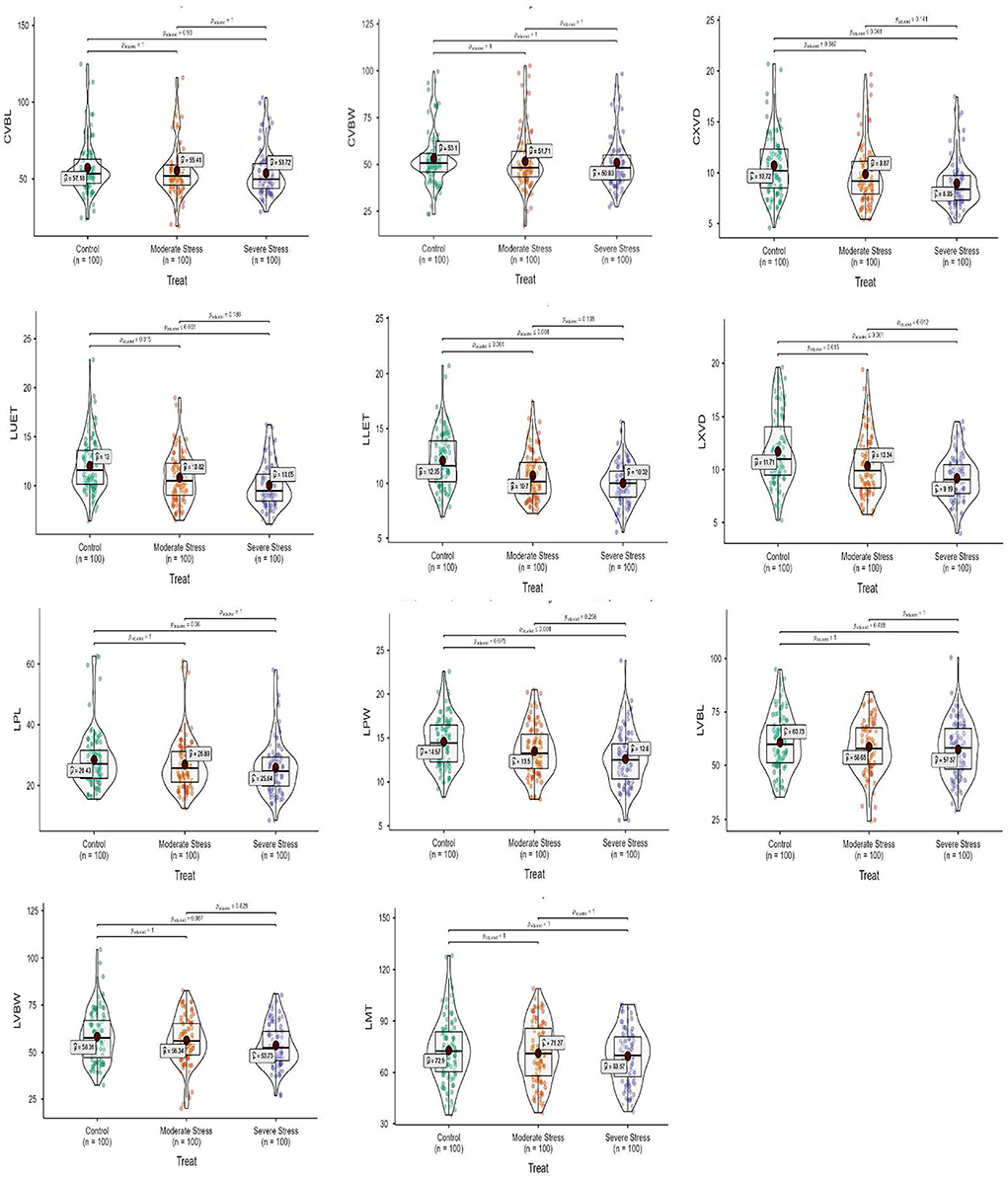
Figure 3. Box-violin plots presenting culm and leaf anatomical features under control (C), moderate (MS), and severe (SS) water stress conditions. The interquartile range is depicted by the length of the boxes, the median by the horizontal line within the box, the mean by the red diamonds within the box, and the probability density of the data at different values by the widths of the violin plots.
Mean squares of leaf anatomical traits indicated that lower and upper epidermal thicknesses, xylem vessel diameter, phloem length and width, vascular bundle length and width, and mesophyll tissue thickness were different among Elymus species and drought stress treatments. However, the interaction between the studied plant materials and drought stress treatments exhibited differences with respect to leaf xylem vessel diameter only. Leaf upper and lower epidermal thickness decreased by 9.8 and 11.1% in MS, while 16.2 and 16.8% in SS, respectively. Similarly, xylem vessel diameter declined by 11.7%, phloem length by 5.4%, phloem width by 7.3%, vascular bundle length by 3.4%, vascular bundle width by 3.4%, and mesophyll tissue thickness by 2.2% under MS, while these declined by 21.6, 9.1, 13.6, 5.5, 7.8, and 4.6% under SS, respectively. Generally, all the studied leaf vascular anatomical traits were significantly reduced under both water stress conditions (Figures 1, 3; Supplementary materials 4, 7).
Correlation and principal component analysis
A heatmap showing the Pearson correlation coefficient and associated probabilities (p ≤ 0.05, ≤ 0.01, ≤ 0.001, respectively) among Elymus species and wheat cultivars for the studied traits is shown in Figure 4. The Pearson correlation showed the highest positive correlation between MGT and SOD (r = 0.812), followed by POD (r = 0.693) in the control condition, while it was non-significant under moderate and severe water stress. Similarly, a significant relationship was observed between MGT and GI (r = 0.707) in moderate water stress, which decreased in control (r = 0.473) and severe stress (r = 0.365), respectively. The relationship between POD and SOD (r = 0.759) was prominent in the control condition during its decline in moderate stress. A similar trend was also observed for anatomical traits, including CXVD and LXVD.
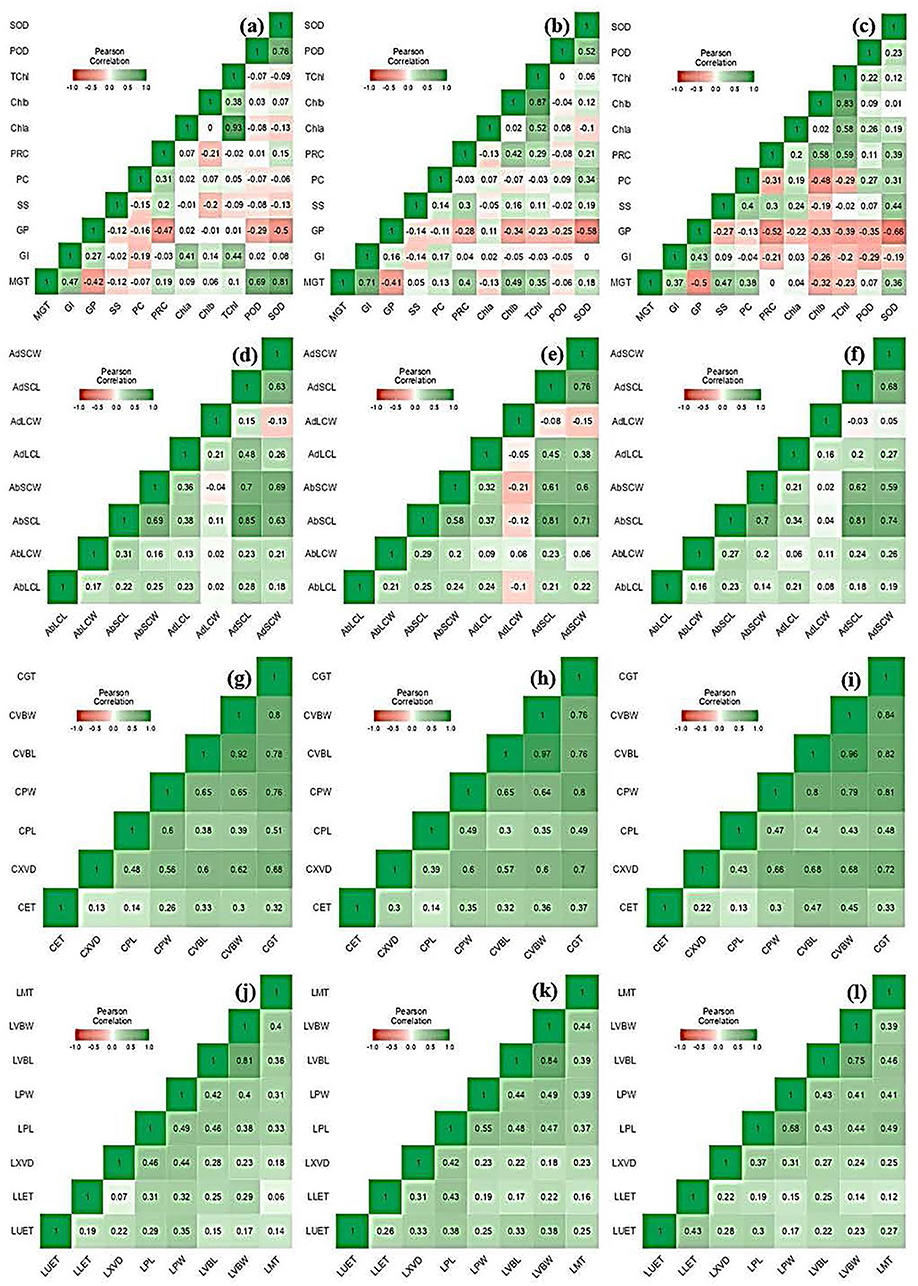
Figure 4. Heatmap showing Pearson correlation coefficient and associated probabilities among Elymus species and wheat cultivars for the studied traits using (n = 10) evaluated under osmotic (germination) moderate (MS; FC = 50%) and severe (SS; FC = 30%) water stress. (a) control, (b) MS, and (c) SS showing a relationship for morphological and physio biochemical traits; (d) control, (e) MS, and (f) SS showing a relationship for leaf micromorphological traits; (g) control, (h) MS, and (i) SS showing relationship for culm anatomical traits; and (j) control, (k) MS, and (l) SS showing relationship for anatomical and traits. The values in the color bar correspond to the Pearson correlation coefficient. Significant correlations are shown in green (positive) and red (negative) colors at p ≤ 0.05, ≤ 0.01, ≤ 0.001, respectively.
In search of finding the appropriate grouping of the studied traits in response to progressive water stress among Elymus species and wheat cultivars, principal component analysis (PCA) was carried out through mean values (Figure 5, Supplementary material 8). It was predicted that trait vectors exhibiting angles ≤ 90° have a positive relationship, while those with angles depict negative associations ≥90°. Further, the length of the vector in the PCA explains the extent of variation explained by the corresponding trait. Current PCA revealed up to 62.8% variability being explained by the first two axis i.e., PC1 (eigenvalue = 8.12) and PC2 (eigenvalue = 3.82). Considering the first 2 PCs, we observed that mostly morpho-physiological, biochemical, and leaf micromorphological traits contributed to PC1, while the attributes with a major contribution to PC2 were mostly anatomical (culm and leaf). Regarding contribution to PC1, the observed order of the traits included POD (0.83), ABLCL (0.82), SOD (0.74), PC (0.57), ADLCL (0.56), SS (0.52), and PRC (0.36). Similarly, regarding contribution to PC2, the observed contributing traits were ADSCL (0.80), ADLCL (0.79), LPL (0.79), ADSCW (0.74), CET (0.72), CVBW (0.66), LVBL (0.65), CVBL (0.65) and CXVD (0.51). For PC3, CXVD (0.61), CET (0.57), CVBW (0.45), CPL (0.442), and CVBL (0.44) were noted for their prominent contributions, while for PC4, only ADLCL (0.73), GP (0.48), and POD (0.38) were noted for their prominent contributions.
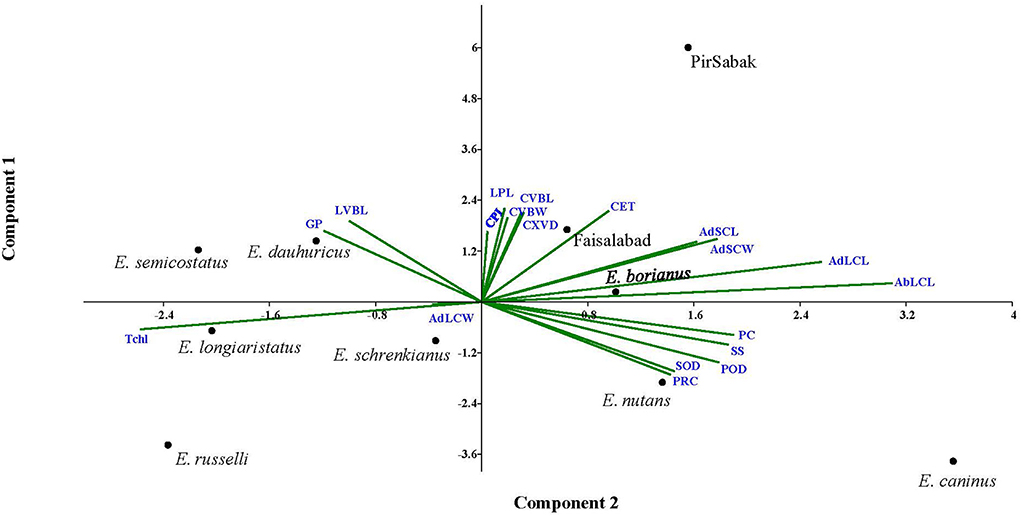
Figure 5. Principal component analysis (PCA) of the studied traits in Elymus species and wheat cultivars. Trait vectors exhibiting angles ≤ 90° have a positive relationship, while those with angles depict negative associations ≥ 90°. PCA revealed up to 62.8% of the variability being explained by the first two PCs.
Discussions
Germination and development of seedlings under laboratory conditions are considered suitable for evaluating plant responses to abiotic stresses. In the current study, the GPs of all the studied Elymus species were reduced significantly. It is a general observation that seeds experiencing osmotic stress hinder their ability to start the germination process efficiently, which may be correlated to the associated change in the activities of enzymes (Wei et al., 2021). This, in turn, leads to shrinkage in cell walls, lowering of turgor-dependent activities, including root elongation and leaf expansion, and ultimately, affecting crop establishment. Our results support the findings of Wu et al. (2019), who reported that an increase in PEG concentration resulted in increased MGT and reduced GP in Echinochloa crusgalli. Our results support the findings of Zhang and Nan (2010), who observed reduced germination in E. dahuricus populations under different osmotic potentials.
The photosynthetic efficiency of a plant in terms of light-harvesting capability is generally predicted through the exploration of its chlorophyll content (Ali et al., 2014b; Ayub et al., 2021). Plants exhibiting higher chlorophyll content are considered an index of drought tolerance. Hence, selection based on these traits may prevent growth inhibition and a reduction in yield under water stress (Ahmadi et al., 2018). In the present study, a mild decreasing trend in all the studied Elymus species and wheat cultivars were recorded regarding chlorophyll content, which may be attributed to their different tolerance responses and enhanced antioxidant and osmoprotectant activities under moderate and severe water stresses, respectively. This degradation of the absorbing pigments may be regarded as an avoidance mechanism since the production of ROS is mainly associated with excess energy absorption within the photosynthetic apparatus (Bouchemal et al., 2017). Further, variable reduction rates in chlorophyll content are also suggestive of the existence of genetic diversity among the studied Elymus species. Reports of such mild decreases or little altered chlorophyll contents have been reported by Xu and Zhou (2007) in Leymus Chinensis and Pour-Aboughadareh et al. (2017) in Aegilops-Triticum accessions.
Accumulation of compatible solutes, including Dhn (dehydrins) and LEA (late embryogenesis abundant) proteins, under scarce water availability, is indicative of plant drought tolerance. However, certain other proteins decline under water deficit conditions, and the extent of decline or accumulation depends largely on the genetic constitution of different plant species (Ahmadi et al., 2018). Similarly, soluble sugars are part of plant hydrolysis processes, have a role in signaling and sensing systems, and play a crucial role in plant metabolism. Soluble sugars also have a distinct osmoprotectant role in maintaining turgor pressure and stabilizing cellular membranes. Hence, differences in the accumulation of soluble sugars are considered among the genetic factors affecting plant performance under water deficit conditions. In the current study, a slight decline in protein content and enhanced accumulation of soluble sugars were observed, which indicated the existence of drought adaptive mechanisms in the studied Elymus species. A similar decrease in protein concentration and enhanced accumulation of soluble sugars under water deficit conditions have previously been reported by Hessini et al. (2009) and Xu and Zhou (2007), respectively. However, our findings do not support the findings of Borrajo et al. (2018), who reported an increase in protein content with an increase in the severity of drought stress, which may be due to differences in experimental plant materials and conditions of drought stress imposition. Further, the increased accumulation of soluble sugars may be attributed to reduced utilization of assimilates during drought due to inhibition of invertase or sucrose synthase activities (Lv et al., 2020).
Osmotic adjustment is a key mechanism of plants under drought stress conditions to maintain water uptake and cell wall pressure, keep stomata open, and keep physiological and biochemical processes intact (Chaves et al., 2009; Cyriac et al., 2018). Improved proline accumulation under water deficit conditions is considered advantageous as osmoregulation and desiccation protectant and, generally, is recommended as criteria for selection tolerance against drought stress (Gazanchian et al., 2007; Hessini et al., 2009; Noein and Soleymani, 2022). Our findings suggest that higher proline content improves a plant's ability to tolerate osmotic stress. Further, relative increase rather than absolute value could be the appropriate indicator of stress tolerance. The present study also revealed enhanced proline content in moderate and severe water stress. The findings of Ahmadi et al. (2018), Martinez et al. (2018), and Borrajo et al. (2018) are in general agreement with the results obtained in the current research, which suggests that the studied Elymus species may be a potential source for this important trait for tolerance to water deficit environments.
As a plant suffers from a water deficit condition, it not only experiences disturbance in cell turgidity and reduction in growth, but it also suffers from oxidative stress due to the presence of ROS. Removing these ROS plants possesses antioxidant mechanisms (Zhang and Nan, 2007; Bouchemal et al., 2017; Suneja et al., 2017). Peroxidase (POD) and superoxide dismutase (SOD) are major enzymes that play a key role in the defense mechanism of plants against various stress conditions by scavenging H2O2 in chloroplasts (Gill and Tuteja, 2010). Increased antioxidants, including superoxide dismutase and peroxidase, have an important role in coping with the oxidative damage caused by free radicals. Thus, they are considered important indices for assessing plant redox status and can be used to select drought-tolerant plants (Alscher et al., 2002). In the current study, drought stress up-regulated the activity of the antioxidative defense system, and we observed variable but enhanced SOD and POD activities in the studied plant materials, which suggests that the varied ROS-scavenging ability of Elymus species is a contributor to its improved drought tolerance. Our results generally agree with those reported by Suneja et al. (2017) and Ahmadi et al. (2018), who reported enhanced antioxidant activities in moderate and severe water stress compared to control.
A PCA biplot analysis can be utilized to select traits that can be categorized into main groups and subgroups based on homogeneity and dissimilarity. In our result, three groups of traits were identified in the PCA biplot, considering both PC1 and PC2 simultaneously. The PRC, SOD, POD, SS, and PC were clustered in group I, while CPL, LPL, CXVD, CVBL, CVBW, and CET were in group II, and GP and LVBL were in group III. Traits in the same group may be interpreted to exhibit closely related physio-biochemical and anatomical relationships (Abdi and Williams, 2010). Other researchers have widely and effectively used PCA biplot analysis for screening stress tolerance of experimental plant materials (Nouraein et al., 2013; Ali et al., 2015; Ahmed et al., 2019).
Diverse mechanisms through which adaptations to water stress are induced may include changes in the anatomy of plant root, stem, and leaf tissues that monitor the transfer of deleterious effects of elevated CO2 concentration and water deficit conditions across the cells (Engloner et al., 2003; Boughalleb et al., 2014). There was a decreasing trend in the studied culm anatomical attributes with increasing water deficit. For instance, phloem length and width, epidermal and ground tissue thickness, xylem vessel diameter, and vascular bundle length and width all exhibited progressive reduction with an increase in the severity of the water stress. These adaptive anatomical traits may be used to identify tolerant Elymus species in water-stress environments. Through current genetic engineering and molecular methods, they can be targeted for integration into susceptible kinds of wheat (Nassar et al., 2020; Liu et al., 2021). Reduced xylem vessel diameter in Elymus species subjected to moderate water stress may be related to the maintenance of the water conductivity, which may contribute to reduced susceptibility to xylem embolism (Lovisolo and Schubert, 1998; Haworth et al., 2017; Dolezal et al., 2019).
Regarding phloem length and width, the observed decrease in the water deficit environment may also be assumed as an adaptation strategy because water stress resulted in reduced growth in comparison to controlled treatment. The decline in both leaf and culm xylem vessel diameter may also be regarded as advantageous as it may reduce cavitation of the xylem vessels, which further suggests that Elymus species can thrive well in drought-stress environments through different anatomical adaptations. A similar reducing trend in anatomic attributes under progressive water stress has also been previously reported by El-Afry (2012), Selim and El-Nady (2011), Arantes et al. (2020), and David et al. (2017).
To save water, leaf anatomical adaptation to progressive water stress is also considered important for selection against plant drought tolerance (Boughalleb et al., 2014). In the present study, moderate and severe water stress highly affected leaf anatomical traits in the studied Elymus species and wheat cultivars. Similarly, we observed that while confronting moderate to severe water stress, a significant decrease in xylem vessel diameter, leaf lower and upper epidermal thicknesses, vascular bundle length and width, mesophyll tissue thickness, and phloem length and width were observed among Elymus species. This declining trend in leaf anatomical traits may be regarded as an important structural response to progressive water stress, probably affecting the conductance of CO2 diffusion (Wang et al., 2011). Hence, reduced tissue size in Elymus culm and leaves may be interpreted as a tolerance mechanism for maintaining turgor even under a lesser amount of water. We noted that progression from moderate to severe water stress led to a decrease in bundle sheath dimensions and a reduction in the thickness of both the xylem and phloem, suggesting the ability of the leaf to maintain water transport even under unfavorable conditions. For some of the studied attributes, slight enhancement was observed under moderate stress, which declined under severe stress. This signifies that Elymus grass may have leaf trait plasticity in response to unfavorable environmental conditions. Reduced stomatal length and width in comparison to controlled grown plants were also observed in all the studied Elymus species and wheat cultivars. This may be regarded as a dehydration avoidance mechanism for sustaining proper water status and reducing water loss under water deficit conditions (Xu and Zhou, 2008; Thangthong et al., 2019). Our findings are in general agreement with those previously reported by Wang et al. (2011), Selim and El-Nady (2011), Petrov et al. (2018), Arantes et al. (2020), Chartzoulakis et al. (2002), and Bosabalidis and Kofidis (2002). David et al. (2017), El-Afry (2012), and Selim et al. (2019).
Improvement in cultivated kinds of wheat may/can be achieved through approaches like mutation breeding, genetic transformation, and hybridizing wild resources (Ilyas et al., 2015; Kazi et al., 2015). Owing to the exceptional stress tolerance and disease resistance potential of the Elymus genus, it is considered a valuable gene pool that can be used for the genetic enhancement of crops like wheat, rye, barley, and wheat (Yang et al., 2017; Xiong et al., 2021; Tan et al., 2022; Zhang et al., 2022). For instance, ESTs identified by Habora et al. (2012) may locate introgression of Leymus chromosomes having genes linked with stress tolerance in cultivated wheat, which in turn can assist marker-assisted breeding. Similarly, E. dahuricus, which is found mainly in temperate zones, has been reported to be potentially salt tolerant (Borrajo et al., 2018). Moreover, E. elongatus subspecies ponticus has been reported to possess high water use efficiency and hence is regarded as potentially drought tolerant (Gazanchian et al., 2007). Elymus wawawaiensis is a long-lived and drought-tolerant wheat grass. Elymus wawawaiensis, E. Canadensis, E. nutans, and E. glaucus are also considered novel germplasm, having promising flooding and drought tolerance potential (Jensen et al., 2012; Lee et al., 2015). Elymus repens has been reported to possess a high level of resistance to Fusarium head blight and has, therefore, been recommended as an important genetic resource for breeding FHB-wheat (Zeng et al., 2013). Elymus semicostatus has recently been reported to exhibit high water use efficiency while maintaining stable relative water content and high chlorophyll despite being exposed to an unfavorable environment, suggesting it is a reservoir of diverse, tolerant genes (Kumar et al., 2022). In the current study, while comparing the overall performances of the studied plant materials (Supplementary material 9), an arbitrary scoring was done by assigning a score of 3 to the top 33% of Elymus species for any specific trait, a score of 2 to the next 33%, and a score of 1 to the remaining species. Accordingly, the maximum score was recorded for E. borianus (i.e., 39 in MS, 37 in SS, and 42 in both combined stresses), followed by E. russelli, E. caninus, E. longioristatus, and E. dauhuricus. Interestingly, in terms of stress tolerance, these Elymus species outperformed the check wheat cultivar, Pirsabak 2005, which is a rainfed variety. Conclusively, E. borianus, which is endemic to Swat, Pakistan, along with E. russelli, E. caninus, E. longioristatus, and E. dauhuricus. have proved as wild wheat relatives harbor the untapped genetic diversity for a variety of morpho-physiological and anatomical attributes under progressive water stress conditions.
Conclusions
We investigated responses of Elymus species and wheat cultivars under osmotic (germination traits) and progressive water stress (physio-biochemical and anatomical characteristics). Several structural, morpho-physiological, and biochemical responses observed during the current study may be interpreted to have assisted these species in tolerating progressive water stress conditions. In conclusion, E. borianus, which is endemic to Swat, Pakistan, along with E. russelli, E. caninus, E. longioristatus, and E. dauhuricus, have proved wild wheat relatives harboring the untapped genetic diversity for a variety of the studied traits under progressive water stress conditions. Further, exploration/characterization of these Elymus species, including ex situ conservation/domestication and molecular confirmation, is suggested for the successful use and introgression of desirable traits in future wheat breeding programs.
Data availability statement
The original contributions presented in the study are included in the article/Supplementary material, further inquiries can be directed to the corresponding author/s.
Author contributions
AK, AA, and ZU designed the research. AK conducted the research. PK, AA, IA, and MA analyzed the data. AA, AR, and HS checked the final content of the manuscript. All authors contributed to the article and approved the submitted version.
Acknowledgments
The authors would like to extend their sincere appreciation to the Researchers Supporting Project Number (RSP-2021/180), King Saud University, Riyadh, Saudi Arabia. The authors would also thankful to Bio Resource Conservation Institute, National Agriculture Center, Islamabad, Pakistan for providing seeds of checked wheat cultivars.
Conflict of interest
The authors declare that the research was conducted in the absence of any commercial or financial relationships that could be construed as a potential conflict of interest.
The reviewer JI declared a shared affiliation with the author AR to the handling editor at the time of the review.
Publisher's note
All claims expressed in this article are solely those of the authors and do not necessarily represent those of their affiliated organizations, or those of the publisher, the editors and the reviewers. Any product that may be evaluated in this article, or claim that may be made by its manufacturer, is not guaranteed or endorsed by the publisher.
Supplementary material
The Supplementary Material for this article can be found online at: https://www.frontiersin.org/articles/10.3389/fpls.2022.982844/full#supplementary-material
References
Abbas, Z., Khan, S. M., Abbasi, A. M., Pieroni, A., Ullah, Z., Iqbal, M., et al. (2016). Ethnobotany of the balti community, tormik valley, karakorum range, Baltistan, Pakistan. J. Ethnobiol. Ethnomed. 12, 1–16. doi: 10.1186/s13002-016-0114-y
Abdi, H., and Williams, L. J. (2010). Principal component analysis. Wiley interdisciplinary reviews. Comput. Stat. 2, 433–459. doi: 10.1002/wics.101
Afzal, F., Ali, A., Ullah, Z., Sher, H., Gul, A., Mujeeb-Kazi, A., et al. (2018). Terminal drought stress adaptability in synthetic-derived bread wheat is explained by alleles of major adaptability genes and superior phenology. Int. J. Agric. Biol. 20,1623–1631. doi: 10.17957/IJAB/15.0680
Ahmadi, J., Pour-Aboughadareh, A., Ourang, S. F., Mehrabi, A. A., and Siddique, K. H. (2018). Wild relatives of wheat: Aegilops–Triticum accessions disclose differential antioxidative and physiological responses to water stress. Acta Physiol. Plant 40, 90. doi: 10.1007/s11738-018-2673-0
Ahmed, H. G. M. D., Sajjad, M., Li, M., Azmat, M. A., Rizwan, M., Maqsood, R. H., et al. (2019). Selection criteria for drought-tolerant bread wheat genotypes at the seedling stage. Sustainability 11, 2584. doi: 10.3390/su11092584
Ali, A., Ali, Z., Quraishi, U. M., Kazi, A. G., Malik, R. N., Sher, H., et al. (2014a). “Integrating physiological and genetic approaches for improving drought tolerance in crops,” in Emerging Technologies and Management of Crop Stress Tolerance. Academic Press, p. 315–345. doi: 10.1016/B978-0-12-800875-1.00014-4
Ali, A., Arshad, M., Naqvi, S. M. S., Ahmad, M., Sher, H., Fatima, S., et al. (2014b). Exploitation of synthetic-derived wheats through osmotic stress responses for drought tolerance improvement. Acta Physiol. Plant 36, 2453–2465. doi: 10.1007/s11738-014-1618-5
Ali, A., Arshad, M., Naqvi, S. M. S., Rasheed, A., Sher, H., Kazi, A. G., et al. (2015). Comparative assessment of synthetic-derived and conventional bread wheat advanced lines under osmotic stress and implications for molecular analysis. Plant Mol. Biol. Rep. 33, 1907–1917. doi: 10.1007/s11105-015-0884-8
Ali, I., Salah, K. B. H., Sher, H., Ali, H., Ullah, Z., Ali, A., et al. (2022a). Drought stress enhances the efficiency of floral dip method of Agrobacterium-mediated transformation in Arabidopsis thaliana. Brazil. J. Biol. 84:e259326. doi: 10.1590/1519-6984.259326
Ali, I., Sher, H., Ali, A., Hussain, S., and Ullah, Z. (2022b). Simplified floral dip transformation method of Arabidopsis thaliana. J. Microbiol. Methods 197, 106492. doi: 10.1016/j.mimet.2022.106492
Ali, Z., Ali, B., Mohammad, A., Ahmad, M., Ahmad, I., Napar, A. A., et al. (2013). “Combating water scarcity for global food security,” in Agricultural Systems in the 21st Century, p. 1–30
Alscher, R. G., Erturk, N., and Heath, L. S. (2002). Role of superoxide dismutases (SODs) in controlling oxidative stress in plants. J. Exp. Bot. 53, 1331–1341. doi: 10.1093/jexbot/53.372.1331
Arantes, M. K., da Silva Filho, M. P., Pennacchi, J. P., das Chagas Mendonca, A. M., and Barbosa, J. P. R. A.D. (2020). Phenotypic plasticity of leaf anatomical traits helps to explain gas-exchange response to water shortage in grasses of different photosynthetic types. Theor. Exp. Plant. Physiol. 32, 341–356. doi: 10.1007/s40626-020-00190-x
Ashraf, M. (2010). Inducing drought tolerance in plants: recent advances. Biotechnol. Adv. 28, 169–183. doi: 10.1016/j.biotechadv.2009.11.005
Association of Official Seed Analysts (AOSA). (1983). Seed vigor testing handbook. Association of Official Seed Analysts. Contribution 32 to the Handbook on Seed Testing. Association of Official Seed Analysis, Springfield, IL.
Aubry, C. A., Shoal, R. Z., and Erickson, V. (2005). Grass Cultivars: Their Origins, Development, and Use on National Forests and Grasslands of the Pacific Northwest. US Forest Service, Pacific Northwest Region, p. 44.
Aung, T. (1991). Intergeneric hybrids between Hordeum vulgare and Elymus trachycaulus resistant to Russian wheat aphid. Genome 34, 954–960. doi: 10.1139/g91-148
Ayub, M., Ashraf, M. Y., Kausar, A., Saleem, S., Anwar, S., Altay, V., et al. (2021). Growth and physio-biochemical responses of maize (Zea mays L.) to drought and heat stresses. Plant Biosyst. 155, 535–542. doi: 10.1080/11263504.2020.1762785
Bates, L. S., Waldren, R. P., and Teare, I. D. (1973). Rapid determination of free proline for water-stress studies. Plant Soil 39, 205–207. doi: 10.1007/BF00018060
Bian, S., and Jiang, Y. (2009). Reactive oxygen species, antioxidant enzyme activities and gene expression patterns in leaves and roots of Kentucky bluegrass in response to drought stress and recovery. Sci. Hortic. 120, 264–270. doi: 10.1016/j.scienta.2008.10.014
Borrajo, C. I., Sanchez-Moreiras, A. M., and Reigosa, M. J. (2018). Morpho-physiological responses of tall wheatgrass populations to different levels of water stress. PloS ONE 13, 0209281. doi: 10.1371/journal.pone.0209281
Bosabalidis, A. M., and Kofidis, G. (2002). Comparative effects of drought stress on leaf anatomy of two olive cultivars. Plant Sci. J. 163, 375–379. doi: 10.1016/S0168-9452(02)00135-8
Bouchemal, K., Bouldjadj, R., Belbekri, M. N., Ykhlef, N., and Djekoun, A. (2017). Differences in antioxidant enzyme activities and oxidative markers in ten wheat (Triticum durum Desf.) genotypes in response to drought, heat and paraquat stress. Arch. Agron. Soil Sci. 63, 710–722. doi: 10.1080/03650340.2016.1235267
Boughalleb, F., Abdellaoui, R., Ben-Brahim, N., and Neffati, M. (2014). Anatomical adaptations of Astragalus gombiformis Pomel. under drought stress. Open Life Sci. 9, 1215–1225. doi: 10.2478/s11535-014-0353-7
Chartzoulakis, K., Patakas, A., Kofidis, G., Bosabalidis, A., and Nastou, A. (2002). Water stress affects leaf anatomy, gas exchange, water relations and growth of two avocado cultivars. Sci. Hortic. 95, 39–50. doi: 10.1016/S0304-4238(02)00016-X
Chaudhary, H. K., Kaila, V., Rather, S. A., Badiyal, A., Hussain, W., Jamwal, N. S., et al. (2014). “Wheat,” in Alien Gene Transfer in Crop Plants, Volume 2. Springer: New York, NY. p. 1–26. doi: 10.1007/978-1-4614-9572-7_1
Chaves, M. M., Flexas, J., and Pinheiro, C. (2009). Photosynthesis under drought and salt stress: regulation mechanisms from whole plant to cell. Ann. Bot. 103, 551–560. doi: 10.1093/aob/mcn125
Clark, J. (1960). Preparation of leaf epidermis for topographic study. Stain Technol. 35, 35–39. doi: 10.3109/10520296009114713
Cope, T. A. (1982). “Flora of Pakistan, No. 143: Poaceae,” in eds E. Nasir and S. I. Ali (Pakistan Agricultural Research Council Islamabad and University of Karachi, Pakistan), p. 678.
Cyriac, D., Hofmann, R. W., Stewart, A., Sathish, P., Winefield, C. S., and Moot, D. J. (2018). Intraspecific differences in long-term drought tolerance in perennial ryegrass. PloS ONE 13, 0194977. doi: 10.1371/journal.pone.0194977
David, O. A., Osonubi, O., Olaiya, C. O., Agbolade, J. O., Ajiboye, A. A., Komolafe, R. J., et al. (2017). Anatomical response of wheat cultivars to drought stress. Ife J. Sci. 19, 323–331. doi: 10.4314/ijs.v19i2.12
Dewey, D. R. (1984). “The genomic system of classification as a guide to intergeneric hybridization with the perennial Triticeae,” in Gene Manipulation in Plant Improvement (Springer, Boston, MA), p. 209–279. doi: 10.1007/978-1-4613-2429-4_9
Dolezal, J., Klimes, A., Dvorsky, M., Riha, P., Klimesova, J., and Schweingruber, F. (2019). Disentangling evolutionary, environmental and morphological drivers of plant anatomical adaptations to drought and cold in Himalayan graminoids. Oikos 128, 1576–1587. doi: 10.1111/oik.06451
Dubois, M., Gilles, K. A., Hamilton, J. K., Rebers, P. T., and Smith, F. (1956). Colorimetric method for determination of sugars and related substances. Anal. Chem. 28, 350–356. doi: 10.1021/ac60111a017
Ehdaie, B., Alloush, G. A., Madore, M. A., and Waines, J. G. (2006). Genotypic variation for stem reserves and mobilization in wheat. Crop. Sci. 46, 2093–2103. doi: 10.2135/cropsci2006.01.0013
El-Afry, M. M. (2012). Anatomical studies on drought-stressed wheat plants (Triticum aestivum L.) treated with some bacterial strains. Acta Biol. Szeged 56, 165–174.
Ellis, R. H., and Roberts, E. H. (1981). The quantification of ageing and survival in orthodox seeds. Seed Sci. Technol. 9, 373–409.
Engloner, A. I., Kovacs, D., Balogh, J., and Tuba, Z. (2003). Anatomical and eco-physiological changes in leaves of couch-grass (Elymus repens L.), a temperate loess grassland species, after 7 years growth under elevated CO2 concentration. Photosynthetica 41, 185–189. doi: 10.1023/B:PHOT.0000011950.80843.79
Erenstein, O., Jaleta, M., Mottaleb, K. A., Sonder, K., Donovan, J., and Braun, H. J. (2022). “Global trends in wheat production, consumption and trade,” in Wheat Improvement (Springer, Cham), p. 47–66. doi: 10.1007/978-3-030-90673-3_4
Fatima, S., Arshad, M. N., Chaudhari, S. K., Ali, A., Amjad, M. S., and Kausar, R. (2014). Utilization of synthetics for drought tolerance in bread wheat (Triticum aestivum L.). Int. J. Biosci. 5, 104–112. doi: 10.12692/ijb/5.1.104-112
Gazanchian, A., Hajheidari, M., Sima, N. K., and Salekdeh, G. H. (2007). Proteome response of Elymus elongatum to severe water stress and recovery. J. Exp. Bot. 58, 291–300. doi: 10.1093/jxb/erl226
Giannopolitis, C. N., and Ries, S. K. (1977). Superoxide dismutases: I. Occurrence in higher plants. Plant Physiol. 59, 309–314. doi: 10.1104/pp.59.2.309
Gill, S. S., and Tuteja, N. (2010). Reactive oxygen species and antioxidant machinery in abiotic stress tolerance in crop plants. Plant Physiol. Biochem. 48, 909–930. doi: 10.1016/j.plaphy.2010.08.016
Gong, B., Zhu, W., Li, S., Wang, Y., Xu, L., Wang, Y., et al. (2019). Molecular cytogenetic characterization of wheat–Elymus repens chromosomal translocation lines with resistance to Fusarium head blight and stripe rust. BMC Plant Biol. 19, 1–11. doi: 10.1186/s12870-019-2208-x
Gorham, J., McDonnell, E., and Jones, R. W. (1984). Salt tolerance in the Triticeae: Leymus sabulosus. J. Exp. Bot. 35, 1200–1209. doi: 10.1093/jxb/35.8.1200
Gorin, N., and Heidema, F. T. (1976). Peroxidase activity in golden delicious apples as a possible parameter of ripening and senescence. J. Agric. Food Chem. 24, 200–201. doi: 10.1021/jf60203a043
Graber, E. R., Harel, Y. M., Kolton, M., Cytryn, E., Silber, A., David, D. R., et al. (2010). Biochar impact on development and productivity of pepper and tomato grown in fertigated soilless media. Plant Soil 337, 481–496. doi: 10.1007/s11104-010-0544-6
Grote, U., Fasse, A., Nguyen, T. T., and Erenstein, O. (2021). Food security and the dynamics of wheat and maize value chains in Africa and Asia. Frontiers in Sustainable Food Systems, 4, 617009.Gul, A., Rasheed, A., Afzal, F., Napar, A. A., Ali, A., Jamil, M., Khalid, M., Bux, H., Mujeeb-Kazi, A., 2015. Characterization of synthetic hexaploids derived from same Aegilops tauschii accessions and different durum cultivars. Cytologia 80, 427–440. doi: 10.1508/cytologia.80.427
Habora, M. E. E., Eltayeb, A. E., Tsujimoto, H., and Tanaka, K. (2012). Identification of osmotic stress-responsive genes from Leymus mollis, a wild relative of wheat (Triticum aestivum L.). Breed. Sci. 62, 78–86. doi: 10.1270/jsbbs.62.78
Haworth, M., Centritto, M., Giovannelli, A., Marino, G., Proietti, N., Capitani, D., et al. (2017). Xylem morphology determines the drought response of two Arundo donax ecotypes from contrasting habitats. Glob. Change Biol. Bioenergy 9, 119–131. doi: 10.1111/gcbb.12322
Hessini, K., Martínez, J. P., Gandour, M., Albouchi, A., Soltani, A., and Abdelly, C. (2009). Effect of water stress on growth, osmotic adjustment, cell wall elasticity and water-use efficiency in Spartina alterniflora. Environ. Exp. Bot. 67, 312–319. doi: 10.1016/j.envexpbot.2009.06.010
Ilyas, M., Mahmood, T., Ali, A., Babar, M., Rasheed, A., and Mujeeb-Kazi, A. (2015). Characterization of D-genome diversity for tolerance to boron toxicity in synthetic hexaploid wheat and in silico analysis of candidate genes. Acta Physiol. Plantarum 37, 1–13. doi: 10.1007/s11738-014-1765-8
Jensen, K. B. (1990). Cytology and taxonomy of Elymus kengii, E. grandiglumis, E. alatavicus, and E. batalinii (Poaceae: Triticeae). Genome. 33, 668–673. doi: 10.1139/g90-099
Jensen, K. B., Mott, I. W., Robins, J. G., Waldron, B. L., and Nelson, M. (2012). Genetic improvement and diversity in Snake River wheatgrass (Elymus wawawaiensis) (Poaceae: Triticeae). Rangel Ecol. Manag. 65, 76–84. doi: 10.2111/REM-D-10-00159.1
Kazi, A. G., Rasheed, A., Bux, H., Napar, A. A., Ali, A., and Mujeeb-Kazi, A. (2015). Cytological, phenological and molecular characterization of B (S)-genome synthetic hexaploids (2n= 6x = 42; AABBSS). Cereal Res. Commun. 43, 179–188. doi: 10.1556/CRC.2014.0043
Khan, S. M., Page, S., Ahmad, H., and Harper, D. (2012). Anthropogenic influences on the natural ecosystem of the Naran Valley in the Western Himalayas. Pakistan J. Botany 44, 231.
Kiani, R., Arzani, A., and Habibi, F. (2015). Physiology of salinity tolerance in Aegilops cylindrica. Acta Physiol. Plant 37, 1–10. doi: 10.1007/s11738-015-1881-0
Kumar, A., Sharma, A., Sharma, R., Choudhary, A., Srivastava, P., Kaur, H., et al. (2022). Morpho-physiological evaluation of Elymus semicostatus (Nees ex Steud.) Melderis as potential donor for drought tolerance in Wheat (Triticum aestivum L.). Genet. Resour. Crop. Evol. 69, 411–430. doi: 10.1007/s10722-021-01241-1
Larsen, S. U., and Andreasen, C. (2004). Light and heavy turfgrass seeds differ in germination percentage and mean germination thermal time. Crop. Sci. 4, 1710–1720. doi: 10.2135/cropsci2004.1710
Lee, S. H., Lee, K. W., Lee, D. G., Son, D., Park, S. J., Kim, K. Y., et al. (2015). Identification and functional characterization of Siberian wild rye (Elymus sibiricus L.) small heat shock protein 16.9 gene (EsHsp16. 9) conferring diverse stress tolerance in prokaryotic cells. Biotechnol. Lett. 37, 881–890. doi: 10.1007/s10529-014-1749-1
Liu, H. S., and Li, F. M. (2005). Root respiration, photosynthesis and grain yield of two spring wheat in response to soil drying. Plant Growth Regulat. 46, 233–240. doi: 10.1007/s10725-005-8806-7
Liu, J., Kutschke, S., Keimer, K., Kosmalla, V., Schürenkamp, D., Goseberg, N., et al. (2021). Experimental characterisation and three-dimensional modelling of Elymus for the assessment of ecosystem services. Ecol. Eng. 166, 106233. doi: 10.1016/j.ecoleng.2021.106233
Lovisolo, C., and Schubert, A. (1998). Effects of water stress on vessel size and xylem hydraulic conductivity in Vitis vinifera L. J. Exp. Bot. 49, 693–700. doi: 10.1093/jexbot/49.321.693
Lowry, O. H., Rosebrough, N. J., Farr, A. L., and Randall, R. J. (1951). Protein measurement with the Folin phenol reagent. J. Biol. Chem. 193, 265–275. doi: 10.1016/S0021-9258(19)52451-6
Lv, J., Zong, X. F., Ahmad, A. S., Wu, X., Wu, C., Li, Y. P., et al. (2020). Alteration in morpho-physiological attributes of Leymus chinensis (Trin.) Tzvelev by exogenous application of brassinolide under varying levels of drought stress. Chil. J. Agric. Res. 80, 61–71. doi: 10.4067/S0718-58392020000100061
Mahrookashani, A., Siebert, S., Hüging, H., and Ewert, F. (2017). Independent and combined effects of high temperature and drought stress around anthesis on wheat. J. Agron Crop. Sci. 203, 453–463. doi: 10.1111/jac.12218
Mammadov, J., Buyyarapu, R., Guttikonda, S. K., Parliament, K., Abdurakhmonov, I. Y., and Kumpatla, S. P. (2018). Wild relatives of maize, rice, cotton, and soybean: treasure troves for tolerance to biotic and abiotic stresses. Front Plant Sci. 9, 886. doi: 10.3389/fpls.2018.00886
Martinez, L. M., Fernandez-Ocana, A., Rey, P. J., Salido, T., Amil-Ruiz, F., and Manzaneda, A. J. (2018). Variation in functional responses to water stress and differentiation between natural allopolyploid populations in the Brachypodium distachyon species complex. Ann Bot. 121, 1369–1382. doi: 10.1093/aob/mcy037
Masoomi-Aladizgeh, F., Aalami, A., Esfahani, M., Aghaei, M. J., and Mozaffari, K. (2015). Identification of CBF14 and NAC2 genes in Aegilops tauschii associated with resistance to freezing stress. Appl. Biochem. Biotechnol. 176, 1059–1070. doi: 10.1007/s12010-015-1629-8
Nassar, R., Kamel, H. A., Ghoniem, A. E., Alarcon, J. J., Sekara, A., Ulrichs, C., et al. (2020). Physiological and anatomical mechanisms in wheat to cope with salt stress induced by seawater. Plants 9, 237. doi: 10.3390/plants9020237
Noein, B., and Soleymani, A. (2022). Corn (Zea mays L.) physiology and yield affected by plant growth regulators under drought stress. J. Plant Growth Regulat. 41, 672–681. doi: 10.1007/s00344-021-10332-3
Nouraein, M., Mohammadi, S. A., Aharizad, S., Moghaddam, M., and Sadeghzadeh, B. (2013). Evaluation of drought tolerance indices in wheat recombinant inbred line population. Ann. Biol. Res. 4, 113–122.
Petrov, P., Petrova, A., Dimitrov, I., Tashev, T., Olsovska, K., Brestic, M., et al. (2018). Relationships between leaf morpho-anatomy, water status and cell membrane stability in leaves of wheat seedlings subjected to severe soil drought. J. Agron. Crop. Sci. 204, 219–227. doi: 10.1111/jac.12255
Pour-Aboughadareh, A., Ahmadi, J., Mehrabi, A. A., Etminan, A., Moghaddam, M., and Siddique, K. H. (2017). Physiological responses to drought stress in wild relatives of wheat: implications for wheat improvement. Acta Physiol. Plant 39, 106. doi: 10.1007/s11738-017-2403-z
Prasad, P. V. V., Pisipati, S. R., Momcilovic, I., and Ristic, Z. (2011). Independent and combined effects of high temperature and drought stress during grain filling on plant yield and chloroplast EF-Tu expression in spring wheat. J. Agron. Crop Sci. 197, 430–441. doi: 10.1111/j.1439-037X.2011.00477.x
Rafique, K., Rauf, C. A., Gul, A., Bux, H., Memon, R. A., Ali, A., et al. (2017). Evaluation of d-genome synthetic hexaploid wheats and advanced derivatives for powdery mildew resistance. Pakistan J. Botany 49, 735–743.
Rasheed, R., Mujeeb-Kazi, A., Ogbonnaya, F. C., He, Z., and Rajaram, S. (2018). Wheat genetic resources in the post-genomics era: promise and challenges. Ann. Bot. 121, 603–616. doi: 10.1093/aob/mcx148
Ray, D. K., Gerber, J. S., MacDonald, G. K., and West, P. C. (2015). Climate variation explains a third of global crop yield variability. Nat. Commun. 6, 5989. doi: 10.1038/ncomms6989
Ruzin, S. E. (1999). Plant Microtechnique and Microscopy (Vol. 198). New York: Oxford University Press.
Salam, A., Ali, A., Afridi, M. S., Ali, S., and Ullah, Z. (2022). “Agrobiodiversity: Effect of drought stress on the eco-physiology and morphology of wheat,” in Biodiversity, Conservation and Sustainability in Asia (Springer, Cham), p. 597–618. doi: 10.1007/978-3-030-73943-0_33
Sasanuma, T., Endo, T. R., and Ban, T. (2002). Genetic diversity of three Elymus species indigenous to Japan and East Asia (E. tsukushiensis, E. humidus and E. dahuricus) detected by AFLP. Genes. Genet. Syst. 77, 429–438. doi: 10.1266/ggs.77.429
Schooler, A. B., and Anderson, M. K. (1980). Behaviour of integeneric hybrids between Hordeum vulgare L. (4x) and Elymus mollis type. Cytologia 45, 157–162. doi: 10.1508/cytologia.45.157
Selim, A. F. H., and El-Nady, M. F. (2011). Physio-anatomical responses of drought stressed tomato plants to magnetic field. Acta Astronaut. 69, 387–396. doi: 10.1016/j.actaastro.2011.05.025
Selim, D. A. F. H., Nassar, R. M. A., Boghdady, M. S., and Bonfill, M. (2019). Physiological and anatomical studies of two wheat cultivars irrigated with magnetic water under drought stress conditions. Plant Physiol. Biochem. 135, 480–488. doi: 10.1016/j.plaphy.2018.11.012
Steel, R. G. D., Torrie, J. H., and Dicky, D. A. (1997). Principles and Procedures of Statistics. A Biometrical Approach, 3rd edn. McGraw Hill Book International Co.: Singapore, p. 204–227.
Suneja, Y., Gupta, A. K., and Bains, N. S. (2017). Bread wheat progenitors: Aegilops tauschii (DD genome) and Triticum dicoccoides (AABB genome) reveal differential antioxidative response under water stress. Physiol. Mol. Biol. Plants 23, 99–114. doi: 10.1007/s12298-016-0409-4
Tan, L., Huang, Q. X., Song, Y., Wu, D. D., Cheng, Y. R., Zhang, C. B., et al. (2022). Biosystematics studies on Elymus breviaristatus and Elymus sinosubmuticus (Poaceae: Triticeae). BMC Plant Biol. 22, 1–9. doi: 10.1186/s12870-022-03441-y
Thangthong, N., Jogloy, S., Punjansing, T., Kvien, C. K., Kesmala, T., and Vorasoot, N. (2019). Changes in root anatomy of peanut (Arachis hypogaea L.) under different durations of early season drought. Agronomy 9, 215. doi: 10.3390/agronomy9050215
The jamovi project (2021). Jamovi. (Version 1.8) [Computer Software]. Available online at: https://www.jamovi.org (accessed February 24, 2022).
Ullah, Z., Ahmad, M., Sher, H., Shaheen, H., and Khan, S. M. (2015). Phytogeographic analysis and diversity of grasses and sedges (Poales) of Northern Pakistan. Pakistan J. Botany 47, 93–104.
Verma, K. K., Singh, M., Gupta, R. K., and Verma, C. L. (2014). Photosynthetic gas exchange, chlorophyll fluorescence, antioxidant enzymes, and growth responses of Jatropha curcas during soil flooding. Turk J. Bot. 38, 130–140. doi: 10.3906/bot-1212-32
Wang, R., Huang, W., Chen, L., Ma, L., Guo, C., and Liu, X. (2011). Anatomical and physiological plasticity in Leymus chinensis (Poaceae) along large-scale longitudinal gradient in northeast China. PLoS ONE 6, 26209. doi: 10.1371/journal.pone.0026209
Wei, L., Zhang, C., Dong, Q., Yang, Z., Chu, H., Yu, Y., et al. (2021). Effects of temperature and water potential on seed germination of 13 Poa L. species in the Qinghai-Tibetan Plateau. Glob. Ecol. Conserv. 25, 01442. doi: 10.1016/j.gecco.2020.e01442
Wickham, H., Chang, W., Henry, L., Pedersen, T. L., Takahashi, K., Wilke, C., Woo, K., and RStudio (2018). ggplot2: Create Elegant Data Visualisations Using the Grammar of Graphics. [R package]. Available online at: https://CRAN.R-project.org/package=ggplot2 (accessed February 24, 2022).
Wu, L. M., Fang, Y., Yang, H. N., and Bai, L. Y. (2019). Effects of drought-stress on seed germination and growth physiology of quinclorac-resistant Echinochloa crusgalli. PloS ONE 14, 0214480. doi: 10.1371/journal.pone.0214480
Xiong, Y., Lei, X., Bai, S., Xiong, Y., Liu, W., Wu, W., et al. (2021). Genomic survey sequencing, development and characterization of single-and multi-locus genomic SSR markers of Elymus sibiricus L. BMC Plant Biol. 21, 1–12. doi: 10.1186/s12870-020-02770-0
Xu, Z., and Zhou, G. (2008). Responses of leaf stomatal density to water status and its relationship with photosynthesis in a grass. J. Exp. Bot. 59, 33173325. doi: 10.1093/jxb/ern185
Xu, Z. Z., and Zhou, G. S. (2007). Photosynthetic recovery of a perennial grass Leymus chinensis after different periods of soil drought. Plant Prod. Sci. 10, 277–285. doi: 10.1626/pps.10.277
Yang, C. R., Zhang, H. Q., Chen, W. H., Kang, H. Y., Wang, Y., Sha, L. N., et al. (2017). Genomic constitution and intergenomic translocations in the Elymus dahuricus complex revealed by multicolor GISH. Genome 60, 510–517. doi: 10.1139/gen-2016-0199
Zeng, J., Cao, W., Hucl, P., Yang, Y., Xue, A., Chi, D., et al. (2013). Molecular cytogenetic analysis of wheat–Elymus repens introgression lines with resistance to Fusarium head blight. Genome 56, 75–82. doi: 10.1139/gen-2012-0130
Zhang, H., Mittal, N., Leamy, L. J., Barazani, O., and Song, B. H. (2017). Back into the wild-Apply untapped genetic diversity of wild relatives for crop improvement. Evol. Appl. 10, 5–24. doi: 10.1111/eva.12434
Zhang, Y. P., and Nan, Z. B. (2007). Growth and antioxidative systems changes in Elymus dahuricus is affected by Neotyphodium endophyte under contrasting water availability. J. Agron. Crop. Sci. 193, 377–386. doi: 10.1111/j.1439-037X.2007.00279.x
Zhang, Y. P., and Nan, Z. B. (2010). Germination and seedling antioxidative enzymes of endophyte-infected populations of Elymus dahuricus under osmotic stress. Seed Sci. Technol. 38, 522–527. doi: 10.15258/sst.2010.38.2.25
Zhang, Z., Zheng, Y., Zhang, J., Wang, N., Wang, Y., Liu, W., et al. (2022). High-altitude genetic selection and genome-wide association analysis of yield-related traits in Elymus sibiricus L. Using SLAF Sequencing. Front. Plant Sci. 13, 874409. doi: 10.3389/fpls.2022.874409
Keywords: crop wild resources, Elymus species, bread wheat, drought stress, leaf and culm anatomy
Citation: Khan A, Ali A, Ullah Z, Ali I, Kaushik P, Alyemeni MN, Rasheed A and Sher H (2022) Exploiting the drought tolerance of wild Elymus species for bread wheat improvement. Front. Plant Sci. 13:982844. doi: 10.3389/fpls.2022.982844
Received: 30 June 2022; Accepted: 29 August 2022;
Published: 06 October 2022.
Edited by:
Shah Fahad, The University of Haripur, PakistanReviewed by:
Fazal Ullah, Chengdu Institute of Biology (CAS), ChinaChengxuan Chen, Institute of Genetics and Developmental Biology (CAS), China
Javed Iqbal, Quaid-i-Azam University, Pakistan
Copyright © 2022 Khan, Ali, Ullah, Ali, Kaushik, Alyemeni, Rasheed and Sher. This is an open-access article distributed under the terms of the Creative Commons Attribution License (CC BY). The use, distribution or reproduction in other forums is permitted, provided the original author(s) and the copyright owner(s) are credited and that the original publication in this journal is cited, in accordance with accepted academic practice. No use, distribution or reproduction is permitted which does not comply with these terms.
*Correspondence: Ahmad Ali, aali_swat@yahoo.com; Mohammed Nasser Alyemeni, mnalyemeni@gmail.com
†ORCID: Ahmad Ali orcid.org/0000-0001-7204-1870